Biliary cancer: intrahepatic cholangiocarcinoma vs. extrahepatic cholangiocarcinoma vs. gallbladder cancers: classification and therapeutic implications
Introduction
Biliary cancers (BCs) are rare but heterogeneous, and comprised of intrahepatic cholangiocarcinoma (IHCC), extrahepatic cholangiocarcinoma (EHCC) and gallbladder cancers. They may arise along any portion of the bile duct system, from the neoplastic proliferation of cholangiocytes, the epithelium of the bile ducts. While all three anatomic groups fall under BC, our increased understanding has allowed us to rationalize that each may be in fact distinct diseases, with differences in patterns of recurrence and prognosis (1). Pre-clinical studies have reinforced this thought process, where immunohistochemical studies from BC samples revealed phenotypic traits of cholangiocytes and progenitor cells consistent with their anatomic sites of origin, confirming the heterogeneity between the three groups (2). Progenitor cells from the canals of Hering have been identified in IHCC, while those within the peribiliary glands have been identified in EHCC and gallbladder cancers, respectively (2-5). Furthermore, the increased availability of next-generation sequencing panels has facilitated this thought process by allowing us to understand the tumor somatic variants and genomic heterogeneity between the three groups. Herein, we will the current treatment in BC, genomic landscape in BC and its role in treatment selection and integration in clinical trials.
Therapeutic regimens for biliary tract cancer
Chemotherapeutic treatment options
In patients who are diagnosed with early stage disease, surgical resection, regardless of anatomic location, is the sole curative treatment option (6). Unfortunately, most patients present with advanced disease at diagnosis, where no curative treatments exist and are primarily supportive. Based on ABC-02, a large, randomized stage III trial conducted by Valle et al., the combination of gemcitabine and platinum based chemotherapy has become the standard approach in treating advanced BC, for all anatomic groups. The combination demonstrated superior clinical efficacy when compared to single-agent gemcitabine (7). Patients who received the combination experienced an absolute 3.6 months survival benefit in comparison to gemcitabine monotherapy with similar rates of adverse events. Eighty percent of patients experienced disease control. Despite this, the vast majority of patients develop treatment resistance after few months on therapy and the overall survival (OS) remains at less than 1 year (7). Outcomes in second-line therapies after gemcitabine platinum-based therapy failure result in dismal outcomes with progression-free survival (PFS) only several months, highlighting the need to develop new and effective therapies in BTC (Table 1) (8-14).
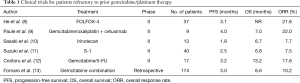
Full table
Targeted therapies and the role of cancer genomics in its treatment selection process
Over the past two decades, the discovery and successful targeting of genes involved in oncogenesis has helped increase our understanding of targeted management of solid tumor malignancies. Pre-clinical studies revealing a high proportion of genomic alterations in critical signaling pathways involved in tumor proliferation, growth and treatment resistance has led to an interest in developing clinical trials investigating novel targeted therapies in BC.
While early studies investigating novel agents targeting relevant signaling pathways demonstrated interesting anti-tumor activity in a small proportion of patients, the overall results have been disappointing, with outcomes largely similar to chemotherapy agents in the refractory setting (Table 2) (15-25). This may be in part due to the utilization of non-selected trials, where patients are eligible for enrollment regardless of their tumor genomic alterations. Furthermore, the challenge to have sufficient enrollment and timely completion of clinical trials has led to lumping all sites of BC into its eligibility, irrespective of anatomic subtype.
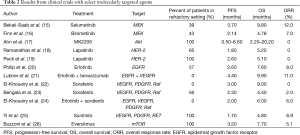
Full table
Through the availability and implementation of next generation sequencing technologies, recent reports have allowed us to understand the genomic landscape present in BC, as well as understand and identify actionable molecular alterations in this disease. While studies have identified common mutations in KRAS (up to 20%), BRAF (5%) and epidermal growth factor receptor (EGFR) (3%) in gallbladder, IHCC and EHCCs, targetable mutations are enriched in IHCC that have only been identified in this anatomic group, suggesting differing influences in pathogenesis and reinforcing the thought process that BC is comprised of three heterogenous diseases. Herein, we briefly discuss common targetable mutations, while focusing on recently identified genes that may be treated with novel, targeted therapies.
ErbB family signaling pathway in BC
Four receptors belong to the ErbB family of receptor tyrosine kinases: EGFR, ErbB-2 (HER-2 or HER-2/neu), ErbB-3 (HER-3) and ErbB-4 (HER-4). ErbB receptors and the binding to its ligands are integral to biliary carcinogenesis. Anti EGFR and HER-2 therapies have demonstrated interesting anti-tumor activity in preclinical studies (27), resulting in their investigation in clinical trials.
EGFR signaling pathway
Preclinical work has identified EGFR to be aberrantly activated in BC, across all three anatomic groups (28,29). The EGFR signaling pathway is integral in epithelial cell growth and proliferation, where preclinical studies have shown tumor regression with EGFR inhibition (28,30), forming the rationale for targeting EGFR for treatment. While initial small studies demonstrated promising anti-tumor activity with anti-EGFR therapy in combination with gemcitabine-based chemotherapy (31,32), these findings were unable to translate into a significant clinical benefit in larger randomized clinical trials (33,34).
One rationale for the limited clinical activity seen with anti-EGFR therapies may be due to the activation of downstream effectors, including the PI3K/Akt and mitogen activated protein kinase (MAPK) pathways. The MAPK pathway is a downstream signaling pathway, where upstream receptor tyrosine kinases (EGFR, TGF-β, etc.) result in the phosphorylation, activation and signal transduction via Ras, Raf, MEK and ERK. Activated ERK (pERK) translocates to the nucleus and affects many cellular responses. In the PI3K/Akt pathway, PI3K activates Akt (pAkt), which mediates its effects through various downstream substrates. Activation of Akt and ERK contribute to tumor proliferation, invasiveness and chemotherapy resistance, representing rational therapeutic targets against these pathways (35,36).
Both pathways are frequently activated, often concomitantly in all subtypes of BC (37-39). While the overall results of several studies investigating were unimpressive, interesting clinical activity was observed in several patients with advanced, refractory BC who received MEK and Akt inhibitors (15,16,40). The clinical benefit from targeting single signaling pathways is often short lived due to mechanisms of resistance including communication between parallel signaling pathways, activation of downstream effectors and negative loop feedback inhibition.
HER-2/neu gene
Human growth factor receptor 2, also known as HER-2/neu or ERBB2, is an oncogene encoded by the ERBB2 gene, and is a member of the human EGFR family. Its amplification or overexpression has been shown to play an important role in the development and progression of several solid tumor malignancies, and is an effective therapeutic target in breast and gastric cancer (41-43). Studies evaluating HER-2 overexpression in BC have identified HER-2 mutations in gallbladder (about 10%) and EHCCs (up to 25%), and have been associated with a more aggressive phenotype (27,44). While small case series demonstrated anti-tumor activity with anti HER-2 directed therapy in patients whose tumors expressed HER-2 overexpression or amplification, these findings did not translate in BC patients (18,19,45,46). However, these studies were not limited to HER-2 overexpressing tumors and allowed all comers. Improved patient selection, in addition to dual anti HER-2 targeted therapies may result in improved clinical efficacy, and are a consideration for future clinical trials.
Vascular endothelial growth factor (VEGF) pathway in BC
Angiogenesis is a mechanism by which tumor cells can proliferate and is controlled by VEGF. In addition to angiogenesis and vascular permeability, VEGF mediated signaling facilitates signaling in tumor cells that contribute to cholangiocarcinogenesis, including facilitating the function of tumor stem cells, tumor invasiveness and modulate regulatory T cells that can contribute to treatment resistance (47). It is highly expressed in BC, in up to 75%, and is associated with a more aggressive phenotype and poorer prognosis (48). Therapies targeting VEGF, including monoclonal antibodies against VEGF (bevacizumab) or multi-targeted small molecule inhibitors with activity against VEGFR have failed to demonstrate any significant clinical activity in randomized clinical trials in BC (21,49-52). While there is no indication for anti-VEGF therapies in BC at this time, given its activity in other solid tumors, it remains a therapeutic target of interest. The identification of prognostic and predictive biomarkers may improve patient selection and identify patients who are likely to benefit from anti-VEGF therapies.
Novel genomic alterations identified in IHCC
BRAF mutations
As described above, the MAPK pathway regulates cellular proliferation, survival and migration and is constitutively activated in BC. Mutation of the BRAF gene is a mechanism of aberrant activation of the MAPK pathway and occurs in many solid tumors, including melanoma, colorectal cancer and non-small cell lung cancer (53-56). The most common BRAF mutation results in a single amino acid substitution of glutamic acid to valine at residue 600 (V600E). Larger studies suggest BRAF V600E mutations are exclusive to IHCC, with an incidence of 3–5% (57,58). The presence of a BRAF mutation has been associated with a more aggressive phenotype, with a higher tumor stage and likelihood of lymph node involvement at diagnosis (59). While inhibition of BRAF with small molecule inhibitors have demonstrated anti-tumor activity in various other BRAF-mutated malignancies and have become a standardized treatment in BRAF-mutated melanoma, its therapeutic relevance in IHCC is unknown, but represents an intriguing therapeutic option for this disease (60-66). Ongoing early clinical trials are investigating the role of BRAF mutations as a potential targetable mutation in BC (Table 3).
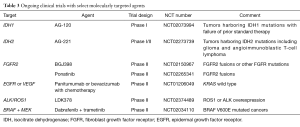
Full table
Isocitrate dehydrogenase 1/2 (IDH1/2)
IDH1 and IDH2 function to encode metabolic enzymes that normally convert isocitrate, a metabolic intermediate, to α-ketoglutarate. Somatic mutations in IDH1/2 can occur in upwards of 20% of IHCC and result in abnormal enzymatic activity, allowing them to reduce α-ketoglutarate to 2-hydroxyglutarate, which has been identified as an “oncometabolite” that inhibits enzymatic activity dependent on α-ketoglutarate (67-69). This results in altered cell maturation, differentiation and survival. Pre-clinical studies have confirmed the role of IDH mutations in the pathogenesis of intrahepatic BC, where their mutations inhibit hepatocyte differentiation and induce proliferation of hepatic progenitors and the development of premalignant biliary lesions (70). Based on early promising results in preclinical studies, specific inhibitors for IDH1 and IDH2 are currently under investigation in clinical trials (Table 3).
Fibroblast growth factor receptor (FGFR)
The FGFR comprise a family of highly conserved tyrosine kinase receptors consisting of four members (FGFR1, 2, 3 and 4). These receptors bind to one of 18 secreted glycoprotein ligands, or fibroblast growth factors (FGFs), to their extracellular domain (71). Several FGFR2 chromosomal fusions, with several genomic partners, have been identified in IHCC (72-77). Preclinical studies have identified several FGFR2 fusions (FGFR2-PPHLN1, FGFR2-BICC1, FGFR2-TACC3, AHCYL1) specifically in intrahepatic BC, where genomic sequencing identified an incidence ranging up to 50% of intrahepatic BC patients. Upon its fusion, activation of the tyrosine kinase protein results in the activation of the FGFR2 receptor with autophosphorylation and activation of downstream signaling pathways, including Ras/MAPK, PI3K/Akt and the signaling transducer and activator of transcription (STAT) pathway, which play a critical role in the regulation of cell proliferation, differentiation, and survival (78). Additionally, identification of an association between the presence of KRAS mutations and FGFR2 fusions suggest a mutual role in driving oncogenesis.
While pre-clinical studies and isolated case reports have demonstrated anti-tumor activity with FGFR inhibition in intrahepatic BC exhibiting FGFR2 fusions, its actual role in oncogenesis and its sensitivity as a targetable actionable mutation is unknown. Ongoing phase II studies, specifically in BC patients with FGFR2 mutations are ongoing and may reveal the potential therapeutic benefit from FGFR inhibition (Table 3).
ROS1 gene
ROS1 fusions, a proto-oncogene, have been identified in up to 9% of IHCC (79). Its role as an oncogenic driver and potential therapeutic target has been recently validated in preclinical studies, where its inactivation led to a potent anti-tumor effect (80). While its role as a potential therapeutic target has been confirmed in other solid tumor malignancies, notably non-small cell lung cancer (81), further validation will be required to assess its frequency in BC in addition to assessing its relevancy as a potential targetable mutation in patients exhibiting ROS1 fusions.
Notch pathway
The Notch signaling pathway plays an important role in embryogenesis and the proper structural development of the liver. It is also plays an important role in oncogenesis, where its dysregulation results in increased inflammation and development in intrahepatic BC. Mouse models with increased Notch1 expression resulted in the formation of intrahepatic BC, reinforcing its importance in tumor development and growth (82). Notch receptor 1–4 upregulation has been identified in all anatomic subsets of BC, and in up to 80% of intrahepatic BC (83,84). In addition to its role in BC, Notch expression has also been implicated in the development of primary hepatocellular carcinomas (HCC). Interestingly, a study utilizing mouse models driven by Ras, demonstrated that the inhibition of specific Notch receptors resulted in reducing HCC like primary tumors, while leading to the development of primary BC like tumors (85). Thus, while targeting Notch represents an intriguing therapeutic option in BC, further studies are needed to validate its role in this disease.
Conclusions
BCs are a rare, heterogeneous disease group that have limited treatment options and are associated with universally poor outcomes. Recent advances in sequencing technologies have resulted in an increased understanding of the genomic alterations present in BC, and understand the differences in the genomic makeup in each anatomic subgroup. Through these efforts, the identification of targetable mutations has allowed us to develop and tailor novel therapeutic agents against these genes of interest. The completion of ongoing prospective trials may result in the shift in the treatment paradigm in BC, where patients will be able to receive treatment tailored specifically based on each individual’s tumor genomic profile. Prospective correlative studies that include the utilization of a biorepository will allow us to understand mechanisms of treatment efficacy, study the process of treatment resistance, and importantly, identifying biomarkers of secondary resistance that may allow for development of alternate therapeutic options.
Acknowledgements
None.
Footnote
Conflicts of Interest: The authors have no conflicts of interest to declare.
References
- Jarnagin WR, Ruo L, Little SA, et al. Patterns of initial disease recurrence after resection of gallbladder carcinoma and hilar cholangiocarcinoma: implications for adjuvant therapeutic strategies. Cancer 2003;98:1689-700. [Crossref] [PubMed]
- Cardinale V, Carpino G, Reid L, et al. Multiple cells of origin in cholangiocarcinoma underlie biological, epidemiological and clinical heterogeneity. World J Gastrointest Oncol 2012;4:94-102. [Crossref] [PubMed]
- Theise ND, Saxena R, Portmann BC, et al. The canals of Hering and hepatic stem cells in humans. Hepatology 1999;30:1425-33. [Crossref] [PubMed]
- Cardinale V, Wang Y, Carpino G, et al. Multipotent stem/progenitor cells in human biliary tree give rise to hepatocytes, cholangiocytes, and pancreatic islets. Hepatology 2011;54:2159-72. [Crossref] [PubMed]
- Carpino G, Cardinale V, Onori P, et al. Biliary tree stem/progenitor cells in glands of extrahepatic and intraheptic bile ducts: an anatomical in situ study yielding evidence of maturational lineages. J Anat 2012;220:186-99. [Crossref] [PubMed]
- Bridgewater J, Galle PR, Khan SA, et al. Guidelines for the diagnosis and management of intrahepatic cholangiocarcinoma. J Hepatol 2014;60:1268-89. [Crossref] [PubMed]
- Valle J, Wasan H, Palmer DH, et al. Cisplatin plus gemcitabine versus gemcitabine for biliary tract cancer. N Engl J Med 2010;362:1273-81. [Crossref] [PubMed]
- He S, Shen J, Sun X, et al. A phase II FOLFOX-4 regimen as second-line treatment in advanced biliary tract cancer refractory to gemcitabine/cisplatin. J Chemother 2014;26:243-7. [Crossref] [PubMed]
- Paule B, Herelle MO, Rage E, et al. Cetuximab plus gemcitabine-oxaliplatin (GEMOX) in patients with refractory advanced intrahepatic cholangiocarcinomas. Oncology 2007;72:105-10. [Crossref] [PubMed]
- Sasaki T, Isayama H, Nakai Y, et al. A pilot study of salvage irinotecan monotherapy for advanced biliary tract cancer. Anticancer Res 2013;33:2619-22. [PubMed]
- Suzuki E, Ikeda M, Okusaka T, et al. A multicenter phase II study of S-1 for gemcitabine-refractory biliary tract cancer. Cancer Chemother Pharmacol 2013;71:1141-6. [Crossref] [PubMed]
- Croitoru A, Gramaticu I, Dinu I, et al. Fluoropyrimidines plus cisplatin versus gemcitabine/gemcitabine plus cisplatin in locally advanced and metastatic biliary tract carcinoma - a retrospective study. J Gastrointestin Liver Dis 2012;21:277-84. [PubMed]
- Fornaro L, Vivaldi C, Cereda S, et al. Second-line chemotherapy in advanced biliary cancer progressed to first-line platinum-gemcitabine combination: a multicenter survey and pooled analysis with published data. J Exp Clin Cancer Res 2015;34:156. [Crossref] [PubMed]
- Bridgewater J, Palmer D, Cunningham D, et al. Outcome of second-line chemotherapy for biliary tract cancer. Eur J Cancer 2013;49:1511. [Crossref] [PubMed]
- Bekaii-Saab T, Phelps MA, Li X, et al. Multi-institutional phase II study of selumetinib in patients with metastatic biliary cancers. J Clin Oncol 2011;29:2357-63. [Crossref] [PubMed]
- Finn R, Javle M, Tan B, et al. A Phase I Study of MEK Inhibitor MEK162 (ARRY-438162) in patients with biliary tract cancer. J Clin Oncol 2012;30:abstr 220.
- Ahn DH, Li J, Wei L, et al. Results of an abbreviated phase-II study with the Akt Inhibitor MK-2206 in Patients with Advanced Biliary Cancer. Sci Rep 2015;5:12122. [Crossref] [PubMed]
- Ramanathan RK, Belani CP, Singh DA, et al. A phase II study of lapatinib in patients with advanced biliary tree and hepatocellular cancer. Cancer Chemother Pharmacol 2009;64:777-83. [Crossref] [PubMed]
- Peck J, Wei L, Zalupski M, et al. HER2/neu may not be an interesting target in biliary cancers: results of an early phase II study with lapatinib. Oncology 2012;82:175-9. [Crossref] [PubMed]
- Philip PA, Mahoney MR, Allmer C, et al. Phase II study of erlotinib in patients with advanced biliary cancer. J Clin Oncol 2006;24:3069-74. [Crossref] [PubMed]
- Lubner SJ, Mahoney MR, Kolesar JL, et al. Report of a multicenter phase II trial testing a combination of biweekly bevacizumab and daily erlotinib in patients with unresectable biliary cancer: a phase II Consortium study. J Clin Oncol 2010;28:3491-7. [Crossref] [PubMed]
- El-Khoueiry AB, Rankin CJ, Ben-Josef E, et al. SWOG 0514: a phase II study of sorafenib in patients with unresectable or metastatic gallbladder carcinoma and cholangiocarcinoma. Invest New Drugs 2012;30:1646-51. [Crossref] [PubMed]
- Bengala C, Bertolini F, Malavasi N, et al. Sorafenib in patients with advanced biliary tract carcinoma: a phase II trial. Br J Cancer 2010;102:68-72. [Crossref] [PubMed]
- El-Khoueiry AB, Rankin C, Siegel AB, et al. S0941: a phase 2 SWOG study of sorafenib and erlotinib in patients with advanced gallbladder carcinoma or cholangiocarcinoma. Br J Cancer 2014;110:882-7. [Crossref] [PubMed]
- Yi JH, Thongprasert S, Lee J, et al. A phase II study of sunitinib as a second-line treatment in advanced biliary tract carcinoma: a multicentre, multinational study. Eur J Cancer 2012;48:196-201. [Crossref] [PubMed]
- Buzzoni R, Pusceddu S, Bajetta E, et al. Activity and safety of RAD001 (everolimus) in patients affected by biliary tract cancer progressing after prior chemotherapy: a phase II ITMO study. Ann Oncol 2014;25:1597-603. [Crossref] [PubMed]
- Pignochino Y, Sarotto I, Peraldo-Neia C, et al. Targeting EGFR/HER2 pathways enhances the antiproliferative effect of gemcitabine in biliary tract and gallbladder carcinomas. BMC Cancer 2010;10:631. [Crossref] [PubMed]
- Xu L, Hausmann M, Dietmaier W, et al. Expression of growth factor receptors and targeting of EGFR in cholangiocarcinoma cell lines. BMC Cancer 2010;10:302. [Crossref] [PubMed]
- Yoon JH, Gwak GY, Lee HS, et al. Enhanced epidermal growth factor receptor activation in human cholangiocarcinoma cells. J Hepatol 2004;41:808-14. [Crossref] [PubMed]
- Yoshikawa D, Ojima H, Kokubu A, et al. Vandetanib (ZD6474), an inhibitor of VEGFR and EGFR signalling, as a novel molecular-targeted therapy against cholangiocarcinoma. Br J Cancer 2009;100:1257-66. [Crossref] [PubMed]
- Gruenberger B, Schueller J, Heubrandtner U, et al. Cetuximab, gemcitabine, and oxaliplatin in patients with unresectable advanced or metastatic biliary tract cancer: a phase 2 study. Lancet Oncol 2010;11:1142-8. [Crossref] [PubMed]
- Sohal DP, Mykulowycz K, Uehara T, et al. A phase II trial of gemcitabine, irinotecan and panitumumab in advanced cholangiocarcinoma. Ann Oncol 2013;24:3061-5. [Crossref] [PubMed]
- Lee J, Park SH, Chang HM, et al. Gemcitabine and oxaliplatin with or without erlotinib in advanced biliary-tract cancer: a multicentre, open-label, randomised, phase 3 study. Lancet Oncol 2012;13:181-8. [Crossref] [PubMed]
- Malka D, Cervera P, Foulon S, et al. Gemcitabine and oxaliplatin with or without cetuximab in advanced biliary-tract cancer (BINGO): a randomised, open-label, non-comparative phase 2 trial. Lancet Oncol 2014;15:819-28. [Crossref] [PubMed]
- Tanno S, Yanagawa N, Habiro A, et al. Serine/threonine kinase AKT is frequently activated in human bile duct cancer and is associated with increased radioresistance. Cancer Res 2004;64:3486-90. [Crossref] [PubMed]
- Yoon H, Min JK, Lee JW, et al. Acquisition of chemoresistance in intrahepatic cholangiocarcinoma cells by activation of AKT and extracellular signal-regulated kinase (ERK)1/2. Biochem Biophys Res Commun 2011;405:333-7. [Crossref] [PubMed]
- Deshpande V, Nduaguba A, Zimmerman SM, et al. Mutational profiling reveals PIK3CA mutations in gallbladder carcinoma. BMC Cancer 2011;11:60. [Crossref] [PubMed]
- Li Q, Yang Z. Expression of phospho-ERK1/2 and PI3-K in benign and malignant gallbladder lesions and its clinical and pathological correlations. J Exp Clin Cancer Res 2009;28:65. [Crossref] [PubMed]
- Schmitz KJ, Lang H, Wohlschlaeger J, et al. AKT and ERK1/2 signaling in intrahepatic cholangiocarcinoma. World J Gastroenterol 2007;13:6470-7. [Crossref] [PubMed]
- Bendell JC, Papadopoulos K, Jones SF, et al. A phase I dose-escalation study of MEK inhibitor MEK162 (ARRY-438162) in patients with advanced solid tumors. Mol Cancer Ther 2011;10:abstr B243.
- Verma S, Miles D, Gianni L, et al. Trastuzumab emtansine for HER2-positive advanced breast cancer. N Engl J Med 2012;367:1783-91. [Crossref] [PubMed]
- Bang YJ, Van Cutsem E, Feyereislova A, et al. Trastuzumab in combination with chemotherapy versus chemotherapy alone for treatment of HER2-positive advanced gastric or gastro-oesophageal junction cancer (ToGA): a phase 3, open-label, randomised controlled trial. Lancet 2010;376:687-97. [Crossref] [PubMed]
- Janjigian YY, Werner D, Pauligk C, et al. Prognosis of metastatic gastric and gastroesophageal junction cancer by HER2 status: a European and USA International collaborative analysis. Ann Oncol 2012;23:2656-62. [Crossref] [PubMed]
- Kim HJ, Yoo TW, Park DI, et al. Gene amplification and protein overexpression of HER-2/neu in human extrahepatic cholangiocarcinoma as detected by chromogenic in situ hybridization and immunohistochemistry: its prognostic implication in node-positive patients. Ann Oncol 2007;18:892-7. [Crossref] [PubMed]
- Javle M, Churi C, Kang HC, et al. HER2/neu-directed therapy for biliary tract cancer. J Hematol Oncol 2015;8:58. [Crossref] [PubMed]
- Kawamoto T, Ishige K, Thomas M, et al. Overexpression and gene amplification of EGFR, HER2, and HER3 in biliary tract carcinomas, and the possibility for therapy with the HER2-targeting antibody pertuzumab. J Gastroenterol 2015;50:467-79. [Crossref] [PubMed]
- Goel HL, Mercurio AM. VEGF targets the tumour cell. Nat Rev Cancer 2013;13:871-82. [Crossref] [PubMed]
- Quan ZW, Wu K, Wang J, et al. Association of p53, p16, and vascular endothelial growth factor protein expressions with the prognosis and metastasis of gallbladder cancer. J Am Coll Surg 2001;193:380-3. [Crossref] [PubMed]
- Zhu AX, Meyerhardt JA, Blaszkowsky LS, et al. Efficacy and safety of gemcitabine, oxaliplatin, and bevacizumab in advanced biliary-tract cancers and correlation of changes in 18-fluorodeoxyglucose PET with clinical outcome: a phase 2 study. Lancet Oncol 2010;11:48-54. [Crossref] [PubMed]
- Valle JW, Wasan H, Lopes A, et al. Cediranib or placebo in combination with cisplatin and gemcitabine chemotherapy for patients with advanced biliary tract cancer (ABC-03): a randomised phase 2 trial. Lancet Oncol 2015;16:967-78. [Crossref] [PubMed]
- Lee JK, Capanu M, O'Reilly EM, et al. A phase II study of gemcitabine and cisplatin plus sorafenib in patients with advanced biliary adenocarcinomas. Br J Cancer 2013;109:915-9. [Crossref] [PubMed]
- Santoro A, Gebbia V, Pressiani T, et al. A randomized, multicenter, phase II study of vandetanib monotherapy versus vandetanib in combination with gemcitabine versus gemcitabine plus placebo in subjects with advanced biliary tract cancer: the VanGogh study. Ann Oncol 2015;26:542-7. [Crossref] [PubMed]
- Schindler G, Capper D, Meyer J, et al. Analysis of BRAF V600E mutation in 1,320 nervous system tumors reveals high mutation frequencies in pleomorphic xanthoastrocytoma, ganglioglioma and extra-cerebellar pilocytic astrocytoma. Acta Neuropathol 2011;121:397-405. [Crossref] [PubMed]
- Michaloglou C, Vredeveld LC, Mooi WJ, et al. BRAF(E600) in benign and malignant human tumours. Oncogene 2008;27:877-95. [Crossref] [PubMed]
- Brose MS, Volpe P, Feldman M, et al. BRAF and RAS mutations in human lung cancer and melanoma. Cancer Res 2002;62:6997-7000. [PubMed]
- Davies H, Bignell GR, Cox C, et al. Mutations of the BRAF gene in human cancer. Nature 2002;417:949-54. [Crossref] [PubMed]
- Simbolo M, Fassan M, Ruzzenente A, et al. Multigene mutational profiling of cholangiocarcinomas identifies actionable molecular subgroups. Oncotarget 2014;5:2839-52. [Crossref] [PubMed]
- Goeppert B, Frauenschuh L, Renner M, et al. BRAF V600E-specific immunohistochemistry reveals low mutation rates in biliary tract cancer and restriction to intrahepatic cholangiocarcinoma. Mod Pathol 2014;27:1028-34. [Crossref] [PubMed]
- Robertson S, Hyder O, Dodson R, et al. The frequency of KRAS and BRAF mutations in intrahepatic cholangiocarcinomas and their correlation with clinical outcome. Hum Pathol 2013;44:2768-73. [Crossref] [PubMed]
- Sosman JA, Kim KB, Schuchter L, et al. Survival in BRAF V600-mutant advanced melanoma treated with vemurafenib. N Engl J Med 2012;366:707-14. [Crossref] [PubMed]
- Flaherty KT, Puzanov I, Kim KB, et al. Inhibition of mutated, activated BRAF in metastatic melanoma. N Engl J Med 2010;363:809-19. [Crossref] [PubMed]
- Falchook GS, Long GV, Kurzrock R, et al. Dabrafenib in patients with melanoma, untreated brain metastases, and other solid tumours: a phase 1 dose-escalation trial. Lancet 2012;379:1893-901. [Crossref] [PubMed]
- Hauschild A, Grob JJ, Demidov LV, et al. Dabrafenib in BRAF-mutated metastatic melanoma: a multicentre, open-label, phase 3 randomised controlled trial. Lancet 2012;380:358-65. [Crossref] [PubMed]
- Rush S, Foreman N, Liu A. Brainstem ganglioglioma successfully treated with vemurafenib. J Clin Oncol 2013;31:e159-60. [Crossref] [PubMed]
- Kim KB, Cabanillas ME, Lazar AJ, et al. Clinical responses to vemurafenib in patients with metastatic papillary thyroid cancer harboring BRAF(V600E) mutation. Thyroid 2013;23:1277-83. [Crossref] [PubMed]
- Dietrich S, Glimm H, Andrulis M, et al. BRAF inhibition in refractory hairy-cell leukemia. N Engl J Med 2012;366:2038-40. [Crossref] [PubMed]
- Borger DR, Tanabe KK, Fan KC, et al. Frequent mutation of isocitrate dehydrogenase (IDH)1 and IDH2 in cholangiocarcinoma identified through broad-based tumor genotyping. Oncologist 2012;17:72-9. [Crossref] [PubMed]
- Kipp BR, Voss JS, Kerr SE, et al. Isocitrate dehydrogenase 1 and 2 mutations in cholangiocarcinoma. Hum Pathol 2012;43:1552-8. [Crossref] [PubMed]
- Wang P, Dong Q, Zhang C, et al. Mutations in isocitrate dehydrogenase 1 and 2 occur frequently in intrahepatic cholangiocarcinomas and share hypermethylation targets with glioblastomas. Oncogene 2013;32:3091-100. [Crossref] [PubMed]
- Saha SK, Parachoniak CA, Ghanta KS, et al. Mutant IDH inhibits HNF-4alpha to block hepatocyte differentiation and promote biliary cancer. Nature 2014;513:110-4. [Crossref] [PubMed]
- Belov AA, Mohammadi M. Molecular mechanisms of fibroblast growth factor signaling in physiology and pathology. Cold Spring Harb Perspect Biol 2013;5:a015958. [Crossref] [PubMed]
- Borad MJ, Champion MD, Egan JB, et al. Integrated genomic characterization reveals novel, therapeutically relevant drug targets in FGFR and EGFR pathways in sporadic intrahepatic cholangiocarcinoma. PLoS Genet 2014;10:e1004135. [Crossref] [PubMed]
- Ross JS, Wang K, Gay L, et al. New routes to targeted therapy of intrahepatic cholangiocarcinomas revealed by next-generation sequencing. Oncologist 2014;19:235-42. [Crossref] [PubMed]
- Sia D, Losic B, Moeini A, et al. Massive parallel sequencing uncovers actionable FGFR2-PPHLN1 fusion and ARAF mutations in intrahepatic cholangiocarcinoma. Nat Commun 2015;6:6087. [Crossref] [PubMed]
- Arai Y, Totoki Y, Hosoda F, et al. Fibroblast growth factor receptor 2 tyrosine kinase fusions define a unique molecular subtype of cholangiocarcinoma. Hepatology 2014;59:1427-34. [Crossref] [PubMed]
- Wu YM, Su F, Kalyana-Sundaram S, et al. Identification of targetable FGFR gene fusions in diverse cancers. Cancer Discov 2013;3:636-47. [Crossref] [PubMed]
- Graham RP, Barr Fritcher EG, Pestova E, et al. Fibroblast growth factor receptor 2 translocations in intrahepatic cholangiocarcinoma. Hum Pathol 2014;45:1630-8. [Crossref] [PubMed]
- Kouhara H, Hadari YR, Spivak-Kroizman T, et al. A lipid-anchored Grb2-binding protein that links FGF-receptor activation to the Ras/MAPK signaling pathway. Cell 1997;89:693-702. [Crossref] [PubMed]
- Gu TL, Deng X, Huang F, et al. Survey of tyrosine kinase signaling reveals ROS kinase fusions in human cholangiocarcinoma. PLoS One 2011;6:e15640. [Crossref] [PubMed]
- Saborowski A, Saborowski M, Davare MA, et al. Mouse model of intrahepatic cholangiocarcinoma validates FIG-ROS as a potent fusion oncogene and therapeutic target. Proc Natl Acad Sci U S A 2013;110:19513-8. [Crossref] [PubMed]
- Shaw AT, Ou SH, Bang YJ, et al. Crizotinib in ROS1-rearranged non-small-cell lung cancer. N Engl J Med 2014;371:1963-71. [Crossref] [PubMed]
- Zender S, Nickeleit I, Wuestefeld T, et al. A critical role for notch signaling in the formation of cholangiocellular carcinomas. Cancer Cell 2013;23:784-95. [Crossref] [PubMed]
- Yoon HA, Noh MH, Kim BG, et al. Clinicopathological significance of altered Notch signaling in extrahepatic cholangiocarcinoma and gallbladder carcinoma. World J Gastroenterol 2011;17:4023-30. [Crossref] [PubMed]
- Wu WR, Shi XD, Zhang R, et al. Clinicopathological significance of aberrant Notch receptors in intrahepatic cholangiocarcinoma. Int J Clin Exp Pathol 2014;7:3272-9. [PubMed]
- Huntzicker EG, Hotzel K, Choy L, et al. Differential effects of targeting Notch receptors in a mouse model of liver cancer. Hepatology 2015;61:942-52. [Crossref] [PubMed]