Neoadjuvant hyperfractionated-accelerated radiotherapy with concomitant chemotherapy in esophageal cancer: phase II study
Introduction
Despite therapeutic advances, the prognosis of esophageal cancer remains poor. Esophagectomy is the standard treatment option for resectable esophageal cancers, but its efficacy is limited in locally advanced disease. The failure to administer effective loco-regional treatment and early spread of the disease are the main factors associated with poor prognosis, and therefore local control is currently considered a major determinant of survival.
A multidisciplinary approach is necessary for the management of locally advanced esophageal cancer, as reflected by the fact that surgery alone can only provide low cure rates (1,2). Therefore, studies have focused on the neoadjuvant chemotherapy (CT), radiotherapy (RT), and chemoradiotherapy (CRT) combinations in order to increase resectability. Evidence for the efficacy of neoadjuvant monotherapy with chemotherapy or radiotherapy is limited; however, several comparative studies have reported superior results with neoadjuvant chemoradiotherapy (3-5). However, there is still need for studies that evaluate the role of novel chemotherapies or more efficient use of RT.
For instance, concomitant use of chemotherapy and a radiation dose schedule that is more efficient compared to conventional radiotherapy may provide better complete response and survival rates and reduce the tumor stage (3). In this regard, the fundamental principle forming the basis of the accelerated fractionated radiotherapy is to shorten the duration of treatment as well as decreasing the regeneration of tumor cells during treatment in order to improve tumor control and to achieve therapeutic gains in terms of reduced tissue injury in the long term. This approach does not require any increase in the dose applied per fraction and allows for adequate time between the fractions for tissue repair.
This study aimed to assess the efficacy and tolerability of neoadjuvant chemotherapy and hyperfractionated accelerated radiotherapy in patients with esophageal cancer.
Methods and materials
Patient eligibility
An observational study was carried out to evaluate the efficacy and safety of hyperfractionated-accelerated radiotherapy with concomitant chemotherapy followed by surgery in patients admitted with a diagnosis of esophageal cancer between June 2006 and January 2008 in the Department of Radiation Oncology, Istanbul Faculty of Medicine, Istanbul University. A total of 20 eligible patients were included in the study. Patients were considered eligible if they met all of the following criteria: presence of newly diagnosed treatment-naïve esophageal squamous cell cancer (cervical squamous cell cancers and adenocarcinomas of the lower esophagus excluded); disease limited to esophagus and regional lymph nodes; Eastern Cooperative Oncology Group Performance Status (ECOG PS) 0-1; acceptable blood count and biochemistry values (i.e. hemoglobin >10 g/dL, WBC >4,000/mL, PLT >100,000/µL, total bilirubin <2 mg/dL, creatinine <1.3 mg/dL, ALT-AST <80 U/L); and willingness to give written informed consent for the treatment of the study protocol. Exclusion criteria were as follows: presence of distant metastases; known active infection; cardiac failure; myocardial infarction within the last 6 months; inadequately controlled diabetes; severe COPD; pregnancy or lactation; current or past history of substance or alcohol abuse (social drinking was not an exclusion criteria). In addition, patients were excluded if they were deemed uncooperative by the investigator. The study protocol was approved by the local ethics committee and study procedures were conducted in accordance with Declaration of Helsinki of 1975, as revised in 2000. All patients gave informed consent prior to study entry. Figure 1 shows study diagram.
Patient assessment before neoadjuvant radiochemotherapy
All diagnoses were based on the result of endoscopic biopsy, and after the initial evaluation of medical history, ECOG performance status, and physical examination, the following assessments were performed: histopathological consultation; complete blood count; blood biochemistry; computerized tomography of the thorax and abdomen and/or positron emission tomography (PET-CT). Planning was done using PET CT in 16 patients and computerized tomography in four patients. Endoscopic ultrasound (EUS) was not performed for staging or evaluation of response. Patients had pulmonary function tests before the treatment. Eligible patients were evaluated and staged by a medical team whose members consisted of a surgeon, a radiation oncologist, and a medical oncologist. Before the study, a chemotherapy port was placed and supplemental enteral nutritional support was initiated.
Chemotherapy and radiotherapy regimen
After the preperatory stage of the study was completed, neoadjuvant cisplatin and 5-FU were given with 28 day intervals in a total of three courses with the following administration schedule: 60 mg/m2 cisplatin infusion on day 1, and 600 mg/m2 5-FU infusion (120 hours) on days 1-5.
Along with the third course of chemoterapy, hyperfractionated accelerated radiotherapy (HART) was given with the following dose schedule: 5760 cGy/36 fr/16 day. Patients received 3 fractions per day with at least 6-hour intervals between the fractions (07:00-08:00, 13:00-14:00 and 19:00-20:00) for 5 days a week. The treatment was completed in a total of 16 days including weekends. During the entire treatment period patients received a daily dose of 480 cGy/3fr (150-150-180 cGy). A 3D conformal RT was done. In Phase I, AP/PA field and in phase II three (posterior/two oblique) fields were used. In some patients three-field approach was used in both phases.
Normal tissues constraints included 3840 cGy to the spinal cord, lung V20 doses less than 27%, heart V60 doses less than 30% and a maximum of 4000 cGy to the whole heart.
Using computerized tomography and/or PET-CT images, the target volumes and high-risk organs were determined according to the prechemotherapy volumes and the volumes were checked by a radiation oncologist and radiologist in each patient. GTV (Gross Tumor Volume) was the tumor volume seen in PET-CT or computerized tomography. During phase I CTV was GTV plus 3 cm and PTV1 was CTV plus 2 cm. For phase II, PTV2 was GTV plus 3 cm. In PTV planning, a 1 cm margin was allowed for GTV in postero-anterior and two lateral directions. Uninvolved regional lymph nodes were not included to treatment volumes. Lymph nodes ≥12 mm on computerized tomography or with FDG pathological uptake on PET (regardless of size) was interpreted as metastatic. A radiation dose higher than conventional RT scheme (5040 cGy/28 fr) was planned based on biological equivalent dose (BED) values and a dose close to CHART scheme was aimed. Dose planning was as follows: BED3, 10580 cGy; BED10, 6430 cGy.
Assessment of response and toxicity
Toxicity was assessed at Days 5, 10, 12 and 16 during treatment, and at each follow-up visit after the treatment. Reactions occurring within the first month after completion of CRT were considered as “early reactions”, while those occurring between months 1 and 6 and after month 6 were designated as subacute and late reactions, respectively. Toxicity of chemotherapy and acute radiotherapy were assessed using “Common Terminology Criteria for Adverse Events” (NCI CTC v3). Late effects of radiotherapy were assessed using Radiotherapy Oncology Group/European Oncology Research and Treatment Center (RTOG/EORTC) late radiation morbidity scores. In both scoring systems, the grading is between 0 and 5 where 0 denotes “no change” and 5 denotes “death”. Evaluation of resectability and decision of operability were evaluated radiologically according to WHO criteria 4 weeks after the completion of CRT using thorax computerized tomography and/or PET-CT findings.
Following the treatment patients attended follow-up visits monthly with complete blood count, blood biochemistry, and PA chest X-rays. The decision for surgery was based on the assessment by a multidisciplinary team according to partial/complete radiological response. The selection criterion for operation was resectability. Five patients refused surgery although they had been evaluated as candidates for operation; seven patients underwent total radical esophagectomy with three-field lymphadenectomy (celiac, mediastinal, cervical) and 8 patients did not receive operation on clinical grounds since they had complete radiological response. All surgeries were planned surgery and were performed within 6 to 8 weeks after neoadjuvant therapy. In addition to imaging studies required for surgical evaluation, a thorax computerized tomography was performed 6 month after the treatment. Local control was assessed radiologically in non-surgical patients using the computerized tomography images at 6 month. In surgical patients, local control was evaluated using pathology findings. The overall survival was defined as the time from diagnosis to last follow-up visit or death.
Statistical methods
Data analysis was performed using SPSS (Statistical Package for Social Sciences) version 16.0. The primary endpoint of our study included acute-late toxicity and treatment response. Secondary endpoints were local control and overall survival. Categorical data have been presented as number and percent of patients, and overall survival was determined using Kaplan-Meier method.
Results
Clinical characteristics of study subjects are presented in Table 1. All patients had a histologically proven squamous cell carcinoma of the esophagus. Of the 20 patients, 19 (95%) completed 2 courses of chemotherapy and 1 course of radiochemotherapy, and the remaining one patient did not receive chemotherapy during the last course due to low tolerance. In two patients a 20% dose reduction in chemotherapy was required.
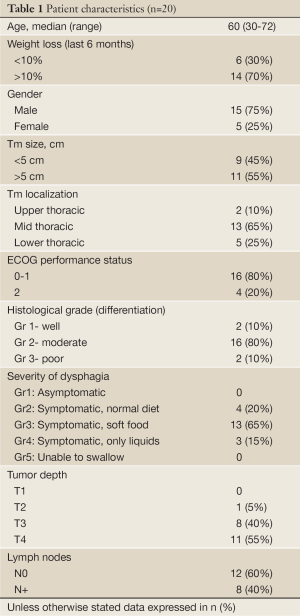
Full Table
All patients could receive the planned RT dose of 5760 cGy. The examination of target volumes in conformal planning showed a median tumor volume of 43.9 cc (13.1-147.8 cc; mean 59.9 cc), PTV1 volume of 310.3 cc (136.9-651.2 cc), and PTV2 volume of 265.5 cc (94.8-548.7 cc). The median dose applied to the 95% of the planned target volume was 5760 cGy (5459-6243 cGy). The maximum median dose that was received by the tumor tissue (GTV) was 6089 cGy (5911-7372 cGy). The minimum median dose was 5712 cGy (5510-6723 cGy). Median dose of the whole heart and 30% of heart were 308 cGy (10-1222 cGy) cGy and 4287 cGy (1820-5656 cGy) cGy. Average median lung dose was 1485 cGy (615-2217 cGy), while the maximum dose on the spinal cord was 4110 cGy. Median lung volumes exposed to 1000 and 1500 cGy were 41.5% (12.2-54%) and 30.8% (8.1-43.9%), respectively.
Acute toxicity
Acute toxicity associated with chemotherapy and radiotherapy is shown in Table 2. Odynophagia was the most frequent grade III toxicity (50%) which usually emerged in the 2nd week of chemoradiotherapy, worsened during the 3rd week, and gradually disappeared after the 5th and 6th weeks. Only one patient had low hemoglobin value (grade II) which resolved spontaneously within 2 weeks after CRT. No Grade IV or higher toxicity was observed. Acute toxicity reactions were generally acceptable and did not require any treatment discontinuation or interruption.
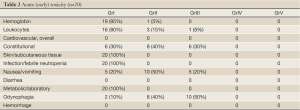
Full Table
Subacute and late toxicity
Subacute and late effects of radiotherapy are shown in Table 3. Grade III or higher toxicity occurred in 15 patients (75%). Of the study subjects 9 (45%) had ≥ Grade III esophageal [upper gastrointestinal system (GIS)] reactions: 5 (20%) had esophageal perforation and bleeding, and 4 died due to severe gastrointestinal bleeding during the subacute stage (1.5-5 months). The maximum dose of radiotherapy in patients with ≥ Grade III esophageal toxicity ranged between 5911 and 6153 cGy. Nine patients (45%) had Grade II lung toxicity that was not associated with severe symptoms and that was readily controlled with steroids and antibiotics. In terms of cardiac effects, only one patient had pericardial effusion approximately 1.5 months after the treatment. Due to worsening respiratory status, the patient required pericardiectomy for the treatment of cardiac tamponade. In this patient the maximum point dose on the heart, the average cardiac dose, and the dose received by the entire cardiac volume were 6090 cGy, 3535 cGy and 380 cGy, respectively. No patients had L’Hermitte’s syndrome or myelitis.

Full Table
Efficacy of neoadjuvant radiochemotherapy
Thorax CT and/or PET-CT scan were used to determine tumor response. To avoid a possible damage to fragile esophageal tissue, esophagogastroduodenoscopy (EGD) was not used to confirm pathologic complete response (pCR) after chemoradiotherapy. Radiologically, 8 patients (40%) had complete response, 8 (40%) had partial response, and 3 (15%) had stable disease, with only 1 patient (5%) with progressive disease. Seven patients underwent surgery and had R0 resection, and in 6 (85%), pathological complete response was demonstrated. In 13 patients without surgery, 2 (15%) had radiological complete response at 6-month follow-up examination. Overall, 8 patients (40%) had local control.
The median duration of follow-up was 13 months (range: 4-64 months). Figure 2 shows survival function. Eleven patients (55%) died, 9 patients (45%) are alive. The 5 years overall survival rate was 38.1%.
Discussion
In patients with esophageal cancer, radiation dose and dose-intensity of radiotherapy can be increased using hyperfractionated accelerated RT, without prolonging the duration of neoadjuvant chemoradiotherapy following two courses of induction chemotherapy. Table 4 shows previous neoadjuvant radiotherapy plus concurrent chemotherapy studies. Our results indicate that in this patient group, it is possible to achieve encouraging local control and survival rates with appropriate chemotherapy and hyperfractionated accelerated radiotherapy. Accelerated fractions previously used generally include those dose schedules that consisted of hyperfractionated accelerated RT (HART), continuous hyperfractionated accelerated RT (CHART), concomitant boost RT, and late course accelerated fractionated RT (LCAF). In 1993, Kikuchi (6) reported his results with HART for the treatment of esophageal cancer with 6200-6400 cGy in 40 fractions that were given in 4.5 weeks. The 5-year local control and survival rates were superior compared to the control group (57.2% vs. 31.5%, P˂0.05). Powel et al. (7) used short-term intensive accelerated fractions in 24 subjects, followed by 3 daily fractions (150 cGy per fraction) given with 6-hour intervals for a total of 12 days to achieve a total dose of 5400 cGy. On average, dysphagia emerged after 7.8 months (0-41.4 months) in the CHART group, as compared to 5.5 months (0-48 months) in the controls. The mean duration of survival was 12 months (0.5-112 months) in the CHART group and 15 months (3.6-56 months) among the controls. The 3-year cause-specific survival rates were 40%, 22%, and 6% in T1, T2, and T3 patients, respectively.
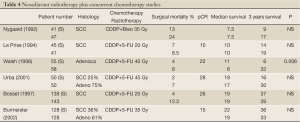
Full Table
Table 5 shows a comparison of several Phase II and III studies in terms of the radiation dose applied, biological effective doses, chemotherapy schedules according to pathological complete response (pCR), operational mortality, median survival, and 3-year survival rates (8-17). Despite significant differences in patient cohorts and tumor- and patient-related parameters, the studies were examined with regard to BED and pCR ratios. If pCR is regarded as a meaningful marker of radiation dose after neoadjuvant CRT, then the pCR ratio increases along with the increase in the corrected-dose based on BED calculation (α/β ratio is 10, and α is 0.3 for the time-dependent tumor proliferation). Examination of the RT in these studies reveals a pCR ratio of 10% with 1850 cGy (2000 cGy/10 fr/2wk) of BED, while the corresponding figure is 26% with a BED of 3900 cGy (4400 cGy/22 fr/4.4 wk) and 28% with a BED of 4600 cGy (4500 cGy/30 fr/3 wk).
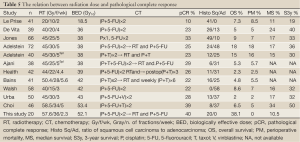
Full Table
In the study by Choi et al. (17), pCR was 39% with a BED of 5340 cGy (5850 cGy/34 fr/5 wk). In this study, the BED in the HART schedule applied was 5210.4 cGy (5760 cGy/36 fr/ 16 days). Only 7 out of 20 patients could be surgically treated, and pathological complete response was observed in 6. Also in two patients who are still alive without surgery, the tumor radiologically disappeared and no pathological sign of tumor was found in control biopsies, yielding a total local control rate of 40% for our study.
In this study, CDDP and 5-FU were administered in doses that were below the standard, which may account for the low rates of hematologic toxicity in our patients. Absence of skin and subcutaneous reactions > Grade I could be explained by the use of 15 MV linear accelerator (LINAC) device and by the reduced skin dose due to multiple-area planning in the treatment. Twenty-five percent of our patients had Grade III nausea/vomiting and 50% had Grade III odynophagia, which occurred early in the course of chemoradiotherapy and resolved thereafter. In general, acute reactions were acceptable and allowed completion of the planned treatment without interruption.
Overall, proportion of patients with Grade III or higher toxicity during subacute and late phases was 75%, with 4% having Grade V toxicity. Grade I and II lung toxicity occurred in 55% and 45% of the patients, respectively, with no patients experiencing lung toxicity higher than Grade III. In two-dimensional treatments, Radiation pneumonitis is the most feared complication and lung is the dose-limiting organ. In this regard, Lee et al. (18) reported on the association between lower lung volume doses during concomitant chemoradiotherapy in esophageal cancer and postoperative pulmonary complications and observed lung toxicity in 11 of 61 patients (18%), 2 dying due to worsening pneumonia. In that study significant differences were observed if the percentage of lung volume receiving 1000 cGy is higher than 40% as compared to less than 40% (35% vs. 8%, P=0.014) and if the percentage of lung volume receiving 1500 cGy is higher than 30% as compared to less than 30% (33% vs. 10%, P=0.036). However, patients who received 2000 cGy in less or more than 20% of their lung volumes did not differ significantly with regard to toxicity (32% vs. 10%, P=0.079). These data suggest that life-threatening pulmonary complications can be avoided by decreasing the radiation received by the lungs using 3-dimensional conformal RT and by reducing RT doses (1000-1500 cGy). Although in this study an effort was made to limit V20 doses below 27%, this was not possible in 9 patients (45%) due to tumor location and dimension (>5 cm). Of the 9 patients with a V20 greater than 27%, 5 are alive and disease-free, and 8 received three-field radiotherapy in a single phase or received three-field radiotherapy in both phases.
In radiobiological studies, when the average duration of time between fractions was less than 4.5 hours in hyperfractionated protocols, increased incidence of late complications was reported (19). According to clinical observations, 6 hours suffice for normal tissues with the exception of spinal cord, for which a dose below 4000 cGy is recommended in hyperfractionated accelerated RT. The maximum point dose received by the spinal cord in our study was 4110 cGy, with 10 patients receiving doses above 4000 cGy. Of these 10 patients, 5 died, and the median duration of follow up in the remaining 5 patients is 15 months (9-23 months). No patients in the study had L’Hermitte’s syndrome or myelitis.
No cardiac toxicity occurred in 19 of our study subjects (95%). In only one patient (5%), pericardial effusion developed approximately 1.5 months after the treatment. DVH examination showed that the radiation dose received by the entire cardiac volume was 308 cGy. In the study by Ishikura et al. (20), 78 patients with esophageal cancer received concomitant CRT (6000 cGy plus brachytherapy) and 8 patients (10%) had Grade III pericarditis, 3 patients (4%) had radiation pneumonia, and 4 patients (5%) had esophageal strictures. In the study by Yamada et al. (21) where concomitant CRT (5500-6600 cGy with brachytherapy) was given to 63 patients with T1 N0 esophageal cancer, late toxicities included pericardial effusion in 3 cases, and esophageal fistula (Grade IV and V) in 2 cases. Three-dimensional conformal RT, intensity-adjusted RT and proton treatment as well as avoidance from pre-load areas are recommended to avoid from cardiac side effects.
In this study, four patients died due to gastrointestinal bleeding, which was probably due to esophageal perforation resulting from tumor necrosis. An additional five patients had grade III esophageal toxicity. A higher than expected rate of esophageal toxicity observed in this study may be due to the high radiation dose used. In addition, hyperfractionated dosing may not allow appropriate tissue repairing.
In one patient, PET-CT showed metabolic complete response, but the patient died at week 5 before undergoing surgery. Early thorax computerized tomography images following chemoradiotherapy did not allow an accurate distinction between edematous and tumor tissues due to acute side effects. We believe that if surgery can be accomplished in patients with clinical response, it may be possible to minimize deaths due to esophageal perforation.
Conclusions
Improved radiation dose schedules and achievement of maximum possible pCR rates may improve survival and organ protection in patients with esophageal cancer. In these patients, HART may help to target local disease control and increased survival. However, several factors including the performance status, treatment compliance, and tumor dimensions also play an important role in patient selection. Further studies to improve neoadjuvant and radical chemoradiotherapy dose schedules are warranted for maximum local control rates with minimal toxicity. In particular, high esophageal toxicity should be addressed.
Acknowledgements
Disclosure: The authors declare no conflict of interest.
References
- Kasai M, Mori S, Watanabe T. Follow-up results after resection of thoracic esophageal carcinoma. World J Surg 1978;2:543-51. [PubMed]
- Earlam R, Cunha-Melo JR. Oesophageal squamous cell carcinoma: I. A critical review of surgery. Br J Surg 1980;67:381-90. [PubMed]
- Urschel JD, Vasan H. A meta-analysis of randomized controlled trials that compared neoadjuvant chemoradiation and surgery to surgery alone for resectable esophageal cancer. Am J Surg 2003;185:538-43. [PubMed]
- Fiorica F, Di Bona D, Schepis F, et al. Preoperative chemoradiotherapy for oesophageal cancer: a systematic review and meta-analysis. Gut 2004;53:925-30. [PubMed]
- Greer SE, Goodney PP, Sutton JE, et al. Neoadjuvant chemoradiotherapy for esophageal carcinoma: a meta-analysis. Surgery 2005;137:172-7. [PubMed]
- Kikuchi Y. Study on clinical application of multiple fractions per day radiation therapy with concomitant boost technique for esophageal cancer. Hokkaido Igaku Zasshi 1993;68:537-56. [PubMed]
- Powell ME, Hoskin PJ, Saunders MI, et al. Continuous hyperfractionated accelerated radiotherapy (CHART) in localized cancer of the esophagus. Int J Radiat Oncol Biol Phys 1997;38:133-6. [PubMed]
- Le Prise E, Etienne PL, Meunier B, et al. A randomized study of chemotherapy, radiation therapy, and surgery versus surgery for localized squamous cell carcinoma of the esophagus. Cancer 1994;73:1779-84. [PubMed]
- De Vita F, Di Martino N, Orditura M, et al. Preoperative chemoradiotherapy for squamous cell carcinoma and adenocarcinoma of the esophagus: a phase II study. Chest 2002;122:1302-8. [PubMed]
- Jones DR, Detterbeck FC, Egan TM, et al. Induction chemoradiotherapy followed by esophagectomy in patients with carcinoma of the esophagus. Ann Thorac Surg 1997;64:185-91; discussion 191-2. [PubMed]
- Adelstein DJ, Rice TW, Becker M, et al. Use of concurrent chemotherapy, accelerated fractionation radiation, and surgery for patients with esophageal carcinoma. Cancer 1997;80:1011-20. [PubMed]
- Adelstein DJ, Rice TW, Rybicki LA, et al. Does paclitaxel improve the chemoradiotherapy of locoregionally advanced esophageal cancer? A nonrandomized comparison with fluorouracil-based therapy. J Clin Oncol 2000;18:2032-9. [PubMed]
- Ajani JA, Komaki R, Putnam JB, et al. A three-step strategy of induction chemotherapy then chemoradiation followed by surgery in patients with potentially resectable carcinoma of the esophagus or gastroesophageal junction. Cancer 2001;92:279-86. [PubMed]
- Bains MS, Stojadinovic A, Minsky B, et al. A phase II trial of preoperative combined-modality therapy for localized esophageal carcinoma: initial results. J Thorac Cardiovasc Surg 2002;124:270-7. [PubMed]
- Walsh TN, Noonan N, Hollywood D, et al. A comparison of multimodal therapy and surgery for esophageal adenocarcinoma. N Engl J Med 1996;335:462-7. [PubMed]
- Urba SG, Orringer MB, Turrisi A, et al. Randomized trial of preoperative chemoradiation versus surgery alone in patients with locoregional esophageal carcinoma. J Clin Oncol 2001;19:305-13. [PubMed]
- Choi N, Park SD, Lynch T, et al. Twice-daily radiotherapy as concurrent boost technique during two chemotherapy cycles in neoadjuvant chemoradiotherapy for resectable esophageal carcinoma: mature results of phase II study. Int J Radiat Oncol Biol Phys 2004;60:111-22. [PubMed]
- Lee HK, Vaporciyan AA, Cox JD, et al. Postoperative pulmonary complications after preoperative chemoradiation for esophageal carcinoma: correlation with pulmonary dose-volume histogram parameters. Int J Radiat Oncol Biol Phys 2003;57:1317-22. [PubMed]
- Ang KK, Thames HD, Peters LJ. Altered fractionation schedules. In: Perez CA, Brady LW. eds. Principles and practice of radiation oncology. 3rd ed. Philadelphia: Lippincott-Raven, 1998:119-42.
- Ishikura S, Nihei K, Ohtsu A, et al. Long-term toxicity after definitive chemoradiotherapy for squamous cell carcinoma of the thoracic esophagus. J Clin Oncol 2003;21:2697-702. [PubMed]
- Yamada K, Murakami M, Okamoto Y, et al. Treatment results of chemoradiotherapy for clinical stage I (T1N0M0) esophageal carcinoma. Int J Radiat Oncol Biol Phys 2006;64:1106-11. [PubMed]