Promising therapeutics of gastrointestinal cancers in clinical trials
Introduction
Over the past few decades, the treatment paradigm of gastrointestinal (GI) cancers has changed substantially. Monoclonal antibodies targeting angiogenesis and the epidermal growth factor receptor (EGFR) signaling pathway have become standard treatments for advanced colorectal cancers (CRCs) (1,2). Small molecule multikinase inhibitors have also demonstrated survival benefit in patients with refractory CRCs and hepatocellular carcinomas (HCCs) (3,4). Most recently, immunotherapy has shown promising clinical efficacy in multiple types of GI cancers (5,6). Although chemotherapy remains the mainstay of treatments for the majority of GI cancers, novel agents either as single agents or in combination are being evaluated in clinical trials at an accelerated pace. Here we review the major drugs that have demonstrated promising therapeutic activity in clinical trials. Immunotherapy is not a significant part of the discussion here as it is discussed in great detail in another review in this issue.
Growth signaling pathway inhibitors
Inhibition of growth signaling pathways has traditionally been a research hot spot for GI cancers, and it has achieved significant success with the development and clinical use of small molecule kinase inhibitors and monoclonal antibodies (1,2,4). Strategies of inhibiting the growth factor pathways include targeting cell surface receptors or their downstream cellular cascades. Vascular endothelial growth factor receptor (VEGFR), MET, c-kit, platelet-derived growth factor receptor (PDGFR), and fibroblast growth factor receptor (FGFR) are commonly targeted cell surface receptors. Activation of these receptors stimulates various downstream signaling pathways that promote tumor cell proliferation and sustain cell survival. One of the pathways commonly involved is the Raf-MEK-ERK mitogen-activated protein kinase (MAPK) cascade, in which activated Raf kinases phosphorylate the downstream MEK kinases, and the latter activate the extracellular signal-regulated kinases (ERK), which lead to cell growth (Figure 1) (7). Therapeutics targeting the above cell surface receptors and the downstream Raf-MEK-ERK pathway have shown some promising results in GI cancers in early phase clinical studies.
Cabozantinib is a dual inhibitor of the cell surface growth receptors VEGFR2 and MET. In a phase II study, patients with advanced HCCs were treated with cabozantinib at 100 mg daily for 12 weeks as the lead-in stage (8). Patients who demonstrated partial response (PR) were continued on the treatment, whilst those with stable disease (SD) were randomized to continue receiving cabozantinib or placebo. Among the 41 patients enrolled, half had received prior sorafenib. Cabozantinib was frequently associated with fatigue, diarrhea, hand-foot syndrome, nausea/vomiting, and thrombocytopenia. Sixty percent of patients needed dose reductions during the lead-in stage. The overall response rate (ORR) was 5%, but 78% of patients had SD. The median progression-free survival (PFS) was 4.4 months, and the median overall survival (OS) was 15.1 months. These results were encouraging given that historically, the ORR and the median OS for untreated HCC patients who received sorafenib were only 2% and 10.7 months, respectively (4). A phase III study evaluating cabozantinib at a dose of 60 mg daily versus placebo in patients with advanced HCC who have received prior sorafenib is ongoing (NCT01908426).
Another multikinase inhibitor, regorafenib, targets multiple cell surface growth signaling receptors including VEGFR1-3, KIT, PDGFR, and FGFR. It has been approved for the treatment of advanced CRC. Recent data indicated that it is also effective in treating advanced HCC. The phase III RESORCE study tested the efficacy of regorafenib in patients with HCC who had progressed on sorafenib. The results were presented at the European Society for Medical Oncology (ESMO) World Congress of Gastrointestinal Cancer 2016 (9). A total of 573 patients were randomized 2:1 to receive regorafenib at 160 mg for 3 weeks every 4-week cycle versus placebo. Severe adverse events were experienced in 79.7% of patients treated with regorafenib versus 58.5% of those received placebo. Common adverse events of regorafenib included hypertension, hand-foot syndrome, fatigue, and diarrhea. Regorafenib significantly improved the ORR (10.6% vs. 4.1%; P=0005), PFS (3.1 vs. 1.5 months; HR 0.46; 95% CI: 0.37–0.56; P<0.001), and OS (10.6 vs. 7.8 months; HR 0.62; 95% CI: 0.50–0.78; P<0.001) compared to placebo. These results are very encouraging and make regorafenib an attractive agent for HCC patients progressed on sorafenib.
Similar to regorafenib, nintedanib is also a multikinase inhibitor targeting VEGFR1–3, FGFR, and PDGFR, but with a better toxicity profile. In a phase I study, 30 patients with previously treated advanced CRC were treated with nintedanib (10). This was a heavily pretreated population with prior lines of treatment ranging from 1–5. Nintedanib was well tolerated with the most common adverse events being mild nausea, vomiting, and diarrhea. Only 10% of patients had ≥ grade 3 toxicities. Twenty-four patients (80%) had SD for ≥8 weeks, and one patient had PR. Compared to regorafenib, which provided a SD rate of 40% and a PR rate of 1% but caused ≥ grade 3 toxicities in more than half of patients (3), nintedanib was much better tolerated and seemed to be quite active in refractory CRCs. The phase III LUME-Colon 1 trial is comparing nintedanib with placebo in patients with previously treated metastatic CRC (NCT02149108). This study has finished accrual of 764 patients and was estimated to be completed in December 2016.
There has been quite a lot of excitement in targeting FGFR, particularly in cholangiocarcinoma. The FGFR family consists of four receptors that are activated by binding fibroblast growth factors using heparin or heparin sulfate proteoglycan as a co-factor (11). Activated FGFR triggers complex downstream pathways including the phosphoinositide 3-kinase (PI3K)-AKT cascades and the Raf-MEK-ERK signaling pathway. FGFR2 fusions have been identified in approximately 13% of intrahepatic cholangiocarcinomas (12,13). Preliminary efficacy of FGFR inhibitors has been demonstrated in patients with FGFR2 fusion and preclinical models (14,15). A phase II study examined the activity of a pan-FGFR inhibitor, BGJ398, in patients with previously treated advanced cholangiocarcinoma harboring FGFR2 alterations (16). Forty-seven patients received the study drug. FGFR2 translocations were found in 38 patients, with FGFR2-BICC1 fusions being the most common (n=9). Nine patients had other FGFR alterations, including mutations in FGFR and amplifications in FGFR2 and FGFR3. This was a heavily pretreated patient population, with 62% of patients receiving ≥2 prior lines of therapies, and 11% with ≥4 prior regimens. Adverse events were overall manageable, with hyperphosphatemia, fatigue, constipation, and diarrhea being the most common. Among a total of 36 evaluable patients, eight patients (22%) had PR and 19 (53%) had SD. Median duration of drug exposure was 188 days. These results were encouraging when compared to the median PFS of approximately 3 months in patients who received second-line therapies for advanced cholangiocarcinoma (17,18). Several clinical studies are ongoing to test the role of other FGFR inhibitors in cholangiocarcinomas or other solid tumors harboring FGFR alterations (NCT02834780, NCT01752920).
Moving from the cell surface receptors to downstream signaling cascades, the Raf-MEK-ERK signaling pathway is frequently involved in carcinogenesis. The BRAF gene encodes an intracellular Raf protein, which upon activation, phosphorylates and triggers sequential activation of the MEK/ERK protein cascade. BRAF mutations occurred in approximately 12% of CRCs (19). Patients with metastatic BRAF-mutant CRCs have a worse survival compared to their BRAF wild type counterparts, with a median survival of approximately one year (20). In contrast to the potent activity of the BRAF inhibitor vemurafenib in melanoma, single-agent vemurafenib failed in a phase II study of patients with metastatic BRAF-mutated CRCs, with an ORR of 5% and a median PFS of 2.1 months (21). This indicated that the BRAF inhibition alone might not be sufficient in treating BRAF-mutant CRCs, and combination therapy might be a better treatment approach. In a phase II study, investigators examined the combination of a BRAF inhibitor, encorafenib, and the anti-EGFR antibody, cetuximab, with or without the PI3Kα inhibitor, alpelisib, in patients with previously treated advanced CRCs that are BRAF-mutated (22). A total of 102 patients were randomized to the triplet versus the doublet arm. Grade 3–4 adverse events including anemia, hyperglycemia, and elevated lipase were more common in the triplet arm. The ORR was 27% for the triplet arm versus 22% for the doublet arm. Both median PFS (5.4 months for triplet versus 4.2 months for doublet; HR 0.8; 95% CI: 0.5–1.2; P=0.14) and median OS were similar between the two arms (13.1 months for triplet versus 12.4 months for doublet; HR 1.1; 95% CI: 0.6–2.0). The median OS in this study was much better compared to the historical OS of approximately 6 months for this patient population (23,24), but the survival benefit needs to be confirmed in phase III clinical trials. Furthermore, whether the survival benefit with additional alpelisib outweighs the increased toxicities awaits further examination in future studies.
Further down the Raf-MEK-ERK pathway, small molecule kinase inhibitors targeting the MEK protein, such as cobimetinib and trametinib, have prolonged survival in patients with BRAF-mutant melanoma (25,26). Ongoing efforts are examining the role of MEK inhibitors in CRC. A phase Ib study tested the combination of cobimetinib with the PD-L1 inhibitor atezolizumab in patients with microsatellite stable CRCs. Single agent PD-1 blockade does not appear to have significant anti-tumor activity in microsatellite stable tumors (5). Inhibiting MEK with cobimetinib upregulates the major histocompatibility complex (MHC) class I on tumor cells, enhances intratumoral T cell filtration, and increases the activity of atezolizumab (27). In this study, a total of 23 patients received cobimetinib and atezolizumab (28). This was a heavily pretreated patient population, with a median of three lines of prior therapies. The microsatellite status was stable in 30% of patients and unknown in the other 70%. There were no dose-limiting toxicities (DLTs) observed with the maximum dose of cobimetinib at 60 mg daily. The most common adverse events included diarrhea, fatigue, rash, pruritus, and nausea. One third of patients experienced grade 3 toxicities, with diarrhea being the most common. But there were no grade 4 or 5 toxicities. The ORR was 17%, and the duration of response was not reached (range, 5.4 to 11.1+ months) by the time results were presented. The 6-month PFS and OS rates were 35% and 72%, respectively. Again, compared to the historical ORR of 1% and median PFS of approximately 2 months in patients with refractory CRCs, these results looked extremely promising (3). Based on these data, a phase III study investigating the activity of cobimetinib plus atezolizumab in patients with chemotherapy-refractory advanced CRCs is ongoing (NCT02788279).
Targeting the tumor microenvironment
The tumor microenvironment is a complex network comprised of extracellular matrix (ECM) and a myriad of immune cells. It serves as a mechanical and biochemical barrier that shields off the delivery and/or attenuates the antitumor effect of drugs, and more importantly, creates an immunosuppressive environment that impedes tumor immune response. Drugs targeting the tumor microenvironment have been aimed at different facets of the network, including the pro-tumorigenic cytokine signaling pathways such as transforming growth factor-β (TGF-β) and CC chemokine ligand 2-CC chemokine receptor 2 (CCL2-CCR2), enzymes or transmembrane proteins that modulate T cell activities such as indoleamine-2,3-dioxygenase (IDO) and CD40, and the mechanical component of the tumor stroma such as hyaluronan (HA).
The TGF-β cytokine is secreted by tumors and, in turn, facilitates tumor progression and invasion. TGF-β upregulates VEGF and promotes angiogenesis, suppresses the antitumor effect of T cells and natural killer (NK) cells, and stimulates the production of metalloproteinases (MMPs) that degrade ECM and facilitate tumor invasion (29). The TGF-β receptor-1 kinase inhibitor galunisertib was tested in a phase II study in patients with advanced HCC who had progressed on or were ineligible for sorafenib (30). A total of 109 HCC patients with Child Pugh A or B7 disease were randomized to receive galunisertib at 160 or 300 mg/day on a 2-week on, 2-week off schedule. Twenty-four percent of patients had a reduction in serum alpha fetoprotein (AFP) levels by >20% from baseline. The median time-to-progression (TTP) and OS were 2.8 and 8.3 months in the overall population, and 4.3 and 21.4 months in the AFP responders, respectively. Galunisertib was well tolerated at both dose levels. Neutropenia, fatigue, and anemia were the most common grade 3–4 adverse events. The 300 mg/day dose was found to confer better drug exposure, and it was selected for future studies. Another phase II study tested galunisertib in patients with advanced HCC but low serum AFP levels (31). The majority of patients had received prior sorafenib. Twenty-nine out of 40 patients treated with galunisertib had a reduction in serum TGF-β levels by >20%. In patients with a TGF-β reduction >20%, the median OS was 21.8 months, compared to 7.9 months in those without a TGF-β response. Combinations of galunisertib and immunotherapy are being evaluated in patients with HCC (NCT02423343) and pancreatic cancer (NCT02734160). The incorporation of galunisertib into neoadjuvant chemoradiotherapy is also being explored in locally advanced rectal cancer in a phase II study (NCT02688712).
The CCL2-CCR2 chemokine signaling pathway has been avidly studied as a target for anticancer therapies. Tumors and stromal cells synthesize the CCL2 ligand, which recruits CCR2-positive inflammatory monocytes to the tumors, where they can differentiate into immunosuppressive tumor-associated macrophages (TAMs) (32,33). TAMs play an important role in mediating tumor immune evasion and promoting tumor progression and invasion (34,35). High CCL2 expression and low CD8+ T cell infiltrates in the tumors was associated with decreased survival in patients who underwent resection of pancreatic cancers (36). A phase Ib study tested the combination of the CCR2 inhibitor PF-04136309 with FOLFIRINOX in patients with borderline resectable or locally advanced pancreatic cancers. This combination regimen resulted in an ORR of 49% and a disease control rate of 97% (37). PF-04136309 was generally well tolerated. These results looked promising in light of the ORR of 32% and the disease control rate of 70% with FOLFIRINOX alone (38). A phase I study testing the combination of PF-04136309 with gemcitabine and nab-paclitaxel in pancreatic cancer is ongoing (NCT02732938). Another CCR2 inhibitor, CCX872-B, is being tested in a phase Ib study in combination with FOLFIRINOX in patients with advanced pancreatic cancers (NCT02345408).
Apart from the chemokine signaling pathways, T cells are also major components of the tumor microenvironment and are critical in tumor-specific immune responses. Most tumors evade this immune surveillance by a plethora of mechanisms, one of which being the IDO pathway. IDO is the key enzyme in the catabolism of tryptophan that is critical in the differentiation and proliferation of T cells. Both tumors and antigen-presenting cells (APCs) express IDO, which suppresses tumor-specific cytotoxic T cells and enhances the activity of immunosuppressive regulatory T cells (39). In a breast cancer mouse model, an IDO inhibitor in combination with paclitaxel synergistically induced tumor regression (40). A phase I/II study examined the combination of an IDO inhibitor, indoximod, with gemcitabine and nab-paclitaxel in patients with metastatic pancreatic cancer. In this study, five out of 12 patients achieved an objective response (42%), including one patient with a complete response (41). The combination was well tolerated, with common side effects being fatigue, weight loss, diarrhea, and peripheral edema. An interim analysis of the phase II part of this study continued to confirm the efficacy, with 11 out of 30 patients demonstrating an objective response (37%) (42). Given that the ORR with gemcitabine plus nab-paclitaxel was 23% (43), incorporating indoximod into this regimen may provide additional clinical benefits. This study remains ongoing to further examine the role of indoximod in the treatment of pancreatic cancers (NCT02077881).
Another means to activate cytotoxic T cells is via the ligation of the transmembrane protein CD40. CD40 is a member of the tumor necrosis factor (TNF) superfamily. It is widely expressed on APCs and a variety of tumors (44,45). Ligation of CD40 on APCs orchestrates the priming and activation of tumor-specific cytotoxic T cells and eradicates tumors (46,47). CD40 ligation on carcinoma cells also upregulates the expression of Fas and TNF in these cells and induces apoptosis (48). A phase I study examined the combination of an agonist CD40 monoclonal antibody, CP-870,893, with gemcitabine in patients with untreated advanced pancreatic cancers (49). Four out of 22 patients achieved a PR (18%). The combination was generally well-tolerated, with mild cytokine release syndrome, fatigue, and cytopenia being the most common adverse events. Phase I studies are ongoing to evaluate several CD40 agonistic antibodies either alone or in combination with immunotherapy in patients with solid tumors. Other CD40 antibodies under investigation include APX005M (NCT02482168), SEA-CD40 (NCT02376699), and RO7009789 (NCT02665416, NCT02304393).
Besides the signaling pathways that modify immune responses, the tumor stroma itself can also be a therapeutic target. This is particularly important in pancreatic cancer, which is surrounded by a desmoplastic stroma enriched with HA. There was some preclinical evidence suggesting that HA stimulated pancreatic cancer cell migration (50). High expressions of HA in the tumors were associated with shorter survival in patients with resected pancreatic cancer (51). A PEGylated recombinant human hyaluronidase, PEGPH20, depletes HA and was tested in a randomized phase II trial (52). In this study, patients with untreated metastatic pancreatic cancers were randomly assigned to receive nab-paclitaxel plus gemcitabine with or without PEGPH20. A total of 135 patients received at least one dose of the study drugs. In all patients, the ORR (with vs. without PEGPH20: 41% vs. 34%; P=0.48) and PFS (with vs. without PEGPH20: 5.7 vs. 5.2 months; P=0.11) were similar. However, in those with high expressions of HA, the ORR was better in the PEGPH20 arm (52% vs. 24%; P=0.038). The PEGPH20 arm also showed a trend towards longer PFS (9.2 vs. 4.3 months; HR 0.39; 95% CI: 0.15–1.04; P=0.05) and better OS (12 vs. 9 months; HR 0.62; 95% CI: 0.26–1.46). The PEGPH20 group experienced more thromboembolic events, peripheral edema, muscle spasms, and neutropenia, but these toxicities were manageable and the incidence of thromboembolic events decreased substantially after prophylactic enoxaparin was implemented. A phase III study evaluating the efficacy of PEGPH20 in combination with nab-paclitaxel and gemcitabine in patients with HA-high metastatic pancreatic cancers is ongoing (NCT02715804).
Cancer stemness inhibitor
Cancer stem cells are referred to the subpopulations of cancer cells harboring extremely high potential for tumorigenesis and have been isolated from various GI cancers (53-55). Napabucasin (BBI-608) is a first-in-class cancer stemness inhibitor that targets Stat3-dependent gene transcription. In a phase Ib study, napabucasin was tested in combination with FOLFIRI and bevacizumab in patients with advanced CRCs (56). A total of 46 patients were enrolled and randomized to receive napabucasin plus FOLFIRI with or without bevacizumab. There were no DLTs observed in the study, and the most common grade 3 adverse events were diarrhea and fatigue. An objective response was achieved in 13 of the 40 evaluable patients (33%). Furthermore, among the 14 evaluable patients who were previously refractory to FOLFIRI, three achieved PR (25%). These results are intriguing and suggest that a cancer stemness inhibitor may re-sensitize refractory patients to chemotherapy. A phase III study of napabucasin is ongoing in patients with advanced CRCs (NCT02753127).
Other novel targets for GI cancer treatment
IMAB362 is a first-in-class monoclonal antibody against the tight junction protein claudin 18.2 (CLDN18.2), which is frequently expressed on gastric cancers (57). In the phase II FAST trial, patients with CLDN18.2-positive advanced gastric or gastroesophageal junction (GEJ) cancers were randomized 1:1 to receive epirubicin, oxaliplatin, and capecitabine (EOX) with or without IMAB362 (58). A total of 161 patients were randomized. IMAB362 plus chemotherapy significantly improved the median PFS (7.9 vs. 4.8 months; HR 0.47; 95% CI: 0.31–0.70; 1-sided P=0.0001) and OS (13.2 vs. 8.4 months; HR 0.51; 95% CI: 0.36–0.73; P=0.0001). Moreover, IMAB362 seemed to be more beneficial in those who had high expressions of CLDN18.2, defined as 2+ or 3+ CLDN18.2 expression in ≥70% of tumor cells. In these patients, the median OS with and without IMAB362 were 16.7 and 9.0 months (HR 0.45; P≤0.0005), respectively. Also of note, in this study, patients treated with EOX alone only had a median OS of 8.4 months, which was inferior to the median OS of 11.2 months with EOX in the REAL-2 trial (59). Incorporating IMAB362 with a better chemotherapy backbone may be worthy of investigation in future clinical studies.
Conclusions
Novel drugs targeting various antitumor mechanisms of GI cancers are under rapid development (Table 1). Inhibitors targeting growth factor pathways have shown encouraging results in early phase studies. By targeting cell surface receptors, the multikinase inhibitors cabozantinib and regorafenib have demonstrated significant clinical activity in advanced HCCs, and both are being tested in phase III studies. FGFR inhibitors may alter the treatment paradigm of cholangiocarcinoma with FGFR translocations. Agents targeting different levels of the cellular Raf-MEK-ERK signaling pathway are also being tested in GI cancers. In BRAF mutant CRCs, the combination of the BRAF inhibitor encorafenib and cetuximab has demonstrated promising efficacy and warrants further study in phase III trials. The MEK inhibitor cobimetinib seems to enhance the antitumor activity of PD-L1 inhibitor in patients with microsatellite stable CRCs. Drugs that modify the immunosuppressive tumor microenvironment seem to be active against GI cancers, but may need to be combined with other drugs such as chemotherapy or immunotherapy to enhance the antitumor effect. These agents include the TGF-β inhibitor galunisertib, CCR2 inhibitors PF-04136309 and CCX872-B, the IDO inhibitor indoximod, the CD40 agonizing antibody CP-870,893, and the recombinant hyaluronidase PEGPH20. Last but not least, drugs aimed at novel targets are breaking new ground in the treatment of GI cancers. The first-in-class cancer stemness inhibitor napabucasin in combination with chemotherapy has shown encouraging activity in patients with advanced CRCs and may overcome the problem of chemotherapy refractoriness in those who are heavily pretreated. In addition, the CLDN18.2 inhibitor IMAB362 is active against CLDN18.2-positive gastric cancers. Selecting the correct patient population and incorporating IMAB362 with better chemotherapy regimens would be the key for future studies.
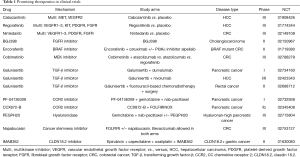
Full table
Acknowledgements
None.
Footnote
Conflicts of Interest: The authors have no conflicts of interest to declare.
References
- urwitz H, Fehrenbacher L, Novotny W, et al. Bevacizumab plus irinotecan, fluorouracil, and leucovorin for metastatic colorectal cancer. N Engl J Med 2004;350:2335-42.
- Cunningham D, Humblet Y, Siena S, et al. Cetuximab monotherapy and cetuximab plus irinotecan in irinotecan-refractory metastatic colorectal cancer. N Engl J Med 2004;351:337-45. [Crossref] [PubMed]
- Grothey A, Van Cutsem E, Sobrero A, et al. Regorafenib monotherapy for previously treated metastatic colorectal cancer (CORRECT): an international, multicentre, randomised, placebo-controlled, phase 3 trial. Lancet 2013;381:303-12. [Crossref] [PubMed]
- Llovet JM, Ricci S, Mazzaferro V, et al. Sorafenib in advanced hepatocellular carcinoma. N Engl J Med 2008;359:378-90. [Crossref] [PubMed]
- Le DT, Uram JN, Wang H, et al. PD-1 Blockade in Tumors with Mismatch-Repair Deficiency. N Engl J Med 2015;372:2509-20. [Crossref] [PubMed]
- El-Khoueiry AB, Melero I, Crocenzi TS, et al. Phase I/II safety and antitumor activity of nivolumab in patients with advanced hepatocellular carcinoma (HCC): CA209-040. J Clin Oncol 2015;33:abstr LBA101.
- Roberts PJ, Der CJ. Targeting the Raf-MEK-ERK mitogen-activated protein kinase cascade for the treatment of cancer. Oncogene 2007;26:3291-310. [Crossref] [PubMed]
- Verslype C, Cohn AL, Kelley RK, et al. Activity of cabozantinib (XL184) in hepatocellular carcinoma: Results from a phase II randomized discontinuation trial (RDT). J Clin Oncol 2012;30:abstr 4007.
- Bruix J, Merle P, Granito A, et al. LBA-03 Efficacy and safety of regorafenib versus placebo in patients with hepatocellular carcinoma (HCC) progressing on sorafenib: results of the international, randomized phase 3 RESORCE trial. Annals of Oncology 2016;27:ii140-ii141. [Crossref]
- Mross K, Buchert M, Frost A, et al. Vascular effects, efficacy and safety of nintedanib in patients with advanced, refractory colorectal cancer: a prospective phase I subanalysis. BMC Cancer 2014;14:510. [Crossref] [PubMed]
- Eswarakumar VP, Lax I, Schlessinger J. Cellular signaling by fibroblast growth factor receptors. Cytokine Growth Factor Rev 2005;16:139-49. [Crossref] [PubMed]
- Arai Y, Totoki Y, Hosoda F, et al. Fibroblast growth factor receptor 2 tyrosine kinase fusions define a unique molecular subtype of cholangiocarcinoma. Hepatology 2014;59:1427-34. [Crossref] [PubMed]
- Graham RP, Barr Fritcher EG, Pestova E, et al. Fibroblast growth factor receptor 2 translocations in intrahepatic cholangiocarcinoma. Hum Pathol 2014;45:1630-8. [Crossref] [PubMed]
- Borad MJ, Champion MD, Egan JB, et al. Integrated genomic characterization reveals novel, therapeutically relevant drug targets in FGFR and EGFR pathways in sporadic intrahepatic cholangiocarcinoma. PLoS Genet 2014;10:e1004135. [Crossref] [PubMed]
- Wang Y, Ding X, Wang S, et al. Antitumor effect of FGFR inhibitors on a novel cholangiocarcinoma patient derived xenograft mouse model endogenously expressing an FGFR2-CCDC6 fusion protein. Cancer Lett 2016;380:163-73. [Crossref] [PubMed]
- Javle MM, Shroff RT, Zhu A, et al. A phase 2 study of BGJ398 in patients (pts) with advanced or metastatic FGFR-altered cholangiocarcinoma (CCA) who failed or are intolerant to platinum-based chemotherapy. J Clin Oncol 2016;34:abstr 335.
- Rogers JE, Law L, Nguyen VD, et al. Second-line systemic treatment for advanced cholangiocarcinoma. J Gastrointest Oncol 2014;5:408-13. [PubMed]
- Brieau B, Dahan L, De Rycke Y, et al. Second-line chemotherapy for advanced biliary tract cancer after failure of the gemcitabine-platinum combination: A large multicenter study by the Association des Gastro-Enterologues Oncologues. Cancer 2015;121:3290-7. [Crossref] [PubMed]
- Davies H, Bignell GR, Cox C, et al. Mutations of the BRAF gene in human cancer. Nature 2002;417:949-54. [Crossref] [PubMed]
- Tran B, Kopetz S, Tie J, et al. Impact of BRAF mutation and microsatellite instability on the pattern of metastatic spread and prognosis in metastatic colorectal cancer. Cancer 2011;117:4623-32. [Crossref] [PubMed]
- Kopetz S, Desai J, Chan E, et al. Phase II Pilot Study of Vemurafenib in Patients With Metastatic BRAF-Mutated Colorectal Cancer. J Clin Oncol 2015;33:4032-8. [Crossref] [PubMed]
- Tabernero J, Van Geel R, Guren TK, et al. Phase 2 results: Encorafenib (ENCO) and cetuximab (CETUX) with or without alpelisib (ALP) in patients with advanced BRAF-mutant colorectal cancer (BRAFm CRC). J Clin Oncol 2016;34:abstr 3544.
- De Roock W, Claes B, Bernasconi D, et al. Effects of KRAS, BRAF, NRAS, and PIK3CA mutations on the efficacy of cetuximab plus chemotherapy in chemotherapy-refractory metastatic colorectal cancer: a retrospective consortium analysis. Lancet Oncol 2010;11:753-62. [Crossref] [PubMed]
- Ulivi P, Capelli L, Valgiusti M, et al. Predictive role of multiple gene alterations in response to cetuximab in metastatic colorectal cancer: a single center study. J Transl Med 2012;10:87. [Crossref] [PubMed]
- Ascierto PA, McArthur GA, Dréno B, et al. Cobimetinib combined with vemurafenib in advanced BRAFV600-mutant melanoma (coBRIM): updated efficacy results from a randomised, double-blind, phase 3 trial. Lancet Oncol 2016;17:1248-60. [Crossref] [PubMed]
- Long GV, Stroyakovskiy D, Gogas H, et al. Dabrafenib and trametinib versus dabrafenib and placebo for Val600 BRAF-mutant melanoma: a multicentre, double-blind, phase 3 randomised controlled trial. Lancet 2015;386:444-51. [Crossref] [PubMed]
- Ebert PJ, Cheung J, Yang YG, et al. MAP Kinase Inhibition Promotes T Cell and Anti-tumor Activity in Combination with PD-L1 Checkpoint Blockade. Immunity 2016;44:609-21. [Crossref] [PubMed]
- Bendell JC, Kim TW, Goh BC, et al. Clinical activity and safety of cobimetinib (cobi) and atezolizumab in colorectal cancer (CRC). J Clin Oncol 2016;34:abstr 3502.
- Yingling JM, Blanchard KL, Sawyer JS. Development of TGF-beta signalling inhibitors for cancer therapy. Nat Rev Drug Discov 2004;3:1011-22. [Crossref] [PubMed]
- Faivre SJ, Santoro A, Kelley RK, et al. A phase 2 study of a novel transforming growth factor-beta (TGF-β1) receptor I kinase inhibitor, LY2157299 monohydrate (LY), in patients with advanced hepatocellular carcinoma (HCC). J Clin Oncol 2014;32:abstr LBA173.
- Faivre SJ, Santoro A, Gane E, et al. A phase 2 study of galunisertib, a novel transforming growth factor-beta (TGF-β) receptor I kinase inhibitor, in patients with advanced hepatocellular carcinoma (HCC) and low serum alpha fetoprotein (AFP). J Clin Oncol 2016;34:abstr 4070.
- Mizutani K, Sud S, McGregor NA, et al. The chemokine CCL2 increases prostate tumor growth and bone metastasis through macrophage and osteoclast recruitment. Neoplasia 2009;11:1235-42. [Crossref] [PubMed]
- Qian BZ, Li J, Zhang H, et al. CCL2 recruits inflammatory monocytes to facilitate breast-tumour metastasis. Nature 2011;475:222-5. [Crossref] [PubMed]
- Mitchem JB, Brennan DJ, Knolhoff BL, et al. Targeting Tumor-Infiltrating Macrophages Decreases Tumor-Initiating Cells, Relieves Immunosuppression, and Improves Chemotherapeutic Responses. Cancer Res 2013;73:1128-41. [Crossref] [PubMed]
- Mantovani A, Sozzani S, Locati M, et al. Macrophage polarization: tumor-associated macrophages as a paradigm for polarized M2 mononuclear phagocytes. Trends Immunol 2002;23:549-55. [Crossref] [PubMed]
- Sanford DE, Belt BA, Panni RZ, et al. Inflammatory Monocyte Mobilization Decreases Patient Survival in Pancreatic Cancer: A Role for Targeting the CCL2/CCR2 Axis. Clin Cancer Res 2013;19:3404-15. [Crossref] [PubMed]
- Nywening TM, Wang-Gillam A, Sanford DE, et al. Targeting tumour-associated macrophages with CCR2 inhibition in combination with FOLFIRINOX in patients with borderline resectable and locally advanced pancreatic cancer: a single-centre, open-label, dose-finding, non-randomised, phase 1b trial. Lancet Oncol 2016;17:651-62. [Crossref] [PubMed]
- Conroy T, Desseigne F, Ychou M, et al. FOLFIRINOX versus gemcitabine for metastatic pancreatic cancer. N Engl J Med 2011;364:1817-25. [Crossref] [PubMed]
- Prendergast GC, Smith C, Thomas S, et al. Indoleamine 2,3-dioxygenase pathways of pathogenic inflammation and immune escape in cancer. Cancer Immunol Immunother 2014;63:721-35. [Crossref] [PubMed]
- Muller AJ, DuHadaway JB, Donover PS, et al. Inhibition of indoleamine 2,3-dioxygenase, an immunoregulatory target of the cancer suppression gene Bin1, potentiates cancer chemotherapy. Nat Med 2005;11:312-9. [Crossref] [PubMed]
- Bahary N, Garrido-Laguna I, Wang-Gillam A, et al. Results of the phase Ib portion of a phase I/II trial of the indoleamine 2,3-dioxygenase pathway (IDO) inhibitor indoximod plus gemcitabine/nab-paclitaxel for the treatment of metastatic pancreatic cancer. J Clin Oncol 2016;34:abstr 452.
- Bahary N, Garrido-Laguna I, Cinar P, et al. Phase 2 trial of the indoleamine 2,3-dioxygenase pathway (IDO) inhibitor indoximod plus gemcitabine/nab-paclitaxel for the treatment of metastatic pancreas cancer: Interim analysis. J Clin Oncol 2016;34:abstr 3020.
- Von Hoff DD, Ervin T, Arena FP, et al. Increased survival in pancreatic cancer with nab-paclitaxel plus gemcitabine. N Engl J Med 2013;369:1691-703. [Crossref] [PubMed]
- Guo J, Xiao JJ, Zhang X, et al. CD40 expression and its prognostic significance in human gastric carcinoma. Med Oncol 2015;32:63. [Crossref] [PubMed]
- Wu Y, Wang L, He X, et al. Expression of CD40 and growth-inhibitory activity of CD40 ligand in colon cancer ex vivo. Cell Immunol 2008;253:102-9. [Crossref] [PubMed]
- French RR, Chan HT, Tutt AL, et al. CD40 antibody evokes a cytotoxic T-cell response that eradicates lymphoma and bypasses T-cell help. Nat Med 1999;5:548-53. [Crossref] [PubMed]
- Diehl L, den Boer AT, Schoenberger SP, et al. CD40 activation in vivo overcomes peptide-induced peripheral cytotoxic T-lymphocyte tolerance and augments anti-tumor vaccine efficacy. Nat Med 1999;5:774-9. [Crossref] [PubMed]
- Eliopoulos AG, Davies C, Knox PG, et al. CD40 induces apoptosis in carcinoma cells through activation of cytotoxic ligands of the tumor necrosis factor superfamily. Mol Cell Biol 2000;20:5503-15. [Crossref] [PubMed]
- Beatty GL, Torigian DA, Chiorean EG, et al. A phase I study of an agonist CD40 monoclonal antibody (CP-870,893) in combination with gemcitabine in patients with advanced pancreatic ductal adenocarcinoma. Clin Cancer Res 2013;19:6286-95. [Crossref] [PubMed]
- Cheng XB, Kohi S, Koga A, et al. Hyaluronan stimulates pancreatic cancer cell motility. Oncotarget 2016;7:4829-40. [PubMed]
- Cheng XB, Sato N, Kohi S, et al. Prognostic impact of hyaluronan and its regulators in pancreatic ductal adenocarcinoma. PLoS One 2013;8:e80765. [Crossref] [PubMed]
- Hingorani SR, Harris WP, Hendifar AE, et al. High response rate and PFS with PEGPH20 added to nab-paclitaxel/gemcitabine in stage IV previously untreated pancreatic cancer patients with high-HA tumors: Interim results of a randomized phase II study. J Clin Oncol 2015;33:abstr 4006.
- Dalerba P, Dylla SJ, Park IK, et al. Phenotypic characterization of human colorectal cancer stem cells. Proc Natl Acad Sci U S A 2007;104:10158-63. [Crossref] [PubMed]
- Li C, Heidt DG, Dalerba P, et al. Identification of pancreatic cancer stem cells. Cancer Res 2007;67:1030-7. [Crossref] [PubMed]
- O'Brien CA, Pollett A, Gallinger S, et al. A human colon cancer cell capable of initiating tumour growth in immunodeficient mice. Nature 2007;445:106-10. [Crossref] [PubMed]
- Phase 1b extension study of cancer stemness inhibitor BB608 (napabucasin) administered in combination with FOLFIRI +/- bevacizumab (Bev) in patients (pts) with advanced colorectal cancer (CRC). J Clin Oncol 2016;34:abstr 3564.
- Sahin U, Koslowski M, Dhaene K, et al. Claudin-18 splice variant 2 is a pan-cancer target suitable for therapeutic antibody development. Clin Cancer Res 2008;14:7624-34. [Crossref] [PubMed]
- Al-Batran SE, Schuler MH, Zvirbule Z, et al. FAST: An international, multicenter, randomized, phase II trial of epirubicin, oxaliplatin, and capecitabine (EOX) with or without IMAB362, a first-in-class anti-CLDN18.2 antibody, as first-line therapy in patients with advanced CLDN18.2+ gastric and gastroesophageal junction (GEJ) adenocarcinoma. J Clin Oncol 2016;34:abstr LBA4001.
- Cunningham D, Starling N, Rao S, et al. Capecitabine and oxaliplatin for advanced esophagogastric cancer. N Engl J Med 2008;358:36-46. [Crossref] [PubMed]