Protons offer reduced bone marrow, small bowel, and urinary bladder exposure for patients receiving neoadjuvant radiotherapy for resectable rectal cancer
Introduction
The introduction of neoadjuvant therapy through short and long courses of radiation therapy for resectable rectal cancer has resulted in reduced relapse rates (1-3). Adding chemotherapy to preoperative long-course radiation has been shown to be superior to radiation alone (2), while preoperative chemoradiation (CRT) results in lower relapse rates and better sphincter preservation than postoperative CRT (3). As a result, preoperative CRT is now a standard of care in locally advanced rectal cancer. Nevertheless, despite neoadjuvant CRT, recurrence rates of locally advanced rectal cancer remain high with systemic recurrence in up to 30% to 40% of patients (1,3).
Historically, radiation was delivered using 3-dimensional conformal radiotherapy (3DCRT) techniques in a 3- or 4-field arrangement. The introduction of intensity-modulated radiation therapy (IMRT) has resulted in improved conformality; however, despite this improvement, organs outside of the planning target volume (PTV), including the bladder, small bowel, and pelvic bone marrow, may still receive a significant radiation dose.
Conventional photon radiation uses X-rays to deliver the dose to the target volume. X-ray therapy, however, results in a significant entrance and exit dose along the path of beam delivery in addition to subsequent dose to normal tissue. Compared to X-ray therapy, proton therapy is a form of charged-particle therapy that allows delivery of the equivalent X-ray dose or dose escalation while sparing normal tissue. More specifically, the properties of the spread-out Bragg peak (SOBP) allow improved sparing of non-targeted organs, with proton beams conformed to fit the exact depth and shape of the required target. Reducing the volume and exposure of normal pelvis and bone marrow to radiation will likely reduce long-term toxicity and preserve pelvic bone marrow, which is increasingly important in the setting of systemic recurrences where patients may require multiple lines of myelosuppressive chemotherapy.
In this study, we sought to compare proton therapy plans for patients treated with neoadjuvant CRT to IMRT and 3DCRT plans in an attempt to quantify the dosimetric benefit of proton therapy in a cohort of patients receiving neoadjuvant CRT.
Materials and methods
Under an institutional review board-approved study, 8 consecutive patients with resectable rectal cancers underwent treatment planning with 3DCRT, IMRT, and conformal proton therapy. All patients were simulated in the prone position with a full bladder and imaged on a Phillips Brilliance (Phillips Healthcare, Andover, MA) large-bore computed tomography (CT) scanner with a 60-cm field-of-view and 1-mm slices.
Target volumes and dose constraints
Initial target volumes (PTV1) were contoured using the guidelines in the Radiation Therapy Oncology Group (RTOG) anorectal atlas (4). The initial clinical target volume (CTV) consisted of the gross tumor volume (GTV) as determined by a combination of physical examination, colonoscopy, and diagnostic CT and/or magnetic resonance imaging (MRI) scan plus the entire mesorectum, including the perirectal fat and presacral space along with the internal iliac lymph nodes. Boost target volumes (PTV2) consisted of the GTV plus a 2-cm uniform expansion. The dose delivered to the PTV1 was 45 Gray (Gy) or Cobalt Gray Equivalent (CGE) in 25 fractions with a boost of 5.4 CGE in 3 fractions to the PTV2, resulting in a total dose of 50.4 CGE over 28 fractions.
Target goals were similar to those used in the RTOG 0822 protocol for resectable rectal cancer. For each treatment phase, 95% of the PTV received 100% of the target dose and 100% of the PTV received 95% of the target dose. Per the normal-tissue constraints, no more than 180 cm3 of small bowel received greater than 35 Gy, while no more than 40% of the femoral heads received greater than 40 Gy; V40Gy for the bladder was less than 40%.
3DCRT plans delivered the target doses via a standardized 3-field (posterior/anterior, right lateral, and left lateral) approach with a 2-to-1 field weighting by dose contributed to the target volume. IMRT plans delivered the initial 45 Gy following the planning and dose delivery guidelines of the RTOG 0822 protocol and a 5.4-Gy boost by following the same field angles as the initial plan. PT plans utilized a 3-field approach similar to the 3DCRT plans with a heavier weighting of the posterior field relative to the right and left lateral fields (3.1 to 1 to 1). To avoid excess skin toxicity, the maximum dose permitted to 1 cm2 of skin was 35 Gy. To account for air within the rectum when designing the proton plan, the Hounsfield units were overridden for the circumferential air-filled portion of the rectum.
Representative colorwash dose distributions for typical proton therapy, IMRT and 3DCRT plans are shown in Figure 1.
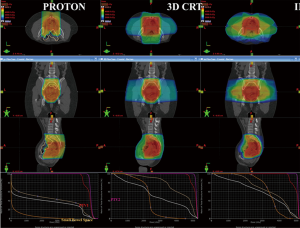
Statistics
Descriptive statistics (median and range) were used to characterize the disease-specific and dosimetric points of interest. A Wilcoxon signed rank sum test for nonparametric paired data was used to compare the 3DCRT and IMRT plans with the proton plans for the various dosimetric points, and to establish statistical significance, P≤0.05 (WinStat Microsoft Excel, Microsoft, Redmond, WA).
Results
Target volume coverage
All 3DCRT, IMRT, and proton plans met all normal-tissue constraints and were isoeffective in terms of PTV coverage.
Pelvic bone marrow dosimetry
The results for median pelvic bone marrow dosimetry comparing the 3 plans are shown in Table 1. At all dose levels evaluated, proton plans offered significantly reduced pelvic bone marrow exposure over 3DCRT and IMRT.
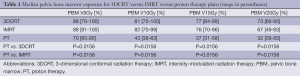
Full table
Small bowel and bladder dosimetry
The results for small bowel and bladder dosimetry are shown in Table 2. Proton therapy was statistically superior to 3DCRT with regard to small bowel exposure at all evaluated dose levels and with regard to the urinary bladder at the V40Gy level. The superiority of proton therapy over IMRT with regard to small bowel exposure was limited to the V10Gy and V20Gy levels. There was no significant improvement with protons compared to IMRT with regard to urinary bladder exposure.
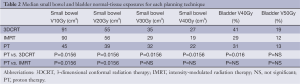
Full table
Discussion
We present the first known dosimetric study comparing 3DCRT, IMRT, and proton therapy plans for neoadjuvant CRT for resectable rectal cancer. The results show superior bone marrow sparing for proton therapy over IMRT and 3DCRT and better sparing of small bowel with proton therapy, particularly at low-dose thresholds.
As a result of its dosimetric advantages in certain tumors, such as childhood cancers (5-10) and skull base tumors (11-13), proton therapy is a well-established radiotherapy treatment technique. Furthermore a growing body of evidence is emerging indicating superior dosimetric profiles and sparing of normal tissue over 3DCRT, IMRT, or both in various other tumor sites, including lung tumors (14-16), lymphoma (17,18) and upper gastrointestinal (GI) tumors (19,20).
While radiation therapy for rectal cancer is a long-established practice and neoadjuvant CRT is a standard of care in the management of operable locally advanced rectal cancer (2,3,21,22), preoperative radiation is still delivered in most cancer centers using 3DCRT. Neoadjuvant CRT with 3DCRT, however, results in non-trivial rates of acute and late treatment toxicity from treatment as well as significant local and distant recurrence rates. In the German study (3) comparing pre- and postoperative CRT in which preoperative CRT was given to a dose of 50.4 Gy with 5 fluourouracil (5-FU) concurrent chemotherapy, the incidence of acute grade 3+ toxicity was 27% with a late grade 3+ toxicity rate at the 5-year follow-up in the preoperative group of 14%. In an updated report of this study (23), at the 11-year follow-up, the 10-year rate of cumulative local recurrence was 7.1% and the rate of distant metastases 29.8%.
In the Sauer study, 6% of patients in the preoperative group experienced grade 3+ haematological toxicity. In addition, with approximately 30% to 40% of patients recurring at 10 years, a large proportion of patients receiving neoadjuvant CRT will likely require future salvage chemotherapy. Thus, the significant sparing of bone marrow seen in our study with proton therapy over both IMRT and 3DCRT (P<0.05 for V5, V10, V15, and V20 for proton therapy versus IMRT and proton therapy versus 3DCRT) may be of substantial benefit. Indeed, sparing bone marrow through the use of proton therapy may reduce the compromise of delivery of CRT in the acute setting while preserving bone marrow function ahead of several lines of myelosuppressive chemotherapy that are delivered in the salvage setting (3).
Proton therapy offers the potential to reduce acute and late bowel toxicity from CRT compared to IMRT or 3DCRT in the treatment of rectal cancer. In our study, proton therapy plans had statistically significant superior sparing of the small bowel compared to both IMRT and 3DCRT for both V10 and V20. Although the median V30 and V40 for IMRT was slightly less than with proton therapy, this was not statistically significant. In this regard, by reducing the low-dose bowel volume irradiated, proton therapy may better allow for dose escalation or avoidance of treatment interruptions in the acute setting.
Current research in the neoadjuvant setting revolves around adding new chemotherapy agents to radiation: capecitabine has been shown to be equally efficacious as infusional 5-FU in the treatment of colon cancer (24,25) and the effectiveness of agents such as oxaliplatin, irinotecan, and bevacuzimab has led to these agents being piloted in early-phase trials of neoadjuvant rectal cancer.
Nevertheless, bowel toxicity can be a limiting factor in this setting; indeed, the phase II randomized RTOG 0247 trial comparing neoadjuvant radiation combined with capecitabine and oxaliplatin versus capecitabine and irinotecan was temporarily suspended due to excess grade 3+ GI toxicity from both the chemotherapy and the radiation. Several studies have shown a potential benefit with IMRT compared to 3DCRT in rectal cancer with regard to the small bowel dose (26,27). Such studies are the foundation to the hypothesis for the RTOG 0822 study, which involves using IMRT with concurrent multiagent chemotherapy to reduce small bowel exposure and therefore acute GI toxicity, thus enabling better dose delivery and dose escalation of concurrent chemotherapy. Similarly, proton therapy may permit additional small bowel sparing, allow chemotherapy dose escalation, and increased patient compliance.
Proton therapy plans in our study utilized a 3-field approach with uniform scanning. This field arrangement was chosen to avoid the excess skin dose with a single posterior field plan. Furthermore, uniform scanning allowed delivery of the dose to a greater depth in the pelvis than would be possible with double-scattered protons. Advancements in proton therapy, such as the introduction of pencil-beam scanning and with it intensity-modulated proton therapy, may result in proton therapy offering further dosimetric advantages over and above those seen in our study and may merit further investigation as intensity-modulated proton therapy becomes increasingly available.
Conclusions
In this small series of patients with rectal cancer undergoing neoadjuvant CRT for rectal cancer, proton therapy plans offered superior sparing of bone marrow and the small bowel compared to both IMRT and 3DCRT. The dosimetric advantages seen with proton therapy may therefore merit further investigation as a means of limiting the acute toxicity of neoadjuvant CRT and preserving both bone marrow and bowel function in advance of future myelosuppressive chemotherapy in the relapse setting.
Acknowledgements
Disclosure: The authors declare no conflict of interest.
References
- Improved survival with preoperative radiotherapy in resectable rectal cancer. Swedish Rectal Cancer Trial. N Engl J Med 1997;336:980-7. [PubMed]
- Gérard JP, Conroy T, Bonnetain F, et al. Preoperative radiotherapy with or without concurrent fluorouracil and leucovorin in T3-4 rectal cancers: results of FFCD 9203. J Clin Oncol 2006;24:4620-5. [PubMed]
- Sauer R, Becker H, Hohenberger W, et al. Preoperative versus postoperative chemoradiotherapy for rectal cancer. N Engl J Med 2004;351:1731-40. [PubMed]
- Myerson RJ, Garofalo MC, El Naqa I, et al. Elective clinical target volumes for conformal therapy in anorectal cancer: a radiation therapy oncology group consensus panel contouring atlas. Int J Radiat Oncol Biol Phys 2009;74:824-30. [PubMed]
- Miralbell R, Lomax A, Cella L, et al. Potential reduction of the incidence of radiation-induced second cancers by using proton beams in the treatment of pediatric tumors. Int J Radiat Oncol Biol Phys 2002;54:824-9. [PubMed]
- Kozak KR, Adams J, Krejcarek SJ, et al. A dosimetric comparison of proton and intensity-modulated photon radiotherapy for pediatric parameningeal rhabdomyosarcomas. Int J Radiat Oncol Biol Phys 2009;74:179-86. [PubMed]
- Yock T, Schneider R, Friedmann A, et al. Proton radiotherapy for orbital rhabdomyosarcoma: clinical outcome and a dosimetric comparison with photons. Int J Radiat Oncol Biol Phys 2005;63:1161-8. [PubMed]
- St Clair WH, Adams JA, Bues M, et al. Advantage of protons compared to conventional X-ray or IMRT in the treatment of a pediatric patient with medulloblastoma. Int J Radiat Oncol Biol Phys 2004;58:727-34. [PubMed]
- Lee CT, Bilton SD, Famiglietti RM, et al. Treatment planning with protons for pediatric retinoblastoma, medulloblastoma, and pelvic sarcoma: how do protons compare with other conformal techniques? Int J Radiat Oncol Biol Phys 2005;63:362-72. [PubMed]
- Krengli M, Hug EB, Adams JA, et al. Proton radiation therapy for retinoblastoma: comparison of various intraocular tumor locations and beam arrangements. Int J Radiat Oncol Biol Phys 2005;61:583-93. [PubMed]
- Sauer R, Becker H, Hohenberger W, et al. Preoperative versus postoperative chemoradiotherapy for rectal cancer. N Engl J Med 2004;351:1731-40. [PubMed]
- Munzenrider JE, Liebsch NJ. Proton therapy for tumors of the skull base. Strahlenther Onkol 1999;175 Suppl 2:57-63. [PubMed]
- Hug EB, Loredo LN, Slater JD, et al. Proton radiation therapy for chordomas and chondrosarcomas of the skull base. J Neurosurg 1999;91:432-9. [PubMed]
- Chang JY, Zhang X, Wang X, et al. Significant reduction of normal tissue dose by proton radiotherapy compared with three-dimensional conformal or intensity-modulated radiation therapy in Stage I or Stage III non-small-cell lung cancer. Int J Radiat Oncol Biol Phys 2006;65:1087-96. [PubMed]
- Nichols RC, Huh SN, Henderson RH, et al. Proton radiation therapy offers reduced normal lung and bone marrow exposure for patients receiving dose-escalated radiation therapy for unresectable stage iii non-small-cell lung cancer: a dosimetric study. Clin Lung Cancer 2011;12:252-7. [PubMed]
- Colaco RJ, Huh S, Nichols RC, et al. Dosimetric rationale and early experience at UFPTI of thoracic proton therapy and chemotherapy in limited-stage small cell lung cancer. Acta Oncol 2013;52:506-13. [PubMed]
- Hoppe BS, Flampouri S, Su Z, et al. Effective dose reduction to cardiac structures using protons compared with 3DCRT and IMRT in mediastinal Hodgkin lymphoma. Int J Radiat Oncol Biol Phys 2012;84:449-55. [PubMed]
- Hoppe BS, Flampouri S, Li Z, et al. Cardiac sparing with proton therapy in consolidative radiation therapy for Hodgkin lymphoma. Leuk Lymphoma 2010;51:1559-62. [PubMed]
- Nichols RC Jr, Huh SN, Prado KL, et al. Protons offer reduced normal-tissue exposure for patients receiving postoperative radiotherapy for resected pancreatic head cancer. Int J Radiat Oncol Biol Phys 2012;83:158-63. [PubMed]
- Nichols RC Jr, George TJ, Zaiden RA Jr, et al. Proton therapy with concomitant capecitabine for pancreatic and ampullary cancers is associated with a low incidence of gastrointestinal toxicity. Acta Oncol 2013;52:498-505. [PubMed]
- Bosset JF, Collette L, Calais G, et al. Chemotherapy with preoperative radiotherapy in rectal cancer. N Engl J Med 2006;355:1114-23. [PubMed]
- Bujko K, Nowacki MP, Nasierowska-Guttmejer A, et al. Long-term results of a randomized trial comparing preoperative short-course radiotherapy with preoperative conventionally fractionated chemoradiation for rectal cancer. Br J Surg 2006;93:1215-23. [PubMed]
- Sauer R, Liersch T, Merkel S, et al. Preoperative versus postoperative chemoradiotherapy for locally advanced rectal cancer: results of the German CAO/ARO/AIO-94 randomized phase III trial after a median follow-up of 11 years. J Clin Oncol 2012;30:1926-33. [PubMed]
- Twelves C, Gollins S, Grieve R, et al. A randomised cross-over trial comparing patient preference for oral capecitabine and 5-fluorouracil/leucovorin regimens in patients with advanced colorectal cancer. Ann Oncol 2006;17:239-45. [PubMed]
- Twelves C, Wong A, Nowacki MP, et al. Capecitabine as adjuvant treatment for stage III colon cancer. N Engl J Med 2005;352:2696-704. [PubMed]
- Duthoy W, De Gersem W, Vergote K, et al. Clinical implementation of intensity-modulated arc therapy (IMAT) for rectal cancer. Int J Radiat Oncol Biol Phys 2004;60:794-806. [PubMed]
- Guerrero Urbano MT, Henrys AJ, Adams EJ, et al. Intensity-modulated radiotherapy in patients with locally advanced rectal cancer reduces volume of bowel treated to high dose levels. Int J Radiat Oncol Biol Phys 2006;65:907-16. [PubMed]