Original Article
Single-fraction image-guided extracranial radiosurgery for recurrent and metastatic abdominal and pelvic cancers: shortterm local control, metabolic response, and toxicity
Charles L Perkins1, Bassel El-Reyes2, Edmund Simon1, David Kooby3, William Torres 4, John S Kauh4, Charles A Staley3, Jerome C Landry1
1Departments of Radiation Oncology, Emory University, Georgia, USA; 2Department of Medical Oncology, Emory University, Georgia, USA; 3Department
of Surgical Oncology, Emory University, Georgia, USA; 4Department of Radiology, Emory University, Georgia, USA
Correspondence author: Jerome C Landry, MD. Professor, Department of Radiation Oncology, Emory University. 1365 Clifton Rd. Atlanta 30138, GA, USA. Tel: 404-778-4469, Fax: 404-616-6380. Email: jland01@emory.edu.
|
Abstract
Purpose:Extracranial radiosurgery (ECRS) is a novel treatment for inoperable recurrent or metastatic abdominopelvic cancers.
However, local control, metabolic response, and acute toxicity remain undefined. We therefore analyzed these endpoints
in patients treated with single-fraction image-guided ECRS at Emory University.
Methods: 20 patients with recurrent or metastatic inoperable abdominal or pelvic cancers (23 sites) were treated with singlefraction
ECRS using a Varian linear accelerator between 08/2006 and 02/2008. Patients with pancreas, biliary and liver cancer
were part of an IRB-approved ongoing dose-escalation trial. 14 patients had received prior abdominal or pelvic external beam
radiation. In 13 patients pre-treatment PET/CT was used to delineate the target volume. Image-guidance was provided by
implanted fiducial markers and on-board imaging in 13 patients, and with cone-beam CT in 1 patient. 8 Patients were treated
with respiratory gating. The median single-fraction dose delivered was 18 Gy. Each patient was assessed at 1 week, 1 month,
and 3 months after radiosurgery for toxicity, and at approximately 1 month and 3 months with PET/CT for metabolic tumor
response. Partial response was defined as a reduction in size of > 10% on CT and a decrease in maximum SUV of > 15% on
PET. Complete response was defined as complete resolution on CT, and a reduction of SUV to background levels on PET.
Results: The median follow-up was 6.3 months (range 1.5-12.2 months). The overall response rate (the sum of complete
responses and partial responses) by treated site was noted in 36% (1 month), 47% (3 months) and 48% (final). A complete
response was achieved in 13% (3 sites). At last follow-up, local control (sum of response rate and stable disease) was 74% . The
metabolic response rate by pet only(sum of partial and complete responders) was 85% on final analysis. 23% of pet avid sites
achieved a complete response. Two pet avid treated sites (13%) did show evidence of progression at 3 months, but subsequent
CT/FDG-PET scans showed a decrease in maximum SUV; no patients suffered progressive disease based on metabolic imaging
at last follow-up. Grade 1-2 upper GI acute toxicity (nausea, vomiting, gastritis, and pain) was noted in 47% and 55% of
patients at 1 week and 1 month, respectively. Correspondingly, acute lower GI toxicity (diarrhea, pain) was lower at 12% and
6%. Overall grade 1-2 GI toxicity was seen in 59% of patients at 1 week (pain and nausea being the most common) and 61% of
patients at 1 month post stereotactic body radiotherapy (SBRT) (nausea being the most common).
Conclusion: Single-fraction image-guided ECRS for recurrent or metastatic abdominopelvic cancers is safe and effective in
the short term. 3-month local control was very good , and was predicted by an early metabolic response as seen on PET/CT.
Acute side effects were mild, with no patient experiencing grade 3 or greater toxicity. Dose escalation and long-term studies are warranted
for this treatment approach.
Key words stereotactic body radiotherapy, pancreatic liver abdominal cancers. Single fraction sbrt, metabolic response toxicity
J Gastrointest Oncol 2010; 1: 16-23. DOI: 10.3978/j.issn.2078-6891.2010.010
|
Introduction
A recent theory proposes that not all metastatic disease is
diffuse or systemic, and may be localized in number and
anatomic location. In such cases of “oligometastases,” durable
response or potentially cure may be obtained with local
therapy (1, 2). In fact, surgical series involving a number of
sites including oligometastatic lung, liver, and adrenal have demonstrated the role of local treatment in such cases (3-6).
Historically, however, such patients generally were not treated
in a curative fashion, and most patients in this setting may not
be surgical candidates for medical or anatomic reasons.
In addition, patients with local or regional recurrence of
malignancy after primary treatment are generally deemed
unsalvageable. Specifically, patients with abdomino-pelvic
malignancies often have received a combination of surgery,
local radiotherapy, and chemotherapy, which often precludes
further local treatment for locoregional recurrence. However,
as in the case with oligometastases, further local therapy for
abdomino-pelvic recurrences may offer benefit in terms of
local control and disease-free survival.
Technological advances have enabled the precise delivery
of highly focused radiation doses to small areas, with minimal
surrounding tissue exposure. Such techniques, termed
stereotactic body radiotherapy (SBRT) or extracranial
radiosurgery (ECRS), have demonstrated promising results
in lung cancer (7-11), and for spinal metastases (12-15). In
addition, phase I/II trials for primary liver malignancies and
liver metastases have demonstrated a local control benefit,
with acceptable toxicity (16-19). However, the majority of
these regimens include fractionation involving 3 or greater
treatments, while the effectiveness and toxicity of single and
highly hypofractionated SBRT in the abdomen and pelvis
remains largely unexplored, as well as the effectiveness of
SBRT in the treatment of recurrent disease in this area.
We therefore undertook a retrospective analysis of patients
with oligometastatic or recurrent or abdomino-pelvic tumors
treated with hypofractionated (1-3 fractions) stereotactic
body radiotherapy at Emory University between May 2006
and April 2008. Primary outcomes measured were local
control and response rate, with secondary outcomes including
acute toxicity and metabolic response.
|
Materials and methods
Patients
After obtaining IRB approval, the records of the Radiation
Oncology department of the Emory Clinic were reviewed
for patients who received hypofractionated stereotactic body
radiotherapy for oligometastatic or recurrent pathologicallyproven
abdomino-pelvic malignancies. Twenty patients
were identified, representing 23 individual anatomic targets
treated between May 2006 and April 2008. Details identified
included radiation treatment specifications, pre- and post-
SBRT CT/ [18F] fluorodeoxyglucose-positron emission
tomography (FDG)-PET scans, serum liver function tests,
and follow-up clinic exams. A Whole-Body Vaclock (Med-
Tec), a device that immobilizes the patient by creating a rigid, conformal mold around the patient’s body as well as utilizing
straps around the patient, was used for each patient at the
time of simulation. Next, a pancreatic protocol 3D CT scan
was performed with the patient in the treatment position. If
respiratory motion was anticipated, a 4D CT “gated” scan was
performed using the Real-time Position Management system
(Varian) and images were transferred to the 4D workstation
(GE Medical) for motion analysis. The images from the
CT scan (3D and/or gated) were then transferred from
the workstation to the Eclipse Treatment Planning System
(Varian) for stereotactic radiation planning.
Response analysis
The response rate and toxicity data were analyzed using
Kaplan-Meier statistics. Response to treatment was
determined by comparing pre-SBRT and post-SBRT CT and
FDG-PET scans at various intervals after SBRT. Each scan
was individually reviewed, and tumor size measurements were
determined by an individual observer and compared to the
official radiology report. Tumor size on CT was determined
by the product of the maximal orthogonal diameters.
Maximum SUV values were based on the official report.
Definitions of response were based on a combination of
RECIST criteria and the revised lymphoma response criteria
(20-22). Complete Response (CR) = complete resolution of
FDG activity (to background levels) on PET with no increase
in size on CT. Partial Response (PR) = >30% decrease in
diameter product of lesion on CT, with no increase in mean
SUV on FDG-PET; or >10% decrease in mean SUV on
PET with no increase in diameter product of lesion on CT.
Progressive Disease (PD) = >25% increase in diameter product
of lesion on CT, or >10% increase in mean SUV on FDGPET.
Stable Disease (SD) = does not meet criteria for CR, PR,
or PD. Local Control (LC) = (CR + PR + SD).
Follow-up clinical visits at 1 week and 1 month were used
to asses for acute symptomatic toxicity. Acute GI toxicity
was scored based on the Common Terminology Criteria for
Adverse Events version 3.0. For patients with liver metastases,
or those patients with target volumes encompassing any
portion of the liver, serum liver function tests (AST, ALT,
and alkaline phosphatase) were drawn pre- and post-SBRT
at 1 week and 1 month per a related institutional phase I dose
escalation protocol. Liver toxicity was graded according to the
RTOG Cooperative Group Common Toxicity Criteria.
|
Results
Treatment characteristics
All patients were treated at the Emor y Clinic with hy pof ractionated stereotactic image-guided body
radiotherapy using a Varian Trilogy linear accelerator.
Treatment details listed by disease site are described in Table
1. Prior radiation therapy was delivered to the treated area
in 40% of patients, and 30% of patients received post-SBRT
chemotherapy for at least one cycle. The target volume for
radiotherapy was delineated by the fusion of the simulation
CT scan with pre-treatment diagnostic CT or CT/FDG-PET
imaging, to encompass the gross tumor volume (GTV) on
CT or volume with SUV > 3.5 units (body weight) on FDGPET. A planning target volume (PTV) was constructed by
adding a custom 2-5mm margin radially around the GTV.
Respiratory gating with a 4-D CT simulation was performed
with 9 treated sites (39%). Radiation was delivered in a single
fraction (87% sites), or fractionated over 2 to 3 treatments,
each at least 3 days apart. Isodose lines of typical treatment
plan for a metastatic colon adenocarcinoma lymph node
treated with one fraction is depicted in Figure 1.
For image guidance, the interventional radiology service
implanted radio-opaque fiducial markers in close proximity to the tumor target in 18 (78%) sites. At the time of treatment,
these markers were utilized as on-board imaging targets for
kv-kv image matching, incorporating respiratory gating as
appropriate. Of the remaining 5 treated sites, image guidance
was performed by cone beam CT at the time of treatment
in 3 cases. Treatment setup was confirmed in the final 2 sites
by bony kv-kv image matching. A summary of treatment
characteristics is listed in Table 2.
Treatment response and local control
Treatment response based on CT & FDG-PET imaging at
1 month, 3 months, and last follow-up is presented in Table 3, with a median follow-up of 6.3 months after SBRT (range
1.5-12.2 months). The overall response rate (the sum of
complete responses and partial responses) by treated site was
noted in 36% (1 month), 47% (3 months) and 48% (final).
A complete response was achieved in 13% (3 sites). At last
follow-up, local control (sum of response rate and stable
disease) was 74% (Tab 3, Fig 2). Table 4 lists local control by
specifically grouped treatment sites.
Metabolic response
Pre-and post-SBRT evaluable CT/FDG-PET scans were
available for review in 39% of treated sites. Based on maximum reported SUV, the metabolic response rate (sum
of partial and complete responders) was 85% on final analysis
(Tab 5, Fig 3). 23% of sites achieved a complete response.
Two treated sites (13%) did show evidence of progression
at 3 months, but subsequent CT/FDG-PET scans showed a
decrease in maximum SUV; no patients suffered progressive
disease based on metabolic imaging at last follow-up.
Acute GI toxicity
Acute symptomatic toxicity was evaluated based on scheduled 1 week and 1 month clinical exam follow-up visits
(Tab 6). Grade 1-2 upper GI acute toxicity (nausea, vomiting,
gastritis, and pain) was noted in 47% and 55% of patients at
1 week and 1 month, respectively. Correspondingly, acute
lower GI toxicity (diarrhea, pain) was lower at 12% and 6%.
Overall grade 1-2 GI toxicity was seen in 59% of patients
at 1 week (pain and nausea being the most common) and
61% of patients at 1 month post SBRT (nausea being the
most common). Although not reported in the manuscript,
acute upper and lower GI toxicity resolved by 3 months post
radiosurgery.
Liver toxicity
In 7 patients (7 sites), the treated volume encompassed a
portion of the liver. Based on pre- and post-SBRT serum LFT’
s (AST, ALT, alk phos), only 1 patient (14%) suffered Grade
2 toxicity at 1-week, and 2 patients (29%) experienced grade
2 toxicity at 1-month (Tab 7). No patients suffered grade 1 or
grade 3+ liver toxicity at last follow-up.
Univariate Analysis of Patient and Treatment Factors Related to Change in Split Renal Function on Renal Scintigraphy
Twenty two patients were identified who had <5% increase, no change, or decrease split function on renogram obtained 6-12 months following radiation and had complete dose volume parameters available for review. Of these, 18 of the patients (82%) had change in the relative renal function of the primarily irradiated kidney of <5% and 4 patients (18%) had decreases of ≥5%. No patient related factors were found to be associated with decrease in split renal function of the primarily irradiated kidney. Percent volumes of the primarily irradiated kidney receiving ≥25 Gy (V25) and 40 Gy (V40) were associated with decrease of ≥5% relative renal function (p=0.0387 and p=0.0438 respectively). Difference in mean kidney dose of the primarily irradiated kidney between patients with <5% change in split renal function and those with ≥5% decrease trended towards significance (p=0.0793)(Table 4).
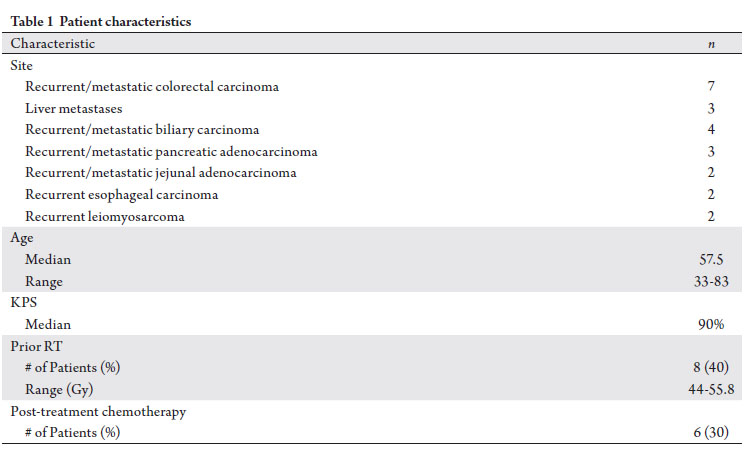
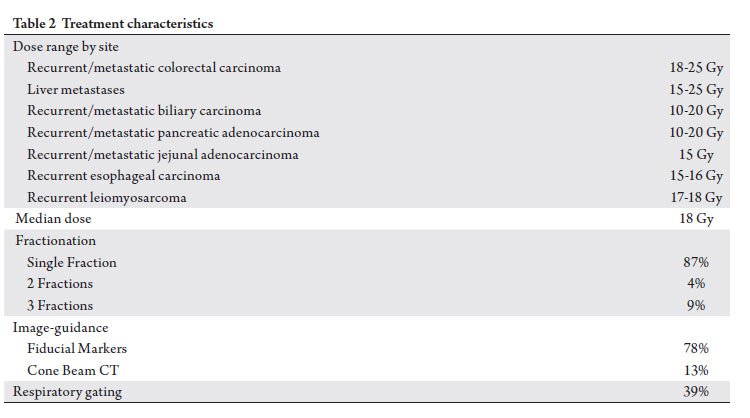
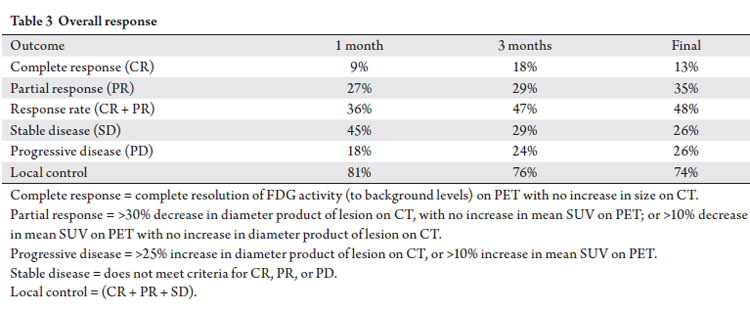
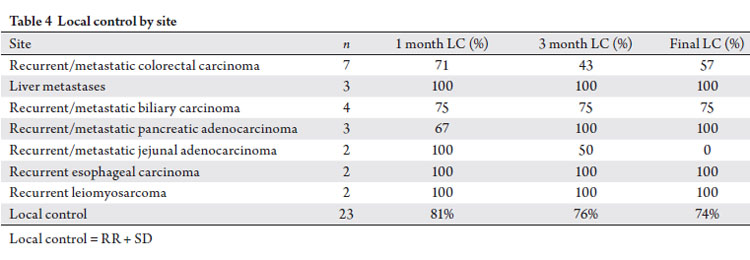
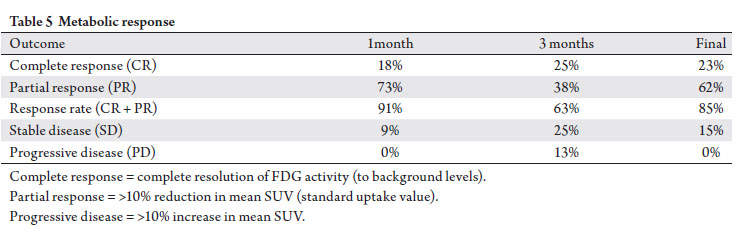
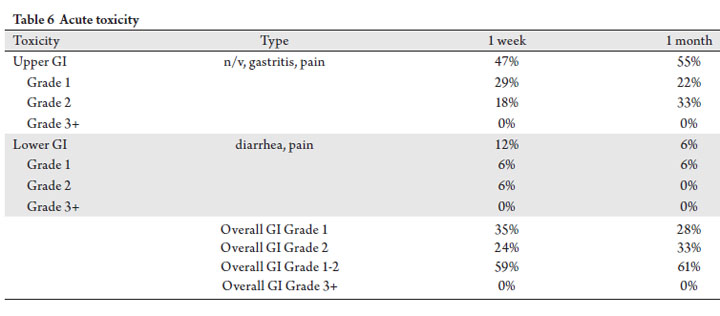
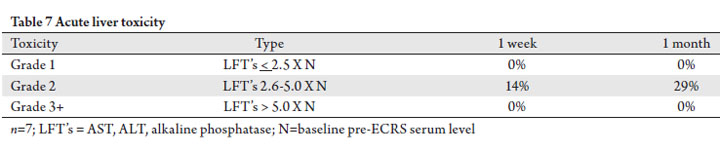
|
Discussion
In this retrospective review, we report on the outcome
of patients treated with hypofractionated image-guided
stereotactic body radiotherapy for oligometastatic and
recurrent abdomino-pelvic malignancies at the Emory Clinic.
In the 20 patients treated (23 individually treated sites),
with a median follow-up of 6.3 months, local control was
74%. Local failures tended to occur within the treated area
(encompassed by the PTV), and did not indicate “marginal
misses.” 30% of the patients on this study did receive post-
SBRT systemic chemotherapy, though the majority of these
cases were in patients who showed evidence of progression
after SBRT. Historically, this local control value is somewhat
less than that expected by cranial radiosurgery (23, 24),
although in the majority of cases no other local treatment
options were available for the patients in this study. The doses in this study ranged from 15-25 Gy, the majority delivered in
a single fraction. These single-fraction treated patients were
part of an institutional dose escalation protocol, while those
patients that received 2 or 3 fractions had previously received
external beam radiotherapy in the treated area. As toxicity
was relatively mild (discussed below), this may indicate room
for dose escalation and or investigation of hypofractionation
over 2-3 treatments in order to deliver a higher effective
dose. A recent phase I study of SBRT for HCC-IHC has been
reported, with dose hypofractionation over 6 treatments
to 24-54 Gy (mean 36 Gy), with acceptable toxicity (19).
Currently there is an ongoing RTOG phase I SBRT study for
liver metastases, incorporating 10 fractions (28).
Although there has been a recent trend to treat cranial
radiosurgery with a frameless setup, the majority of SRS
treatments are still performed with a stereotactic head frame.
Cranial SRS treatment also has the advantage of a relatively
immobile intrafraction target. For cases in the abdomen, in
order to reduce the setup PTV margin, potentially reduce
surrounding tissue dose, and achieve the same precision as
SRS, image-guidance should be an essential component of
abdomino-pelvic radiosurgery. In this series, the majority
of patients’ setup was verified at the time of radiosurgery
with radio-opaque markers implanted at the periphery of
the target. These markers, along with bony anatomy, were
used for on board imaging using kv-kv image matching. This procedure, which typically involved the placement of
3 markers, was performed by interventional radiology and
no complications were reported its use. For those patients
who refused the implantable markers, or whose placement
was deemed to encompass excessive procedural risk, image
guidance was performed with cone beam CT for soft tissue
matching.
Significant intrafraction respiratory motion for targets
in the upper abdomen has been demonstrated (25). While
this motion may have a moderate effect of daily fractionated
treatment, the uncertainty imposed by this organ motion
could potentially compromise target coverage with relatively
tight PTV margins. In order to maintain a small PTV margin
and reduce normal tissue toxicity for lesions in the upper
abdomen, respiratory motion should be accounted for in
the radiosurgical treatment of these lesions. In this series,
patients with targets in the upper abdomen (pancreas, liver,
small bowel) were simulated with a 4D-CT, and planned and
treated at end expiration. The use of implanted fiducial radioopaque
markers has the added advantage of matching these
markers with respiration using real time on board imaging to
verify treatment location and respiration. While cone beam
CT has the advantage of soft tissue matching, at least at our
clinic, we have not been able to incorporate this technology
with respiratory gating for treatment. As such, cone beam
CT was reserved for lower abdomen/pelvic targets, or
those patients who could not receive the implanted fiducial
markers.
Using a combination of RECIST and the updated
lymphoma response criteria (20-22), the overall response rate
in this series was 48%. This value is a sum of the complete
responders and partial responders, and incorporates the
change in the diameter product on CT as well as change in
maximum SUV on FDG-PET. Using the same criteria, the
rate of disease progression at the treated site was 26%. Early
response (PR or CR at 1-month) appeared to correlate with
a durable response, as 84% of those patients with an early
treatment response maintained local control at last followup.
In addition, the based on change in maximum SUV on
FDG-PET, the metabolic response rate was 85%, suggesting a
strong functional response to the radiosurgery. Furthermore,
no patients evaluable in this fashion showed evidence of
metabolic progression after treatment. In other studies and
observations, a “flare” phenomenon has been reported, in
which there may be a transient increase in metabolic activity
as measured by FDG-PET, followed by a reduction in
metabolic activity (26, 27). This is thought to be most likely
due to an inflammatory reaction. However, only 2 treated
sites (both in the same patient) exhibited this phenomenon
in our series, with a transient increase in maximum SUV at 3
months, followed by reduction in values to a point lower than that seen on the pre-SBRT FDG-PET scan.
Mild acute gastrointestinal toxicity was common in our
study, both at 1 week (59%) and 1 month (61%) followup;
however, no patient experienced grade 3 or greater
gastrointestinal toxicity. Among those patients with
symptoms, the most common symptoms were pain (58%)
and nausea (50%). These were relatively well controlled
with supportive medication. At longer follow-up, these
symptoms tended to resolve (data not reported). One patient
who received a single fraction of 25 Gy did develop a grade
2 gastric ulcer, which was managed conservatively with
medication only. As part of a related institutional phase I
dose escalation protocol, seven patients received radiosurgery
within or adjacent to the liver parenchyma. Two patients
experienced grade 2 liver toxicity, with an elevation alkaline
phosphatase over pre-SBRT levels. Both of these patients also
experienced locoregional disease progression with biliary
obstruction, which may have contributed to the elevation
in LFT’s. No other patients experienced measurable liver
toxicity.
In this retrospective series, the use of hypofractionated
image-guided stereotactic body radiotherapy (extracranial
radiosurgery) for oligometastatic and recurrent abdominopelvic
malignancies resulted in excellent short-term
local control rates, with frequent but mild acute toxicity.
The short-term response rate was also excellent, as was
metabolic response as measured by FDG-PET. Although a
single fraction treatment offers certain logistic advantages,
there may be room for improved local control with dose
escalation or further fractionation, as treatment toxicity was
relatively mild. There may also be a benefit for treatment of
gastrointestinal malignancies in the primary curative setting,
with dose escalation boosts to a small treatment area. While
longer follow-up studies are warranted, for patients without
other local therapy options, these results suggest that this
type of radiosurgery may offer a significant clinical benefit.
|
References
- Hellman S, Weichselbaum RR. Oligometastases. J Clin Oncol
1995;13:8-10.[LinkOut]
- Mehta N, Mauer AM, Hellman S, Haraf DJ, Cohen EE, Vokes EE, et
al. Analysis of further disease progression in metastatic non-small
cell lung cancer: implications for locoregional treatment. Int J Oncol
2004;25:1677-83.[LinkOut]
- Long-term results of lung metastasectomy: prognostic analyses based
on 5206 cases. The International Registry of Lung Metastases. J Thorac
Cardiovasc Surg 1997;113:37-49.[LinkOut]
- Fong Y, Cohen AM, Fortner JG, Enker WE, Turnbull AD, Coit DG, et al.
Liver resection for colorectal metastases. J Clin Oncol 1997;15:938-46.[LinkOut]
- Miller G, Biernacki P, Kemeny NE, Gonen M, Downey R, Jarnagin WR, et al. Outcomes after resection of synchronous or metachronous hepatic and
pulmonary colorectal metastases. J Am Coll Surg 2007;205:231-8.[LinkOut]
- Strong VE, D’Angelica M, Tang L, Prete F, Gönen M, Coit D, et al.
Laparoscopic adrenalectomy for isolated adrenal metastasis. Ann Surg
Oncol 2007;14:3392-400.[LinkOut]
- Onishi H, Araki T, Shirato H, Nagata Y, Hiraoka M, Gomi K, et al.
Stereotactic hypofractionated high-dose irradiation for stage I nonsmall
cell lung carcinoma: clinical outcomes in 245 subjects in a Japanese
multiinstitutional study. Cancer 2004;101:1623-31.[LinkOut]
- Wulf J, Haedinger U, Oppitz U, Thiele W, Mueller G, Flentje M. Stereotactic
radiotherapy for primary lung cancer and pulmonary metastases: a
noninvasive treatment approach in medically inoperable patients. Int J
Radiat Oncol Biol Phys 2004;60:186-96.[LinkOut]
- Okunieff P, Petersen AL, Philip A, Milano MT, Katz AW, Boros L, et al.
Stereotactic Body Radiation Therapy (SBRT) for lung metastases. Acta
Oncol 2006;45:808-17.[LinkOut]
- Joyner M, Salter BJ, Papanikolaou N, Fuss M. Stereotactic body radiation
therapy for centrally located lung lesions. Acta Oncol 2006;45:802-7.[LinkOut]
- Xia T, Li H, Sun Q, Wang Y, Fan N, Yu Y, et al. Promising clinical outcome
of stereotactic body radiation therapy for patients with inoperable Stage I/
II non-small-cell lung cancer. Int J Radiat Oncol Biol Phys 2006;66:117-25.[LinkOut]
- Ryu S, Rock J, Rosenblum M, Kim JH. Patterns of failure after single-dose
radiosurgery for spinal metastasis. J Neurosurg 2004;101 Suppl 3:402-5.[LinkOut]
- Gerszten PC, Ozhasoglu C, Burton SA, Vogel WJ, Atkins BA, Kalnicki S, et
al. CyberKnife frameless stereotactic radiosurgery for spinal lesions: clinical
experience in 125 cases. Neurosurgery 2004;55:89-98; discussion 98-9.[LinkOut]
- Rock JP, Ryu S, Shukairy MS, Yin FF, Sharif A, Schreiber F, et al.
Postoperative radiosurgery for malignant spinal tumors. Neurosurgery
2006;58:891-8; discussion 891-8.[LinkOut]
- Sahgal A, Larson DA, Chang EL. Stereotactic body radiosurgery for spinal
metastases: a critical review. Int J Radiat Oncol Biol Phys 2008;71:652-65.[LinkOut]
- Schefter TE, Kavanagh BD, Timmerman RD, Cardenes HR, Baron A,
Gaspar LE. A phase I trial of stereotactic body radiation therapy (SBRT)
for liver metastases. Int J Radiat Oncol Biol Phys 2005;62:1371-8.[LinkOut]
- Kavanagh BD, Schefter TE, Cardenes HR, Stieber VW, Raben D,
Timmerman RD, et al. Interim analysis of a prospective phase I/II trial of
SBRT for liver metastases. Acta Oncol 2006;45:848-55.[LinkOut]
- Wulf J, Guckenberger M, Haedinger U, Oppitz U, Mueller G, Baier K, et al.
Stereotactic radiotherapy of primary liver cancer and hepatic metastases. Acta Oncol 2006;45:838-47.[LinkOut]
- Tse RV, Hawkins M, Lockwood G, Kim JJ, Cummings B, Knox J, et
al. Phase I study of individualized stereotactic body radiotherapy for
hepatocellular carcinoma and intrahepatic cholangiocarcinoma. J Clin
Oncol 2008;26:657-64.[LinkOut]
- Therasse P, Arbuck SG, Eisenhauer EA, Wanders J, Kaplan RS, Rubinstein
L, et al. New guidelines to evaluate the response to treatment in solid
tumors. European Organization for Research and Treatment of Cancer,
National Cancer Institute of the United States, National Cancer Institute of
Canada. J Natl Cancer Inst 2000;92:205-16.[LinkOut]
- Cheson BD, Pfistner B, Juweid ME, Gascoyne RD, Specht L, Horning
SJ, et al. Revised response criteria for malignant lymphoma. J Clin Oncol
2007;25:579-86.[LinkOut]
- Juweid ME, Stroobants S, Hoekstra OS, Mottaghy FM, Dietlein M,
Guermazi A, et al. Use of positron emission tomography for response
assessment of lymphoma: consensus of the Imaging Subcommittee
of International Harmonization Project in Lymphoma. J Clin Oncol
2007;25:571-8.[LinkOut]
- Coia LR, Aaronson N, Linggood R, Loeffler J, Priestman TJ. A report of
the consensus workshop panel on the treatment of brain metastases. Int J
Radiat Oncol Biol Phys 1992;23:223-7.[LinkOut]
- Flickinger JC, Kondziolka D, Lunsford LD, Coffey RJ, Goodman ML, Shaw
EG, et al. A multi-institutional experience with stereotactic radiosurgery for
solitary brain metastasis. Int J Radiat Oncol Biol Phys 1994;28:797-802.[LinkOut]
- Gierga DP, Chen GT, Kung JH, Betke M, Lombardi J, Willett CG.
Quantification of respiration-induced abdominal tumor motion and
its impact on IMRT dose distributions. Int J Radiat Oncol Biol Phys
2004;58:1584-95.[LinkOut]
- Wade AA, Scott JA, Kuter I, Fischman AJ. Flare response in 18F-fluoride
ion PET bone scanning. AJR Am J Roentgenol 2006;186:1783-6.[LinkOut]
- Basu S, Alavi A. Defining co-related parameters between ‘metabolic’
flare and ‘clinical’, ‘biochemical’, and ‘osteoblastic’ flare and establishing
guidelines for assessing response to treatment in cancer. Eur J Nucl Med
Mol Imaging 2007;34:441-3.[LinkOut]
- Katz AW, Dawson LA et al. Radiation Therapy Oncology Group RTOG
0438, a phase I trial of highly conformal radiation therapy for patients with
liver metastasis. www.rtog.org/members/protocols/0438/0438.pdf. [LinkOut]
Cite this article as:
Perkins C, El-Reyes B, Simon E, Kooby D, Torres W, Kauh J, Staley C, Landry J. Single-fraction image-guided extracranial radiosurgery for recurrent and metastatic abdominal and pelvic cancers: shortterm local control, metabolic response, and toxicity. J Gastrointest Oncol. 2010;1(1):16-23. DOI:10.3978/j.issn.2078-6891.2010.010
|