Proton therapy may allow for comprehensive elective nodal coverage for patients receiving neoadjuvant radiotherapy for localized pancreatic head cancers
Background
Pancreatic cancer is the fifth leading cause of cancer mortality in the United States. In 2011, there were an estimated 44,030 new cases and 37,660 deaths (1). Curative therapy for patients with nonmetastatic disease necessarily includes extirpative surgery. Unfortunately, the surgical literature suggests a local-regional failure rate ranging from 50% to 80% for patients undergoing pancreaticoduodenectomy (2,3). Recognizing this concern, postoperative radiotherapy has been offered in an effort to increase the likelihood of local disease control. While the shortcomings of these studies have been well-described in the oncologic literature (4), the results of studies by the European Study Group for Pancreatic Cancer (ESPAC) suggest that postoperative X-ray-based radiotherapy fails to offer an improvement in survival over surgery and chemotherapy alone (5). The problems with postoperative radiation therapy are that (I) radiotherapy cannot be delivered until several weeks after surgery because of postoperative convalescence and (II) postoperative radiotherapy doses are limited by the large volume of transposed small bowel in the radiotherapy target volume.
Preoperative neoadjuvant radiotherapy would potentially avoid these problems. A drawback of preoperative X-ray-based radiotherapy, however, is that small bowel and gastric exposure in the neoadjuvant setting can complicate an already challenging major surgical intervention. Several dosimetric studies suggest that proton therapy has the potential to improve the therapeutic index over X-ray-based radiotherapy by reducing such normal-tissue exposure (6-10). Various clinical outcome studies also suggest low rates of gastrointestinal toxicity when protons are used to treat pancreatic cancers (11,12). Although many published studies on the use of neoadjuvant radiotherapy for patients with pancreatic cancer targeted the primary tumor and selective regional nodes (13-15), others only targeted the gross tumor with no specific effort to cover regional lymph nodes (16,17). In this setting, some nodal targets are ostensibly omitted in an effort to limit gastrointestinal toxicity, even though nodal metastases may be identified in 39% to 71% of these patients (3,18,19) at the time of surgery. The current study was undertaken to assess the feasibility of leveraging the improved therapeutic index of protons to deliver comprehensive elective nodal irradiation in the neoadjuvant setting.
Methods
Twelve consecutive patients with nonmetastatic cancers of the pancreatic head underwent treatment planning for neoadjuvant chemoradiation at our institution. Patients were immobilized using a standard wing-board and a lower extremity stabilizer. Four-dimensional computed tomography (CT) without contrast and three-dimensional CT with oral and intravenous contrast was performed. Patients were imaged on a Philips Brilliance large-bore CT scanner with a 60-cm field of view and 1-mm slices (Philips Healthcare, Amsterdam, the Netherlands). Gross tumor volume was contoured and guided by diagnostic CT scans with contrast, magnetic resonance imaging (MRI), and positron emission tomography (PET)-CT. Four-dimensional planning scans were utilized to define an internal clinical target volume (ICTV). Five-mm planning target volume (PTV) expansions were generated to establish the final PTV (labeled the PTV1) for the gross disease.
A second planning target volume (PTV2) was created using the initial PTV expanded to include the high-risk nodal targets as defined by the Radiation Therapy Oncology Group (RTOG) contouring atlas (20). Elective nodal expansions were based on either (I) the most proximal 1.0 to 1.5 cm of the celiac artery (CA); (II) the most proximal 2.5 to 3.0 cm of the superior mesenteric artery (SMA); (III) the portal vein segment extending from the bifurcation to the confluence with either the superior mesenteric vein (SMV) or splenic vein (SV); and (IV) the aorta from the most cephalad contour of either the celiac axis or portal vein to the bottom of the L2 vertebral body. If the gross tumor volume (GTV) contour extended to or below the bottom of L2, the aorta contour was extended towards the bottom of L3. To achieve elective nodal expansions on the CTV, the CA, SMA, and portal vein were expanded by 1.0 to 1.5 cm in all directions and the aortic region of interest was expanded 2.5 to 3.0 cm to the right, 1.0 cm to the left, 2.0 to 2.5 cm anteriorly, and 0.2 cm posteriorly towards the anterior edge of the vertebral body. The goal of the asymmetric expansion was to include the prevertebral nodal regions (retroperitoneal space) from the top of the portal vein or celiac axis (whichever was most superior) to the bottom of L2 (or L3 if the GTV location was too low).
Proton plans were generated on a Varian Eclipse 8.9 planning system (Varian Medical Systems Inc., Palo Alto, CA).
The proton treatment table top was inserted into the CT images manually and aligned with the CT table top so that the proton range and skin dose could be correctly calculated. A CT-Hounsfield unit to proton relative stopping-power conversion curve was used for proton range calculations. An effort was made to account for patient setup variability, respiratory motion, and delivery uncertainties, both by using appropriate distal and proximal margins to account for uncertainties in stopping-power conversion and by evaluating the presence of bowel and stomach contents in beam paths. The distal and proximal margins for each treatment field were estimated to be 2.5% of the beam range to the distal/proximal PTV plus 1.5 mm. Distal and proximal median spread-out Bragg peak (SOBP) expansions of 8 mm (range, 6-9 mm) and 10 mm (range, 8-12 mm) smearing margins were utilized for each beam.
Field apertures were designed to conform to the PTV in the beam’s-eye view, with an aperture margin adequate to account for the beam penumbrae (typically 10 mm uniformly around the PTV) depending on the beam range, except for edits that may have been necessary to avoid critical organs such as the kidneys. Range compensators were constructed with Lucite using median parameters for smearing margins and border smoothing of 6 and 8 mm, respectively.
A 2-field approach was utilized on all patients (posterior oblique: right lateral oblique) with a 3-to-1 weighting to the posterior field while limiting the spinal cord dose to less than 46 CGE. The heavy weighting of the posterior field allowed for coverage of the retroperitoneal region with minimal dose to the small bowel space anteriorly and to the body of the stomach left of the midline. Since no air-filled space (i.e., small bowel) would be situated in the beam path between the posterior proton source and the targeted tissues, there would be very little range uncertainty for the dose delivered from this field. The more lightly weighted right lateral-oblique field allowed for the degree of spinal cord sparing described above without delivering excessive dose to the liver. Since the lateral field had the potential to pass through a possibly air-filled small bowel space, however, the SOBP was generously expanded proximally and distally to compensate for the associated range uncertainty. This expansion did not result in meaningfully increased normal-tissue exposure due to the low dose delivered (approximately 12.6 Gy at 0.45 Gy per fraction).
Both PTV1 and PTV2 were prescribed to a total dose of 50.4 CGE; 95% of all PTVs received 100% of the target dose and 100% of the PTVs received at least 95% of the target dose. Normal tissue goals of particular interest were as follows: right kidney V18 to <70%; left kidney V18 Gy to <30%; small bowel/stomach V20 Gy to <50%, V45 Gy to <15%, V50 Gy to <10%, and V54 Gy <5%; liver V30 Gy to <60%; and spinal cord maximum to <46 Gy. Typical proton plans are illustrated in Figure 1.
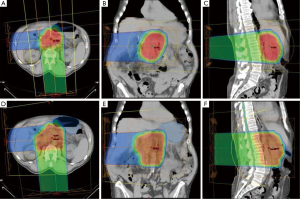
Results
The median PTV1 volume was 270.7 cm3 (range, 133.33-495.61 cm3). Median PTV2 volume was 541.75 cm3 (range, 399.44-691.14 cm3). All proton plans achieved the assigned PTV coverage. The median and range of normal-tissue exposures for each set of treatment plans are shown in Table 1.

Full Table
All 12 plans that treated the PTV1 volumes (gross tumor only) met all of the previously described normal tissue goals. Eight of the 12 plans that targeted the PTV2 volumes (gross tumor plus high-risk nodes) met all constraints. Of the 4 PTV2 plans that did not meet constraints, one failed to meet the bowel space constraint (V54, 9.6%; V50, 10.6%) constraint, one failed to meet the right kidney (V18, 85.5%) and bowel space constraints (V54, 17.1%; V50, 20.2%; V45, 23.8%), one failed to meet the gastric constraint (V50, 15.5%; V45, 23.9%), and one failed to meet the right kidney (V18, 75.8%) and gastric constraints (V50, 10.6%; V45, 19.0%).
Discussion
Various reports in the contemporary literature describe the use of neoadjuvant radiotherapy with or without chemotherapy for nonmetastatic resectable or marginally resectable pancreatic cancers (13-17). Table 2 presents a review of this literature. Neoadjuvant therapies provide numerous theoretical and practical advantages over postoperative treatment:
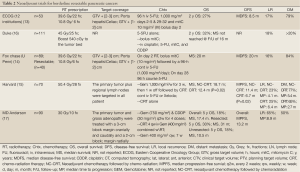
Full Table
- Malignant cells are more likely to oxygenate preoperatively, allowing radiation to be more effective through the production of radicals causing DNA damage;
- Preoperative treatment may reduce the likelihood of tumor spillage, dissemination, or implantation at the time of surgery;
- Since the irradiated bowel is likely to be resected at the time of pancreaticoduodenectomy, patients treated with preoperative radiotherapy may experience less long-term nutritional problems compared to patients irradiated postoperatively;
- With neoadjuvant therapy, there is no delay between systemic therapy and surgery, as opposed to adjuvant therapy where the delay is caused by postoperative recovery, possibly reducing the control of distant metastases;
- Neoadjuvant therapies may effectively downstage marginally resectable tumors and render them resectable.
These theoretical advantages are promising, but, to date, there are no randomized trials that directly compare neoadjuvant and adjuvant therapies.
In a phase 1 clinical trial, Hong et al. demonstrated the feasibility of hypofractionated neoadjuvant proton therapy with concomitant capecitabine for patients with resectable adenocarcinoma of the pancreatic head (11). Fifteen patients received doses ranging from 30 GyE in 10 fractions over 2 weeks to 25 GyE in 5 fractions over 1 week. Chemotherapy consisted of capecitabine at 825 mg/m2 twice daily. No dose-limiting toxicities were observed. Evaluation of 30-day postoperative mortality and morbidity showed no deaths or anastomotic leaks. Limited elective nodal irradiation was offered. Of note, 10 of 11 patients undergoing surgery had positive lymph nodes in the operative specimen.
Nichols et al. reported negligible weight loss and gastrointestinal toxicity in a group of 20 patients treated with conventionally fractionated protons and concomitant capecitabine (1,000 mg orally twice-daily) (12). Patients had marginally resectable (N=5), resected (N=5), or unresectable (N=10) disease and received planning target volume (PTV) proton doses ranging from 50.40 to 59.40 CGE. No elective nodal irradiation was offered to the patients with measurable gross disease. The median PTV volume was 406 cm3 (range, 244 to 1,811 cm3). For the 17 patients treated with a 2-field plan (posterior oblique and right lateral oblique) which minimized gastric and small bowel exposure, the median weight loss was only 1.1l bs (range, gain of 10.4 lbs to loss of 14.1 lbs) over the course of treatment. No patient experienced grade 2 or greater GI toxicity.
Conclusions
Protons allow for substantial gastric and small bowel sparing compared with X-rays in the setting of neoadjuvant radiotherapy for pancreatic cancer. This normal-tissue sparing offers the potential to reduce the risk of perioperative complications. As such, surgeons evaluating patients with resectable disease may ultimately be more willing to accept neoadjuvant radiotherapy if protons are to be used.
Additionally, in the majority of the cases we evaluated, we were able to expand the neoadjuvant radiotherapy field to safely cover both the gross tumor and the high-risk regional lymph nodes without significantly increasing the volume of critical normal tissues irradiated.
In light of this dosimetric data, as well as our clinical data showing a virtual absence of gastrointestinal toxicity when protons are used to treat pancreatic cancer, our current trial in development for neoadjuvant radiotherapy for patients with resectable and marginally resectable disease offers 50.40 CGE over 28 fractions to the above-described PTV2 volume with concomitant capecitabine (1,000 mg orally twice daily). If normal-tissue constraints cannot be met, a reduction in volume (to PTV1) will be made after 45.00 CGE (or as low as 39.60 CGE, if necessary).
Acknowledgements
Disclosure: The authors declare no conflict of interest.
References
- Siegel R, Ward E, Brawley O, et al. Cancer statistics, 2011: the impact of eliminating socioeconomic and racial disparities on premature cancer deaths. CA Cancer J Clin 2011;61:212-36. [PubMed]
- Tepper J, Nardi G, Sutt H. Carcinoma of the pancreas: review of MGH experience from 1963 to 1973. Analysis of surgical failure and implications for radiation therapy. Cancer 1976;37:1519-24. [PubMed]
- Kayahara M, Nagakawa T, Ueno K, et al. An evaluation of radical resection for pancreatic cancer based on the mode of recurrence as determined by autopsy and diagnostic imaging. Cancer 1993;72:2118-23. [PubMed]
- Abrams RA, Winter KA, Regine WF, et al. Failure to adhere to protocol specified radiation therapy guidelines was associated with decreased survival in RTOG 9704--a phase III trial of adjuvant chemotherapy and chemoradiotherapy for patients with resected adenocarcinoma of the pancreas. Int J Radiat Oncol Biol Phys 2012;82:809-16. [PubMed]
- Neoptolemos JP, Stocken DD, Friess H, et al. A randomized trial of chemoradiotherapy and chemotherapy after resection of pancreatic cancer. N Engl J Med 2004;350:1200-10. [PubMed]
- Hsiung-Stripp DC, McDonough J, Masters HM, et al. Comparative treatment planning between proton and X-ray therapy in pancreatic cancer. Med Dosim 2001;26:255-9. [PubMed]
- Kozak KR, Kachnic LA, Adams J, et al. Dosimetric feasibility of hypofractionated proton radiotherapy for neoadjuvant pancreatic cancer treatment. Int J Radiat Oncol Biol Phys 2007;68:1557-66. [PubMed]
- Nichols RC Jr, Huh SN, Prado KL, et al. Protons offer reduced normal-tissue exposure for patients receiving postoperative radiotherapy for resected pancreatic head cancer. Int J Radiat Oncol Biol Phys 2012;83:158-63. [PubMed]
- Johansson J, Isacsson U, Glimelius B. In regard to Zurlo et al., IJROBP 2000;48:277-288. Int J Radiat Oncol Biol Phys 2001;50:279-80. [PubMed]
- Zurlo A, Lomax A, Hoess A, et al. The role of proton therapy in the treatment of large irradiation volumes: a comparative planning study of pancreatic and biliary tumors. Int J Radiat Oncol Biol Phys 2000;48:277-88. [PubMed]
- Hong TS, Ryan DP, Blaszkowsky LS, et al. Phase I study of preoperative short-course chemoradiation with proton beam therapy and capecitabine for resectable pancreatic ductal adenocarcinoma of the head. Int J Radiat Oncol Biol Phys 2011;79:151-7. [PubMed]
- Nichols RC Jr, George TJ, Zaiden RA Jr, et al. Proton therapy with concomitant capecitabine for pancreatic and ampullary cancers is associated with a low incidence of gastrointestinal toxicity. Acta Oncol 2013;52:498-505. [PubMed]
- Hoffman JP, Lipsitz S, Pisansky T, et al. Phase II trial of preoperative radiation therapy and chemotherapy for patients with localized, resectable adenocarcinoma of the pancreas: an Eastern Cooperative Oncology Group Study. J Clin Oncol 1998;16:317-23. [PubMed]
- Meszoely IM, Wang H, Hoffman JP. Preoperative chemoradiation therapy for adenocarcinoma of the pancreas: The Fox Chase Cancer Center experience, 1986-2003. Surg Oncol Clin N Am 2004;13:685-96. [PubMed]
- Arvold ND, Ryan DP, Niemierko A, et al. Long-term outcomes of neoadjuvant chemotherapy before chemoradiation for locally advanced pancreatic cancer. Cancer 2012;118:3026-35. [PubMed]
- White RR, Tyler DS. Neoadjuvant therapy for pancreatic cancer: the Duke experience. Surg Oncol Clin N Am 2004;13:675-84. [PubMed]
- Varadhachary GR, Wolff RA, Crane CH, et al. Preoperative gemcitabine and cisplatin followed by gemcitabine-based chemoradiation for resectable adenocarcinoma of the pancreatic head. J Clin Oncol 2008;26:3487-95. [PubMed]
- Griffin JF, Smalley SR, Jewell W, et al. Patterns of failure after curative resection of pancreatic carcinoma. Cancer 1990;66:56-61. [PubMed]
- Showalter TN, Winter KA, Berger AC, et al. The influence of total nodes examined, number of positive nodes, and lymph node ratio on survival after surgical resection and adjuvant chemoradiation for pancreatic cancer: a secondary analysis of RTOG 9704. Int J Radiat Oncol Biol Phys 2011;81:1328-35. [PubMed]
- Abrams RA, Goodman KA, Ben-Josef E, et al. Pancreas atlas: consensus panel contouring atlas for the delineation of the clinical target volume in the postoperative treatment of pancreatic cancer. Available online: http://www.rtog.org/CoreLab/ContouringAtlases/PancreasAtlas.aspx