Adaptive radiation dose escalation in rectal adenocarcinoma: a review
Introduction
The role of radiation therapy in the treatment of rectal cancer has evolved over the past several decades. The current standard of care consists of pre-operative concurrent chemotherapy and radiotherapy (chemo-RT). Such a treatment strategy has further enhanced the pelvic tumor control of advanced surgical techniques such as total mesorectal excision (TME) and offered greater chance for sphincter preservation with lower rates of toxicity compared to post-operative radiotherapy, or TME alone (1,2).
Unfortunately, the inclusion of surgical resection as standard of care for rectal cancer patients carries implications for quality of life and toxicity (3). The concept of organ preservation in rectal cancer treatment was pioneered by surgeon Angelita Habr-Gama who noted that a subset of patients (about 27%) achieved a complete response after chemoradiation alone and had similar outcomes as those who underwent chemoradiation and subsequent TME, but without the added morbidity that came with surgery (4,5). Their group validated the ‘watch and wait’ strategy by showing that recurrences in the non-operative groups could be managed by salvage surgery with over 90% success (6). Multiple series have been published over the past five years that have demonstrated the efficacy and safety of a ‘watch and wait’ strategy (7).
The efficacy and safety of neoadjuvant chemoradiotherapy (CRT) have been demonstrated by a number of studies, most of which utilize low doses of radiation from 45–50.4 Gy. Indeed, several of the non-operative series have used these moderate doses. Despite these relatively low doses of radiation therapy, such studies, on average, produce pathologic complete responses (pCR) in 10–27% of patients, with clusters of studies reporting closer to 10% (8-11), and few at 27% and above (5,12). The value of a pCR after CRT has been validated as an indicator of increased chance for disease-free survival (13). Efforts to optimize the pCR rate from chemoradiation in rectal cancers have been ongoing. The impact of multiple factors, such as chemotherapy type and radiation dose escalation above 45 Gy have been postulated to be correlated with the degree of response (14).
Of interest, the impact of radiation dose escalation beyond 50.4 Gy on pCR rates has been examined with a recent meta-analysis of patients treated with doses over 60 Gy which showed increased pCR rates (20%) and acceptable short-term toxicity (15). This same group is furthering their investigation into radiotherapy dose escalation in an ongoing prospective trial (16). The rationale behind radiation dose escalation as an avenue to increase pCR rates is based on studies that have shown increased pCR rates and long-term survival in a dose-dependent manner (17), and a trend toward increased pCR rates and disease-free survival with increasing dose (18). Furthermore, a dose-response model derived from patients treated with a combination of external-beam radiation and brachytherapy to doses of 50.4–70 Gy showed a clear dose-response with a predicted pCR rate of 50% at 92 Gy (19).
While the effect of a boost beyond historic doses of 45–50.4 Gy is under current investigation, there remains a gap in the literature delineating effective methods of planning and applying a radiotherapy boost. Specifically the integration of novel imaging techniques, including positron emission tomography (PET) and magnetic resonance imaging (MRI), into selective application of a radiation boost strategy is poorly understood. We sought to review the existing literature of contemporary and personalized radiation therapy boost strategies. Moreover, we aim to provide potential future directions for the integration of innovative response assessment strategies into selective radiation therapy dose escalation for patients with rectal adenocarcinoma.
Radiographic assessment of rectal cancer response to therapy
Magnetic resonance imaging (MRI) response assessment
Monitoring the response of rectal cancer to chemoradiation by non-invasive methods is a vital component of a non-operative management strategy along with a selective and personalized radiation boost strategy. The ability to assess rectal tumors for their treatment response is an area of research that has expanded dramatically over the past 5 years (20). MRI presents a particularly novel and appealing modality to enable such response assessment and an adaptive radiation therapy boost strategy. This is particularly true secondary to the rapid expansion of image guided radiation therapy and specifically linear accelerators equipped with MRI guidance (21,22). MRI offers several considerable advantages over traditional computed tomography (CT) based imaging, particularly for primary tumor response assessment with quantitative sequences (23). One such example is the “apparent diffusion coefficient” (ADC), which is a quantitative value derived from diffusion weighted imaging (DWI) MRI that reflects tissue cellularity, organization and cell membrane permeability (24). This technique has shown promise in predicting complete responses of rectal cancer to CRT in recent years, and poses the benefit of being a quantitative method which is preferred for longitudinal studies and meta-analysis. Overall, DWI MRI is an attractive imaging modality for cancer staging and re-staging because it is noninvasive, relatively quick and does not utilize additional contrast agents or ionizing radiation (25). Multiple studies have shown significant differences in mean tumor ADCs between responders and non-responders after CRT treatment, and that a lower mean pre-treatment ADC correlates to better responses to CRT (26-29). One such example shows the potential of MRI integration into a course of radiation therapy treatment (30). Sun et al. showed that an increase in mean tumor ADC at approximately one week into CRT, along with a low pre-CRT mean ADC correlated with higher rates of response to chemo-RT (30). However, data examining the acquisitions of rectal MRI during a course of radiation therapy are limited.
Positron emission tomography (PET) based response assessment
18F-fluorodeoxyglucose PET (18F-FDG PET) allows for visualization of fluorinated glucose concentrations, indicating tissue with high metabolic activity, including cancers. For this reason, FDG PET is a powerful tool for tumor staging. Additionally, changes in metabolic activity can be monitored after treatments, and can be tracked with the semi-quantitative standardized uptake value (SUV). FDG SUV changes have been correlated with pathologic response to treatment in multiple cancers, including rectal cancer (31). The specific utility of PET, and PET combined with CT in predicting response to chemoradiation is a subject currently under intense investigation. Recent analysis of pooled individual patient data showed that pre-CRT PET/CT had a low positive predictive value for pCR (44%). Moreover, the absence of FDG avidity early in treatment with Chemo-RT has been shown to be indicative of non-responders (31-33). Interestingly, combining the ADC from DW-MRI and the SUVmax from PET to detect changes in tissue structure at the cellular level appears to more accurately reflect rectal cancer response to chemo-RT (34). Unfortunately, PET/CT has proven unreliable in providing accurate re-staging at the completion of chemo-RT (35-37). Additionally, PET/CT provides a difficult logistical and economic barrier to routine acquisition during the course of chemo-RT as radioisotopes carry considerable cost and logistical burden to their use.
Methods of radiation therapy dose escalation
3-dimentional conformal radiation therapy (3D-CRT)
The use of 3D-CRT represents the historical standard of care for patients with rectal adenocarcinoma. 3D-CRT utilizes CT to map a 3D target volume, and deliver conformal radiation beams in the shape of the tumor. This advancement allows for decreased toxicity to normal surrounding tissues and boosting of the tumor to higher radiation doses (38). Recent dose escalation studies using 3D-CRT have shown promise as a key component of achieving higher rates of pathological complete responses than with traditional chemoradiation alone (39).
In a study by Mohiuddin et al., patients treated up to 60 Gy by 3D-CRT with 5-FU chemotherapy had favorable responses, with an acceptable toxicity profile. Diarrhea was the most common acute toxicity at a grade 3, with no grade 4 or 5 toxicities in any category observed. This study illustrates a potential opportunity for dose escalation by 3D-CRT. The total reported pCR rates were 13% (2/15) in patients who received less than 55 Gy, and 44% (8/18) in patients who received over 55 Gy. Interestingly higher pCR rates occurred (66%) with continuous infusion vs bolus of 5-FU (9.5%) (40). In a phase 2 follow-up study by Mohiuddin et al., 106 patients were randomized to continuous venous infusion of 5-FU plus RT boosted to 55.2 for T3 cancers and 60 Gy for T4 cancers. The second treatment arm consisted of 5-FU plus irinotecan and 50.4 Gy for T3 and 54 Gy for T4 tumors. PCR rates were 30% in Arm 1 and 26% in Arm 2 (41).
Closely reproducing these results, is a series by Pfeiffer et al., in which 18 patients with unresectable or recurrent tumors underwent 60 Gy of radiation in 30 fractions with tegafur/uracil (UFT) chemotherapy. Of the 18 patients, 2 (11%) experienced a pathological complete response (42). In a phase II clinical trial by Movsas et al., a 3D-CRT boost was taken to 61.8 Gy with 5-FU in patients with bulky, locally advanced rectal cancer. They found downstaging in 50% of patients (43). In a study by Vestermark et al., rectal cancer patients were treated with a 60 Gy boost to the gross tumor volume in 30 fractions with concurrent chemotherapy. Of the patients who underwent resection, 33% (5/15) had a pathological complete response (39). In a unique study by Engineer et al., patients with clinically unresectable rectal cancer received 45 Gy of EBRT with concurrent oral capecitabine or EBRT alone boosted to 60 Gy. Patients experienced a pCR rate of 7% and 11% for radiation alone vs. radiation + chemo (44). The lower pCR rate in this study is perhaps not unexpected, as the dose escalated treatment arm did not include concurrent chemotherapy.
Together, these studies are difficult to interpret with radiation doses up to 60 Gy yielding dramatic ranges of pCR rates between 11% and 66%. Heterogeneity of patient populations, pathologic specimen processing, and treatment protocols make direct comparisons between studies difficult, although it is clear that escalated doses of radiation delivered by 3D-CRT trend toward higher pCR rates often with acceptable toxicity profiles. This group of studies also emphasizes the importance of optimizing chemotherapy regimens in concert with radiotherapy. This paradigm was also supported by the result of Engineer’s study, in which chemoradiation outperformed radiation alone (44). However, 3D-CRT strategies carry limitations in their ability to conformally address a circumferential target. In addition, they provide higher doses to critical local structures, such as the femoral necks and urinary bladder, which may limit the total radiation dose achievable when embarking upon dose escalation (45).
Intensity modulated radiotherapy (IMRT) boost strategies
IMRT is an advanced form of 3D-CRT in which the radiation beam intensity can be changed, or modulated, during treatment. The ability to deliver a range of radiation doses to the tumor bed allows for a simultaneous integrated boost (SIB) as well as minimizing doses to organs at risk, potentially enabling dose escalated radiation therapy to the tumor (46). In a study by Alongi et al., patients treated with IMRT boosting to 60 Gy in 30 fractions with concurrent capecitabine, resulted in a pCR rate of 17.5% with no grade 3 or higher toxicities (47). The low toxicity profile of this treatment regimen suggests that higher dose escalation via IMRT could be an appealing strategy. Two very similar studies by Ballonoff et al., and Freedman et al., analyzed dose escalation to 55 Gy using IMRT with oral capecitabine (48,49). Interestingly, the main difference between the studies, was that in the Freedman et al. study, capecitabine was administered 7 days per week, while in the Ballonoff study, it was administered 5 days per week with radiation therapy. Reasons for the divergent responses to similar treatments in pCR rates were attributed to the subjectivity of pathological evaluation, coupled with an early termination of the Freedman study due to acute toxicities (48,49). These two examples illustrate that escalating radiation therapy doses with IMRT may be feasible, however toxicity must be carefully monitored in such an approach. A phase II trial by Zhu et al., showed a promising pCR rate of 23.7% in stage II and III rectal cancer with a treatment regimen of 55 Gy to the primary tumor via IMRT, concurrent oxaliplatin and capecitabine and an additional course of Xelox 2 weeks post chemo-RT (50). Cubillo et al. examined patients treated with 57.5 Gy by SIB IMRT with chemotherapy tailored to tumor genotypes. In addition, they monitored PET SUV response before and after chemo-RT. Of the 16 patients, 8 (50%) experienced a pCR. Interestingly, the PET SUV change was a poor predictor of pCR, with only 2 of the 8 patients who experienced a tumor regression grade 4 showing a complete PET response. Additionally, one individual experienced a pCR with a negligible change of PET SUV (51). In a follow-up study Hernando et al. used the same IMRT radiation boost technique with the substitution of a standardized chemotherapy regimen of capecitabine for the customized regimens used previously. The results were understandably less dramatic than for the customized chemotherapies, with a pCR rate of 30.6% (51). These two series illustrate nicely the value of simultaneous integrated boost via IMRT for rectal cancer, which achieved a higher than average 30.6% pCR rate. On the other end of the spectrum, the only study with a radiation boost by IMRT without chemotherapy is a Belgian study in which patients were treated with up to 55.2 Gy. The pCR rate was low at 8% of the 108 participants, again illustrating the importance of concurrent chemotherapy (52). Finally, a recently published single arm phase II trial, Hong and colleagues evaluated the toxicity of IMRT, which did not appear to reduce the rates of GI toxicity, however a selective dose escalation strategy was not attempted in this series (53).
Brachytherapy boost strategies
Endorectal brachytherapy involves temporary insertion of radioactive material into the rectal lumen, delivering therapeutic radiation to the tumor. This technique has largely been used for adjuvant or palliative treatment in patients with rectal cancer (54). Endorectal brachytherapy has advanced from a relatively crude procedure that irradiates the entire rectum circumference, to a highly conformal technique with the use of 3D-CT planning and partial shielding to spare normal tissue adjacent to and opposite the rectal tumor (55). Studies on the use of brachytherapy as the sole neoadjuvant radiation treatment for rectal cancer are limited with the total doses reaching 26 Gy (56,57). Endorectal brachytherapy has also been used to escalate radiation doses beyond 50.4 Gy in conjunction with EBRT with mixed results. In 2006, Jakobsen et al. used EBRT boosted to 60 Gy, plus an additional boost of 5 Gy via brachytherapy. Results were promising with a pCR rate of 27%, attributed by the authors to the high doses of radiation used, with a favorable side-effect profile consisting of diarrhea as the only grade 3 toxicity. In a follow-up study, Jakobsen et al. added a COX-2 inhibitor to the regimen. The goal of the study was to determine the feasibility of adding a COX-2 inhibitor as a radiosensitizing agent to CRT. The COX-2 inhibitor was found to cause a severe maculopapular rash. However, the toxicity profile aside from the skin manifestations was reasonably low, with only 1 incidence of grade 3 diarrhea and leukopenia, while 21% of the patients experienced a pCR, a result that is comparable to the prior study considering the larger tumors treated in the second series (24% T4). Sun Myint et al. followed 34 patients treated with CRT consisting of 45 Gy EBRT, and a boost of 10 Gy via high dose rate brachytherapy. Patients experienced a favorable 31% pCR rate, with acceptable toxicity (58). A randomized trial published in 2012 by a Danish group authored by Jakobsen et al. enrolled 248 patients with T3-T4 tumors, and treated them with 50.4 Gy by EBRT, with an additional 10 Gy brachytherapy boost in the experimental arm. The group found a pCR rate of 18% in both arms, with only one notable difference in response: a 28% to 48% increase in response rate in T3 tumors (59). Published in 2014, a follow-up of the same patients showed that despite improved initial responses, these results did not correspond to long-term responses, and the lack of difference in pCR rate between the groups was reflected in the similar 5-year survival of the patients (60). A subsequent prospective trial by the Danish group utilized 60 Gy via IMRT, with an additional 5 Gy brachytherapy boost to test the watchful waiting strategy in patients with T2-T3 tumors. The outcomes were excellent with a 78% clinical complete response rate, and local control in 58% of patients at 2 years without surgical intervention (61). Together, these studies indicate that brachytherapy may have a role in an organ sparing strategy, particularly when used to add to an already escalated dose delivered by external beam radiation therapy. Additionally, and unsurprisingly, the effect of brachytherapy boost may be most effective with smaller tumors, a partial explanation to this is the fact that brachytherapy is an inherently local treatment, and does not effectively treat at-risk or involved lymph nodes, necessitating an additional boost strategy (55).
Selective and adaptive boost strategies
Patients receiving escalated doses of radiation typically receive a boost to the gross tumor volume (GTV) (42). Delivery of a radiotherapy boost has evolved with the advancement of radiotherapy delivery techniques to a simultaneous integrated boost (SIB) which allows for boost to the GTV without extending the overall treatment period, as utilized with the IMRT strategies mentioned in the above studies (62). In general, the imaging modality used in the dose-escalation planning and adaption for rectal cancer has not been emphasized in the literature as shown in Table 1. This is surprising, due to the advantages of using MRI in staging and planning RT for rectal cancer (63). It seems that rectal adenocarcinoma would be optimally suited for an adaptive boost strategy using radiologic response through either PET or MRI acquired during the course of chemo-RT. In one such example, Alongi et al., utilized PET/CT to plan the radiotherapy boost, and identified hypermetabolic areas that included primary tumor, mesorectum and involved lymph nodes. Patients treated with this protocol received 60 Gy of boost to identified targets yet had a 17.5% pCR rate. The boost strategy did not appear to impact tumor down-staging and PET/CT was not predictive of pCR, as there was no correlation between pre-treatment SUV-max and pCR (47). However, this boost strategy did not incorporate an intra-treatment assessment of rectal tumor response to therapy. An example of a truly adaptive strategy was presented by Avallone et al. who showed that early changes in mean PET SUV after 12 days of CRT, correlated with improved tumor responses, and 5-year relapse-free survival (64). Furthermore, Leccisotti et al. showed that a lack of early changes in SUV in response to CRT correlates with non-responders, and could be used to rapidly adapt the treatment course (65). One considerable limitation of PET is the cost and logistical challenge associated with the acquisition of these images. Alternatively, MRI may prove a powerful tool in selective dose escalation for patients with rectal adenocarcinoma. As the availability of MR guided radiation therapy systems expands, this may allow for real time adaptive treatment strategies. In one such early example, Passoni et al. employed a unique adaptive strategy that involved re-imaging with CT and MRI mid-treatment, and re-planning the target volume based on the residual tumor (66). As MR guided radiotherapy therapy systems become increasingly common, further investigation into adaptive boost strategies using MR guided systems presents a unique and promising opportunity.
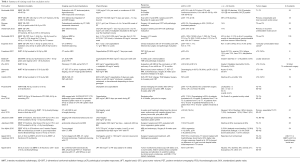
Full table
Conclusions and future directions
There appears to be a role for further evaluation of dose escalation in patients with rectal adenocarcinoma, particularly as it relates to organ preservation. With respect to the optimal RT planning strategy for rectal cancer, further investigation into the value of MRI-predominant adaptive treatment strategies should be considered based on superior soft-tissue detail along with the biological adaptation provided with advanced MR techniques (67,68). Delivery of highly conformal and adaptive doses of radiotherapy, coupled with MRI-guided therapy systems, may allow for higher rates of pCR and may offer improved rates of organ preservation. A list of ongoing clinical trials can be found in Table 2. While many boost trials for rectal cancer are ongoing, few use a true biologically adaptive or selective approach for the incorporation of radiation dose escalation. There is room for further investigation into novel methods of adaptive boost strategies and incorporation of novel imaging techniques for patients with rectal adenocarcinoma.
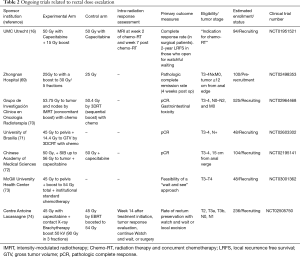
Full table
Acknowledgements
Funding: This publication was supported by the National Center for Research Resources and the National Center for Advancing Translational Sciences, National Institutes of Health, through Grant Number UL1TR001436.
Footnote
Conflicts of Interest: Elekta Inc. provides research support to the Medical College of Wisconsin Department of Radiation Oncology. In addition, WA Hall, MD reports research support from the American Cancer Society and the National Institutes of Health.
Disclaimer: Its contents are solely the responsibility of the authors and do not necessarily represent the official views of the NIH.
References
- Peeters KC, Marijnen CA, Nagtegaal ID, et al. The TME trial after a median follow-up of 6 years: increased local control but no survival benefit in irradiated patients with resectable rectal carcinoma. Ann Surg 2007;246:693-701. [Crossref] [PubMed]
- Sauer R, Liersch T, Merkel S, et al. Preoperative versus postoperative chemoradiotherapy for locally advanced rectal cancer: results of the German CAO/ARO/AIO-94 randomized phase III trial after a median follow-up of 11 years. J Clin Oncol 2012;30:1926-33. [Crossref] [PubMed]
- Luna-Perez P, Rodriguez-Ramirez S, Vega J, et al. Morbidity and mortality following abdominoperineal resection for low rectal adenocarcinoma. Rev Invest Clin 2001;53:388-95. [PubMed]
- Habr-Gama A, Perez RO, Nadalin W, et al. Operative versus nonoperative treatment for stage 0 distal rectal cancer following chemoradiation therapy: long-term results. Ann Surg 2004;240:711-7; discussion 717-8. [PubMed]
- Habr-Gama A, Perez RO, Proscurshim I, et al. Patterns of failure and survival for nonoperative treatment of stage c0 distal rectal cancer following neoadjuvant chemoradiation therapy. J Gastrointest Surg 2006;10:1319-28; discussion 1328-9. [Crossref] [PubMed]
- Habr-Gama A, Gama-Rodrigues J, Sao Juliao GP, et al. Local recurrence after complete clinical response and watch and wait in rectal cancer after neoadjuvant chemoradiation: impact of salvage therapy on local disease control. Int J Radiat Oncol Biol Phys 2014;88:822-8. [Crossref] [PubMed]
- Goodman KA. Definitive Chemoradiotherapy ("Watch-and-Wait" Approach). Semin Radiat Oncol 2016;26:205-10. [Crossref] [PubMed]
- Hiotis SP, Weber SM, Cohen AM, et al. Assessing the predictive value of clinical complete response to neoadjuvant therapy for rectal cancer: an analysis of 488 patients. J Am Coll Surg 2002;194:131-5; discussion 135-6. [Crossref] [PubMed]
- Maas M, Beets-Tan RG, Lambregts DM, et al. Wait-and-see policy for clinical complete responders after chemoradiation for rectal cancer. J Clin Oncol 2011;29:4633-40. [Crossref] [PubMed]
- Nyasavajjala SM, Shaw AG, Khan AQ, et al. Neoadjuvant chemo-radiotherapy and rectal cancer: can the UK watch and wait with Brazil? Colorectal Dis 2010;12:33-6. [Crossref] [PubMed]
- Seong J, Cho JH, Kim NK, et al. Preoperative chemoradiotherapy with oral doxifluridine plus low-dose oral leucovorin in unresectable primary rectal cancer. Int J Radiat Oncol Biol Phys 2001;50:435-9. [Crossref] [PubMed]
- Lambrecht M, Deroose C, Roels S, et al. The use of FDG-PET/CT and diffusion-weighted magnetic resonance imaging for response prediction before, during and after preoperative chemoradiotherapy for rectal cancer. Acta Oncol 2010;49:956-63. [Crossref] [PubMed]
- Maas M, Nelemans PJ, Valentini V, et al. Long-term outcome in patients with a pathological complete response after chemoradiation for rectal cancer: a pooled analysis of individual patient data. Lancet Oncol 2010;11:835-44. [Crossref] [PubMed]
- Sanghera P, Wong DW, McConkey CC, et al. Chemoradiotherapy for rectal cancer: an updated analysis of factors affecting pathological response. Clin Oncol (R Coll Radiol) 2008;20:176-83. [Crossref] [PubMed]
- Burbach JP, den Harder AM, Intven M, et al. Impact of radiotherapy boost on pathological complete response in patients with locally advanced rectal cancer: a systematic review and meta-analysis. Radiother Oncol 2014;113:1-9. [Crossref] [PubMed]
- Burbach JP, Verkooijen HM, Intven M, et al. Randomized controlled trial for pre-operAtive dose-escaLation BOOST in locally advanced rectal cancer (RECTAL BOOST study): study protocol for a randomized controlled trial. Trials 2015;16:58. [Crossref] [PubMed]
- Chan AK, Wong AO, Langevin J, et al. Preoperative chemotherapy and pelvic radiation for tethered or fixed rectal cancer: a phase II dose escalation study. Int J Radiat Oncol Biol Phys 2000;48:843-56. [Crossref] [PubMed]
- Wiltshire KL, Ward IG, Swallow C, et al. Preoperative radiation with concurrent chemotherapy for resectable rectal cancer: effect of dose escalation on pathologic complete response, local recurrence-free survival, disease-free survival, and overall survival. Int J Radiat Oncol Biol Phys 2006;64:709-16. [Crossref] [PubMed]
- Appelt AL, Ploen J, Vogelius IR, et al. Radiation dose-response model for locally advanced rectal cancer after preoperative chemoradiation therapy. Int J Radiat Oncol Biol Phys 2013;85:74-80. [Crossref] [PubMed]
- Memon S, Lynch AC, Bressel M, et al. Systematic review and meta-analysis of the accuracy of MRI and endorectal ultrasound in the restaging and response assessment of rectal cancer following neoadjuvant therapy. Colorectal Dis 2015;17:748-61. [Crossref] [PubMed]
- Mutic S, Dempsey JF. The ViewRay system: magnetic resonance-guided and controlled radiotherapy. Semin Radiat Oncol 2014;24:196-9. [Crossref] [PubMed]
- Jaffray DA. Image-guided radiotherapy: from current concept to future perspectives. Nat Rev Clin Oncol 2012;9:688-99. [Crossref] [PubMed]
- Beets-Tan RG, Lambregts DM, Maas M, et al. Magnetic resonance imaging for the clinical management of rectal cancer patients: recommendations from the 2012 European Society of Gastrointestinal and Abdominal Radiology (ESGAR) consensus meeting. Eur Radiol 2013;23:2522-31. [Crossref] [PubMed]
- Padhani AR, Liu G, Koh DM, et al. Diffusion-weighted magnetic resonance imaging as a cancer biomarker: consensus and recommendations. Neoplasia 2009;11:102-25. [Crossref] [PubMed]
- Kwee TC, Takahara T, Ochiai R, et al. Diffusion-weighted whole-body imaging with background body signal suppression (DWIBS): features and potential applications in oncology. Eur Radiol 2008;18:1937-52. [Crossref] [PubMed]
- Ippolito D, Monguzzi L, Guerra L, et al. Response to neoadjuvant therapy in locally advanced rectal cancer: assessment with diffusion-weighted MR imaging and 18FDG PET/CT. Abdom Imaging 2012;37:1032-40. [Crossref] [PubMed]
- Jung SH, Heo SH, Kim JW, et al. Predicting response to neoadjuvant chemoradiation therapy in locally advanced rectal cancer: diffusion-weighted 3 Tesla MR imaging. J Magn Reson Imaging 2012;35:110-6. [Crossref] [PubMed]
- Monguzzi L, Ippolito D, Bernasconi DP, et al. Locally advanced rectal cancer: value of ADC mapping in prediction of tumor response to radiochemotherapy. Eur J Radiol 2013;82:234-40. [Crossref] [PubMed]
- Intven M, Reerink O, Philippens ME. Diffusion-weighted MRI in locally advanced rectal cancer: pathological response prediction after neo-adjuvant radiochemotherapy. Strahlenther Onkol 2013;189:117-22. [Crossref] [PubMed]
- Sun YS, Zhang XP, Tang L, et al. Locally advanced rectal carcinoma treated with preoperative chemotherapy and radiation therapy: preliminary analysis of diffusion-weighted MR imaging for early detection of tumor histopathologic downstaging. Radiology 2010;254:170-8. [Crossref] [PubMed]
- Cascini GL, Avallone A, Delrio P, et al. 18F-FDG PET is an early predictor of pathologic tumor response to preoperative radiochemotherapy in locally advanced rectal cancer. J Nucl Med 2006;47:1241-8. [PubMed]
- Joye I, Deroose CM, Vandecaveye V, et al. The role of diffusion-weighted MRI and (18)F-FDG PET/CT in the prediction of pathologic complete response after radiochemotherapy for rectal cancer: a systematic review. Radiother Oncol 2014;113:158-65. [Crossref] [PubMed]
- Janssen MH, Ollers MC, Riedl RG, et al. Accurate prediction of pathological rectal tumor response after two weeks of preoperative radiochemotherapy using (18)F-fluorodeoxyglucose-positron emission tomography-computed tomography imaging. Int J Radiat Oncol Biol Phys 2010;77:392-9. [Crossref] [PubMed]
- Ippolito D, Fior D, Trattenero C, et al. Combined value of apparent diffusion coefficient-standardized uptake value max in evaluation of post-treated locally advanced rectal cancer. World J Radiol 2015;7:509-20. [PubMed]
- Capirci C, Rubello D, Chierichetti F, et al. Restaging after neoadjuvant chemoradiotherapy for rectal adenocarcinoma: role of F18-FDG PET. Biomed Pharmacother 2004;58:451-7. [Crossref] [PubMed]
- de Geus-Oei LF, Vriens D, van Laarhoven HW, et al. Monitoring and predicting response to therapy with 18F-FDG PET in colorectal cancer: a systematic review. J Nucl Med 2009;50 Suppl 1:43S-54S. [Crossref] [PubMed]
- Kristiansen C, Loft A, Berthelsen AK, et al. PET/CT and histopathologic response to preoperative chemoradiation therapy in locally advanced rectal cancer. Dis Colon Rectum 2008;51:21-5. [Crossref] [PubMed]
- Ryu JK, Winter K, Michalski JM, et al. Interim report of toxicity from 3D conformal radiation therapy (3D-CRT) for prostate cancer on 3DOG/RTOG 9406, level III (79.2 Gy). Int J Radiat Oncol Biol Phys 2002;54:1036-46. [Crossref] [PubMed]
- Vestermark LW, Jensen HA, Pfeiffer P. High-dose radiotherapy (60 Gy) with oral UFT/folinic acid and escalating doses of oxaliplatin in patients with non-resectable locally advanced rectal cancer (LARC): a phase I trial. Acta Oncol 2012;51:311-7. [Crossref] [PubMed]
- Mohiuddin M, Regine WF, John WJ, et al. Preoperative chemoradiation in fixed distal rectal cancer: dose time factors for pathological complete response. Int J Radiat Oncol Biol Phys 2000;46:883-8. [Crossref] [PubMed]
- Mohiuddin M, Paulus R, Mitchell E, et al. Neoadjuvant chemoradiation for distal rectal cancer: 5-year updated results of a randomized phase 2 study of neoadjuvant combined modality chemoradiation for distal rectal cancer. Int J Radiat Oncol Biol Phys 2013;86:523-8. [Crossref] [PubMed]
- Pfeiffer P. High-dose radiotherapy and concurrent UFT plus l-leucovorin in locally advanced rectal cancer: a phase I trial. Acta Oncol 2005;44:224-9. [Crossref] [PubMed]
- Movsas B, Diratzouian H, Hanlon A, et al. Phase II trial of preoperative chemoradiation with a hyperfractionated radiation boost in locally advanced rectal cancer. Am J Clin Oncol 2006;29:435-41. [Crossref] [PubMed]
- Engineer R, Mohandas KM, Shukla PJ, et al. Escalated radiation dose alone vs. concurrent chemoradiation for locally advanced and unresectable rectal cancers: results from phase II randomized study. Int J Colorectal Dis 2013;28:959-66. [Crossref] [PubMed]
- Arbea L, Ramos LI, Martinez-Monge R, et al. Intensity-modulated radiation therapy (IMRT) vs. 3D conformal radiotherapy (3DCRT) in locally advanced rectal cancer (LARC): dosimetric comparison and clinical implications. Radiat Oncol 2010;5:17. [Crossref] [PubMed]
- Teoh S, Muirhead R. Rectal Radiotherapy--Intensity-modulated Radiotherapy Delivery, Delineation and Doses. Clin Oncol (R Coll Radiol) 2016;28:93-102. [Crossref] [PubMed]
- Alongi F, Fersino S, Mazzola R, et al. Radiation dose intensification in pre-operative chemo-radiotherapy for locally advanced rectal cancer. Clin Transl Oncol 2017;19:189-96. [Crossref] [PubMed]
- Ballonoff A, Kavanagh B, McCarter M, et al. Preoperative capecitabine and accelerated intensity-modulated radiotherapy in locally advanced rectal cancer: a phase II trial. Am J Clin Oncol 2008;31:264-70. [Crossref] [PubMed]
- Freedman GM, Meropol NJ, Sigurdson ER, et al. Phase I trial of preoperative hypofractionated intensity-modulated radiotherapy with incorporated boost and oral capecitabine in locally advanced rectal cancer. Int J Radiat Oncol Biol Phys 2007;67:1389-93. [Crossref] [PubMed]
- Zhu J, Liu F, Gu W, et al. Concomitant boost IMRT-based neoadjuvant chemoradiotherapy for clinical stage II/III rectal adenocarcinoma: results of a phase II study. Radiat Oncol 2014;9:70. [Crossref] [PubMed]
- Cubillo A, Hernando-Requejo O, Garcia-Garcia E, et al. A prospective pilot study of target-guided personalized chemotherapy with intensity-modulated radiotherapy in patients with early rectal cancer. Am J Clin Oncol 2014;37:117-21. [Crossref] [PubMed]
- Engels B, Platteaux N, Van den Begin R, et al. Preoperative intensity-modulated and image-guided radiotherapy with a simultaneous integrated boost in locally advanced rectal cancer: report on late toxicity and outcome. Radiother Oncol 2014;110:155-9. [Crossref] [PubMed]
- Hong TS, Moughan J, Garofalo MC, et al. NRG Oncology Radiation Therapy Oncology Group 0822: A Phase 2 Study of Preoperative Chemoradiation Therapy Using Intensity Modulated Radiation Therapy in Combination With Capecitabine and Oxaliplatin for Patients With Locally Advanced Rectal Cancer. Int J Radiat Oncol Biol Phys 2015;93:29-36. [Crossref] [PubMed]
- Patel SA, Wo JY, Hong TS. Advancing Techniques of Radiation Therapy for Rectal Cancer. Semin Radiat Oncol 2016;26:220-5. [Crossref] [PubMed]
- Vuong T, Belliveau PJ, Michel RP, et al. Conformal preoperative endorectal brachytherapy treatment for locally advanced rectal cancer: early results of a phase I/II study. Dis Colon Rectum 2002;45:1486-93; discussion 1493-5. [Crossref] [PubMed]
- Nout RA, Devic S, Niazi T, et al. CT-based adaptive high-dose-rate endorectal brachytherapy in the preoperative treatment of locally advanced rectal cancer: Technical and practical aspects. Brachytherapy 2016;15:477-84. [Crossref] [PubMed]
- Vuong T, Devic S. High-dose-rate pre-operative endorectal brachytherapy for patients with rectal cancer. J Contemp Brachytherapy 2015;7:183-8. [Crossref] [PubMed]
- Sun Myint A, Mukhopadhyay T, Ramani VS, et al. Can increasing the dose of radiation by HDR brachytherapy boost following pre operative chemoradiotherapy for advanced rectal cancer improve surgical outcomes? Colorectal Dis 2010;12 Suppl 2:30-6. [Crossref] [PubMed]
- Jakobsen A, Ploen J, Vuong T, et al. Dose-effect relationship in chemoradiotherapy for locally advanced rectal cancer: a randomized trial comparing two radiation doses. Int J Radiat Oncol Biol Phys 2012;84:949-54. [Crossref] [PubMed]
- Appelt AL, Vogelius IR, Ploen J, et al. Long-term results of a randomized trial in locally advanced rectal cancer: no benefit from adding a brachytherapy boost. Int J Radiat Oncol Biol Phys 2014;90:110-8. [Crossref] [PubMed]
- Appelt AL, Ploen J, Harling H, et al. High-dose chemoradiotherapy and watchful waiting for distal rectal cancer: a prospective observational study. Lancet Oncol 2015;16:919-27. [Crossref] [PubMed]
- Appelt AL, Sebag-Montefiore D. Technological advances in radiotherapy of rectal cancer: opportunities and challenges. Curr Opin Oncol 2016;28:353-8. [Crossref] [PubMed]
- Dirix P, Haustermans K, Vandecaveye V. The value of magnetic resonance imaging for radiotherapy planning. Semin Radiat Oncol 2014;24:151-9. [Crossref] [PubMed]
- Avallone A, Aloj L, Caraco C, et al. Early FDG PET response assessment of preoperative radiochemotherapy in locally advanced rectal cancer: correlation with long-term outcome. Eur J Nucl Med Mol Imaging 2012;39:1848-57. [Crossref] [PubMed]
- Leccisotti L, Gambacorta MA, de Waure C, et al. The predictive value of 18F-FDG PET/CT for assessing pathological response and survival in locally advanced rectal cancer after neoadjuvant radiochemotherapy. Eur J Nucl Med Mol Imaging 2015;42:657-66. [Crossref] [PubMed]
- Passoni P, Fiorino C, Slim N, et al. Feasibility of an adaptive strategy in preoperative radiochemotherapy for rectal cancer with image-guided tomotherapy: boosting the dose to the shrinking tumor. Int J Radiat Oncol Biol Phys 2013;87:67-72. [Crossref] [PubMed]
- Acharya S, Fischer-Valuck BW, Mazur TR, et al. Magnetic Resonance Image Guided Radiation Therapy for External Beam Accelerated Partial-Breast Irradiation: Evaluation of Delivered Dose and Intrafractional Cavity Motion. Int J Radiat Oncol Biol Phys 2016;96:785-92. [Crossref] [PubMed]
- Mooney K, Zhao T, Duan Y, et al. TU-AB-202-06: Quantitative Evaluation of Deformable Image Registration in MRI-Guided Adaptive Radiation Therapy. Med Phys 2016;43:3737. [Crossref]
- Zhongnan Hospital. Preoperative short-course radiotherapy with local boost versus conventional preoperative short-course radiotherapy for rectal cancer. Available online: (Not yet recruiting).http://ichgcp.net/clinical-trials-registry/NCT02498353
- Grupo de Investigación Clínica en Oncología Radioterapia. Dose-escalation trial of preoperative radiotherapy and concurrent chemotherapy in locally advanced rectal cancer. Available online: : NCT02964468 (This study is currently recruiting participants).https://clinicaltrials.gov/show/NCT02964468
- University of Brasilia. Radiation dose escalation in locally advanced rectal cancer (RaDE). In: ClinicalTrials.gov [Internet]. Bethesda (MD): National Library of Medicine (US). 2000- [cited 2017 Jan 26]. Available online: : NCT02603302 (This study is currently recruiting participants).https://clinicaltrials.gov/show/NCT02603302
- Chinese Academy of Medical Sciences. Tiral of using SIB-IMRT in preoperative radiotherapy for locally advanced rectum cancer. In: ClinicalTrials.gov [Internet]. Bethesda (MD): National Library of Medicine (US). 2000- [cited 2017 Jan 26]. Available online: : NCT02195141 (This study is currently recruiting participants).https://clinicaltrials.gov/show/NCT02195141
- McGill University Health Center. Patients with rectal cancer: a "wait-and-see" approach. In: ClinicalTrials.gov [Internet]. Bethesda (MD): National Library of Medicine (US). 2000- [cited 2017 Jan 26]. Available online: : NCT03001362 (This study is currently recruiting participants).https://clinicaltrials.gov/show/NCT03001362
- Centre Antoine Lacassagne. Safety of a boost (CXB or EBRT) in combination with neoadjuvant chemoradiotherapy for early rectal adenocarcinoma (OPERA). In: ClinicalTrials.gov [Internet]. Bethesda (MD): National Library of Medicine (US). 2000- [cited 2017 Jan 26]. Available online: : NCT02505750 (This study is currently recruiting participants).https://clinicaltrials.gov/show/NCT02505750