Proton beam therapy for gastrointestinal cancers: past, present, and future
Introduction
Each year in the United States approximately 156,000 patients are diagnosed with gastrointestinal (GI) cancers involving the foregut (1). Surgery remains the mainstay of curative therapy in most cases, although randomized trials have demonstrated improved survival with the addition of multimodality therapy compared to surgery alone for esophageal, gastric, and pancreatic malignancies (2-4). When radiotherapy (RT) is employed, it is typically administered preoperatively or postoperatively with concurrent radiosensitizing chemotherapy, or as a definitive modality.
RT for GI tumors is complicated by the need to deliver a sufficiently high dose to tumor while respecting the radiosensitivity of nearby critical organs (5). Nonetheless, the toxicity of chemoradiotherapy (CRT) for GI malignancies remains significant. For example, in the Intergroup 0116 trial, only 65% of gastric cancer patients randomized to CRT completed the planned therapy while 17% prematurely discontinued treatment. In aggregate, 9% of patients received <40 Gy (of a planned 45 Gy) and 1% died during therapy due to complications (6). Reducing acute toxicity and late adverse effects while maintaining or improving treatment efficacy is the central paradigm in the management of GI cancers and provides a strong rationale to consider novel radiation strategies.
Proton beam therapy (PBT) promises greater normal tissue sparing without compromising target coverage compared to X-ray-based techniques. PBT delivers ionizing radiation with charged particles compared to X-rays that have no mass or charge (7). As a result, the dose deposition is fundamentally different between the two radiation modalities. Along the path of a proton beam the dose deposited proximal to target is approximately 30% of the maximum dose with no “exit dose” being delivered distally, thus reducing the total integral dose to the patient by approximately 60% (8). Although still considered experimental by some, more than 118,000 patients have been treated with PBT worldwide through 2014, with approximately 15,400 in that year alone (9).
Recent data demonstrate that PBT for upper GI cancers may decrease acute toxicity and late complications and improve treatment compliance. Here we review the current evidence for PBT in this population and provide an overview of future research and utilization of in patients with foregut malignancies.
Esophageal cancer
Rationale for PBT
Over 18,000 cases of esophageal cancer are diagnosed each year in the United States, with relatively high mortality rates, although treatment outcomes over the years have greatly improved clinical outcomes. Neoadjuvant CRT followed by esophagectomy is the current standard of care for stages II–III esophageal cancer. However, treatment related morbidity and mortality is a relevant issue, primarily due to the exposure of intrathoracic cardiopulmonary organs that could dictate the incidence and severity of toxicities encountered during treatment and postoperatively. Several studies have demonstrated improved clinical outcomes with the use of more conformal X-ray techniques such as intensity modulated radiation therapy (IMRT) as compared to 3D-conformal RT (3DCRT) (10-12). The utilization of PBT could further enhance clinical benefits and have recently borne out to demonstrate further improvements in clinical outcomes.
Dosimetric benefit of PBT
Because of the central location of the esophagus, RT typically causes substantial dose deposition in both the heart and lungs. A number of studies have correlated toxicities and postoperative complications with dose delivered from X-ray therapy to these organs (13,14). Not only does IMRT improve upon the dose distribution to these organs compared to 3DCRT (15,16), the physics of PBT facilitates a dramatic reduction to both the heart and lungs in a number of planning studies and dosimetric comparisons in patients treated with either IMRT or PBT (Figure 1). This is seen for comparison of PBT to 3DCRT or IMRT (17-19). The greatest benefit seems to occur at the level of the heart, with greatest sparing of the anterior cardiac structures (20-22).
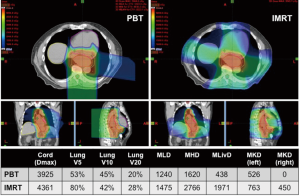
Clinical PBT outcomes
Clinical experiences have been mostly confined to single institutional experiences, with or without concurrent chemotherapy. Initial definitive PBT reports without chemotherapy were published from the University of Tsukuba, in which unresectable patients were treated with X-ray therapy to an initial median dose of 46 Gy with a boost to 80 Gy [relative biological effectiveness (RBE)], or with PBT alone to 79 Gy (RBE). In the most recent report including 51 patients [50 with squamous cell carcinoma (SCC)], the 5-year local control, progression-free survival and overall survival were 38%, 14%, and 21%, respectively (23). The same group reported their experience of adding concurrent cisplatin/5-fluorouracil (5-FU) and 60 Gy (RBE) PBT for 40 unresectable esophageal SCC patients (24). Acute grade 3 esophagitis was seen in 23% (9 of 40), which is about double the rate seen with PBT alone. Still, 2-year OS was 75% with local control was promising at 66%. More recently the outcomes of the first 62 esophageal cancer patients [47 with adenocarcinoma (AC)] treated with PBT at MD Anderson Cancer Center were reported (25). The median dose was 50.4 Gy (RBE) with concurrent 5-FU and taxane or a platinum-based chemotherapy. Nearly half (47%) had neoadjuvant therapy followed by surgery. The 3-year locoregional control, recurrence-free survival and overall survival were 57%, 52%, and 52%, respectively. Only about 10% of patients developed grade 3 esophagitis, and all other grade 3+ toxicities were less than 10%.
Postoperative complications are also commonly seen after surgery, mostly due to cardiac, GI, or pulmonary complications. There is a relationship of hospital volume and surgeon experience on the complication rates. Recently, treatment-related complications were assessed in the context of radiation modality in a multi-institutional study that assessed postoperative complications after neoadjuvant CRT (26). The study combined experiences within the same period of time from 2007–2013 treating 214 with 3DCRT, 255 with IMRT, and 111 with PBT. The study found RT modality to be significantly associated with the incidence of pulmonary, cardiac and wound healing complications. The utilization of advanced technologies including PBT and IMRT resulted in reduced complications compared to 3DCRT. PBT reduced pulmonary and wound complications. The length of hospitalization was significantly shortened for the PBT patients compared to those who received either IMRT or 3D (9.3 vs. 11.6 vs. 13.2 days, respectively, P<0.0001). This difference was not related to institutional bias or the implementation of enhanced recovery after surgery (ERAS) protocols, as the length of hospitalization was similar for patients who didn’t have any complications compared to those who developed any complications, regardless of the institution or the radiation modalities used. Interestingly, the 90-day postoperative mortality rate was nearly the same for 3DCRT and IMRT (4.2% and 4.3% respectively), but was lower for PBT (0.9%). For patients treated with definitive CRT, a single institutional experience from MD Anderson reported recently the long term clinical outcomes of patients treated with IMRT or PBT (27). Although the patients were well balanced between receiving IMRT or 3DCRT except for PBT patients being significantly older, clinical outcomes including overall survival, disease-free survival, and distant metastasis-free survival were significantly better for the PBT patients.
The growing body of clinical outcomes in esophageal cancer patients who received PBT collectively indicates that significant clinical benefits may be achieved when compared to X-ray therapy. These benefits appear to include at least reduced postoperative complications and reduced length of hospitalization after surgery although additional advantages may become apparent upon completion of ongoing clinical trials.
PBT clinical trials
While data from retrospective analyses are important, clinical trials are needed to generate prospective evidence supporting the role of PBT for esophageal cancer. Currently there are four clinical trials testing the role of PBT in esophageal cancer in the United States. Three are single arm studies: a pilot study at Mayo Clinic testing the utility of intensity modulated PBT (IMPT) (NCT02452021), a phase I dose escalation study with PBT and chemotherapy at University of Pennsylvania (NCT02213497), and a phase II study at Loma Linda University (NCT01684904). A randomized phase IIb trial comparing PBT versus IMRT is currently being conducted at MD Anderson under the NCI-U19 grant (NCT01512589). The co-primary endpoints are total toxicity burden (TTB), a composite toxicity weighted based on the grade of multiple toxicities quantified throughout the patients’ treatment experience (28), and PFS. A number of secondary endpoints include patient reported outcomes, quality of life, and cost effectiveness analysis. Looking towards the future there is intent to link the consortium of proton centers represented within NRG Oncology to conduct a definitive phase III trial comparing PBT vs. IMRT.
Gastric cancer
Rationale for PBT
Gastric carcinomas represent 9% of GI tumors with an estimated incidence of 28,000 cases per year in the United States. It is the sixth most common neoplasm in developed countries, but is more prevalent in developing countries (1). Surgical resection is the standard of care for definitive gastric cancer management, although most patients receive multimodality therapy, which may include postoperative chemotherapy, perioperative chemotherapy, or trimodality treatment. Dosimetric studies demonstrated significant improvements in target volume coverage and organ at risk sparing with 3DCRT and IMRT, compared to older 2D techniques (29,30).
Dosimetric benefit of PBT
PBT can both further improve normal organ sparing and significantly reduce the total integral radiation dose compared to IMRT; this may reduce late effects and induction of secondary malignancies (31). In a retrospective analysis, Dionisi et al. created six-field IMRT and double passive scatter/uniform scanning PBT plans (2–3 fields per day) for 13 patients who received adjuvant RT for gastric carcinoma to 45–54 Gy (RBE) at 1.8 Gy per fraction (32). The authors demonstrated that PBT significantly reduced the low-intermediate dose delivered (P<0.05 for all comparisons) to the small bowel (V15: 82 vs. 133 mL), liver (mean 11.9 vs. 14.4 Gy), left/right kidney (mean: 5 and 0.9 vs. 7.8 and 3.1 Gy) and heart (mean: 7.4 vs. 9.5 Gy). PBT also significantly reduced the total integral radiation dose delivered outside of the target volume. In this series, four patients were treated with PBT and the authors reported robust target volume coverage within ±2% on all verification scans.
Clinical PBT outcomes
Although there is potential for PBT to reduce toxicities in gastric cancer patients (13) and potentially allow for safe dose-escalation to improve local control (33), there are very limited published clinical outcomes. Despite the potential benefits, PBT has not yet been widely adopted for the treatment of gastric cancer. Further clinical data with rigorous follow-up are needed to enumerate the benefits of PBT compared to the best X-ray literature. There are not clinical trials for gastric cancer currently recruiting patients, to the best of our knowledge, although at least one is planned at Massachusetts General Hospital (NCT03279237).
Liver cancer
Rationale for PBT
Historically, the use of X-ray therapy for liver tumors has been limited by the inability to deliver curative RT dose without excessive risk of hepatotoxicity, especially in patients with underlying liver disease. The risk of radiation-induced liver disease (RILD) is strongly correlated with mean liver dose and pre-treatment liver function (34). Additionally, there is a dose-response relationship for risk of toxicity to the stomach, bowel, and kidneys (34-36).
Dosimetric benefit of PBT
Planning studies in patients with liver tumors demonstrate that PBT significantly reduces mean liver dose by an average of 30–55% compared to IMRT (37,38). Additionally, dose received by the kidneys, stomach, intestines, and heart were significantly reduced with PBT (38). The favorable dose distribution achieved with PBT (Figure 2) may result in a lower rate of acute and late toxicities and/or a higher rate of tumor control because of the ability to safely administer higher doses.
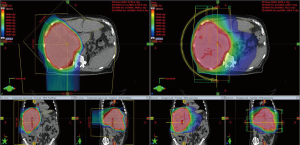
Clinical PBT data for hepatocellular carcinoma (HCC)
Several retrospective and single arm prospective studies have reported favorable outcomes with high-dose, conformal PBT in the treatment of unresectable or locally recurrent HCC. In the 1990s, investigators from the University of Tsukuba reported on initial studies demonstrating the feasibility and early safety and efficacy data for the use of PBT for localized HCC (39,40). A more recent publication from the University of Tsukuba reports outcomes of 266 patients with unresectable/recurrent HCC who were treated with high-dose conformal PBT (41). Most patients (76%) had Child-Pugh class A cirrhosis. Patients were treated with 1 of 3 treatment regimens [66 Gy (RBE) in 10 fractions, 72.6 Gy (RBE) in 22 fractions, or 77 Gy (RBE) in 35 fractions], depending on tumor location and proximity to GI luminal structures. Treatment was well tolerated with only 3% reported to experience severe (grade 3 or higher) treatment-related adverse effects. Rates of tumor local control and overall survival at 5 years were 81% and 48%, respectively. Additional studies have reported the safety and efficacy of PBT for patients with very large tumors (≥10 cm) (42) and/or portal vein tumor thrombus (43); these patients typically are not candidates for any other locoregional therapies. Several prospective phase II trials assessing PBT for HCC have now been published (Table 1) (44-48). A recently published systematic review and meta-analysis demonstrated significant improvements in overall survival, progression-free survival, local control, and high-grade acute and late toxicity with use of charged particle therapy versus conventional X-ray therapy (49). An ongoing phase III randomized trial (NRG Oncology Trial GI-003, NCT03186898) is comparing proton vs. X-ray therapy for unresectable or locally recurrent HCC.
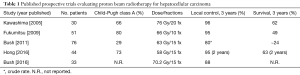
Full table
Clinical PBT data for intrahepatic cholangiocarcinoma
Several recent series have reported outcomes with the use of high-dose conformal PBT for unresectable intrahepatic cholangiocarcinoma (CCA) (47,50,51). In a prospective phase II study, 37 patients with intrahepatic CCA were treated with a PBT to a median dose of 58.05 Gy (RBE) in 15 fractions (47). Severe treatment-related toxicity was observed in 8% of patients. Rates of tumor local control and overall survival at 2 years were promising at 94% and 47%.
Clinical PBT data for liver metastases
There are limited reports of the use of PBT for patients with liver metastases (52-56). In a retrospective series from the University of Tsukuba, 140 patients received PBT most commonly 72.6 Gy (RBE) in 22 fractions. The 5-year local tumor control rate was 53%. Higher biologically equivalent doses are likely needed to control liver metastases; therefore, proton stereotactic body RT (SBRT) regimens deserve further investigation. Since some patients with liver metastasis may have prolonged survival and an excellent prognosis, there is also rationale to consider PBT on the basis of reduced radiation-induced secondary cancers using proton-based SBRT of liver metastases (54).
Pancreatic and extrahepatic biliary tumors
Rationale for PBT
Advanced X-ray techniques such as IMRT and SBRT allow for delivery of highly conformal doses to targets, but the physical properties of the X-rays ultimately limit the ability to minimize dose to surrounding normal tissues. Due to this inherent property of X-ray therapy the delivery of systemically effective multi-agent chemotherapy regimens concurrently with definitive doses of conventional radiation has been largely hampered by the GI toxicity of such regimens (57-59).
Dosimetric benefit of PBT
Multiple dosimetric studies have demonstrated decreased doses to normal tissues using PBT compared to IMRT for both unresected and resected pancreatic cancers (Figure 3). Investigators at the University of Pennsylvania demonstrated that when treating unresected tumors of the pancreatic head both double scattering and pencil beam scanning PBT techniques decreased low dose to the stomach, duodenum and small bowel while slightly increasing the mid to high doses ranges to the duodenum and stomach (60). The same group also compared the dosimetric differences in resected pancreatic tumors and found that PBT plans significantly reduced clinically relevant doses to the left kidney, stomach and spinal cord compared to X-ray therapy plans while pencil beam scanning PBT reduced doses to the right kidney, liver and total and small bowels compared to X-ray plans and double scattering PBT plans (61). Similarly, for patients being treated postoperatively Nichols et al. found significant reductions in small bowel and stomach V20, and right kidney V18 with PBT compared to IMRT (62). Zurlo et al. compared dose-escalated pencil beam scanning PBT plans [50 Gy (RBE) with 20 Gy (RBE) boost] to IMRT plans for two cases of pancreatic cancer and two cases of extrahepatic biliary cancer and found significantly reduced risk of normal tissue complications in the liver, kidneys or bowel with the PBT plans (63). Dose to the stomach and bowel in the intermediate dose range have been found to correlate with nausea and vomiting during CRT for pancreatic cancer (64). The results of these studies suggest that the advantageous dose distribution properties of PBT may result in decreased acute and late toxicity compared to X-ray-based therapies and potentially enable the delivery of higher doses of radiation and/or more systemically effective chemotherapy regimens concurrently with RT.
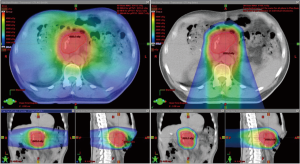
Clinical PBT data
Though clinical data on PBT for pancreatic cancer is limited, early results are promising. Hong et al. conducted a phase I/II study evaluating a short course of PBT to 25 Gy (RBE) in 5 fractions with concurrent capecitabine for resectable pancreatic cancer. The regimen demonstrated an acceptable low grade 3 toxicity rate of 4.1%, locoregional failure rate of 16.2% and median overall survival of 17 months (65). Outcomes of resectable (n=5), borderline resectable (n=5) and locally advanced (n=12) pancreatic cancer patients treated with conventionally fractionated PBT [50.4–59.4 Gy (RBE) in 1.8 Gy (RBE) per fraction] with concurrent chemotherapy were reported by investigators at the University of Florida. There were no instances of grade 3 acute or late toxicity and concurrent chemotherapy was well tolerated with 99% of prescribed doses delivered. In addition, investigators noted that grade 2 toxicity was eliminated in patients that did not receive anterior or left lateral beams (66). Fifty patients with locally advanced pancreatic cancer were treated on a phase I/II protocol in Japan to three dose levels [50 or 67.5 Gy (RBE) in 25 fractions or 70.2 Gy (RBE) in 26 fractions] with concurrent and adjuvant gemcitabine. Grade 3 or greater gastric ulcer or hemorrhage was reported in 10% of patients, including one death from gastric hemorrhage, all treated in the two dose-escalation cohorts. At a median follow-up of 13 months, 1-year freedom from local progression, progression-free survival, and overall survival were 82%, 64%, and 77%, respectively (67). Post-treatment endoscopic examinations revealed 45 of 91 (49.5%) patients receiving PBT and concurrent gemcitabine developed stomach or duodenal ulcers, however only 10% of patients developed grade 3 or greater clinically symptomatic ulcers. No patient, tumor or treatment factors were found to predict for radiation-induced ulcers (68). Additional studies are needed to further elucidate the benefits of PBT for pancreatic cancer.
Clinical data on PBT for extrahepatic biliary tumors is very limited. The only published retrospective series included extrahepatic biliary tumors along with intrahepatic tumors when reporting outcomes. Makita et al. treated 28 patients with CCA including 12 with intrahepatic tumors, 3 with extrahepatic cholangiocarcinoma, 3 with gallbladder cancer and 10 with a local or lymph node recurrence after surgery. Median PBT dose was 68.2 Gy (RBE) [range, 50.6 to 80 Gy (RBE) in 2.0 to 3.2 Gy (RBE) per fraction]. Fifteen patients received adjuvant chemotherapy and the 1-year local control, progression-free survival, and overall survival were 67.7%, 29.5% and 49.0%, respectively. A biologically effective dose (α/β =10) exceeding 70 Gy was associated with a higher rate of local control while tumor size and performance status were associated with OS. Acute grade 3 toxicity consisted of cholangitis in one patient. Late grade 3 toxicities consisted of cholangitis (n=2), bile duct stenosis (n=1), duodenal ulcer or hemorrhage (n=3), and duodenal stenosis (n=1). There was no acute or late grade 4 or 5 toxicity (51).
Conclusions
Given the accruing data showing a strong relationship between clinical outcomes and low dose received by organs at risk, there is a strong rationale to consider PBT for patients with cancers of the foregut. While not all patients likely benefit from PBT, mounting retrospective data indicate that ongoing and future clinical trials may demonstrate that PBT provides clinically meaningful benefit for a subset of patients with GI cancers.
Acknowledgements
None.
Footnote
Conflicts of Interest: The authors have no conflicts of interest to declare.
References
- Siegel RL, Miller KD, Jemal A. Cancer Statistics, 2017. CA Cancer J Clin 2017;67:7-30. [Crossref] [PubMed]
- Macdonald JS, Smalley SR, Benedetti J, et al. Chemoradiotherapy after surgery compared with surgery alone for adenocarcinoma of the stomach or gastroesophageal junction. N Engl J Med 2001;345:725-30. [Crossref] [PubMed]
- Cunningham D, Allum WH, Stenning SP, et al. Perioperative chemotherapy versus surgery alone for resectable gastroesophageal cancer. N Engl J Med 2006;355:11-20. [Crossref] [PubMed]
- Oettle H, Post S, Neuhaus P, et al. Adjuvant chemotherapy with gemcitabine vs observation in patients undergoing curative-intent resection of pancreatic cancer: a randomized controlled trial. JAMA 2007;297:267-77. [Crossref] [PubMed]
- Bentzen SM, Constine LS, Deasy JO, et al. Quantitative Analyses of Normal Tissue Effects in the Clinic (QUANTEC): an introduction to the scientific issues. Int J Radiat Oncol Biol Phys 2010;76:S3-9. [Crossref] [PubMed]
- Smalley SR, Benedetti JK, Haller DG, et al. Updated analysis of SWOG-directed intergroup study 0116: a phase III trial of adjuvant radiochemotherapy versus observation after curative gastric cancer resection. J Clin Oncol 2012;30:2327-33. [Crossref] [PubMed]
- Jones B, Dale RG, Carabe-Fernandez A. Charged particle therapy for cancer: the inheritance of the Cavendish scientists? Appl Radiat Isot 2009;67:371-7. [Crossref] [PubMed]
- Mitin T, Zietman AL. Promise and pitfalls of heavy-particle therapy. J Clin Oncol 2014;32:2855-63. [Crossref] [PubMed]
- Jermann M. Particle therapy statistics in 2014. Int J Part Ther 2015;2:50-4. [Crossref]
- Lin SH, Wang L, Myles B, et al. Propensity score-based comparison of long-term outcomes with 3-dimensional conformal radiotherapy vs intensity-modulated radiotherapy for esophageal cancer. Int J Radiat Oncol Biol Phys 2012;84:1078-85. [Crossref] [PubMed]
- Freilich J, Hoffe SE, Almhanna K, et al. Comparative outcomes for three-dimensional conformal versus intensity-modulated radiation therapy for esophageal cancer. Dis Esophagus 2015;28:352-7. [Crossref] [PubMed]
- Lin SH, Zhang N, Godby J, et al. Radiation modality use and cardiopulmonary mortality risk in elderly patients with esophageal cancer. Cancer 2016;122:917-28. [Crossref] [PubMed]
- Wang J, Wei C, Tucker SL, et al. Predictors of postoperative complications after trimodality therapy for esophageal cancer. Int J Radiat Oncol Biol Phys 2013;86:885-91. [Crossref] [PubMed]
- Hatakenaka M, Yonezawa M, Nonoshita T, et al. Acute cardiac impairment associated with concurrent chemoradiotherapy for esophageal cancer: magnetic resonance evaluation. Int J Radiat Oncol Biol Phys 2012;83:e67-73. [Crossref] [PubMed]
- Nutting CM, Bedford JL, Cosgrove VP, et al. A comparison of conformal and intensity-modulated techniques for oesophageal radiotherapy. Radiother Oncol 2001;61:157-63. [Crossref] [PubMed]
- Fenkell L, Kaminsky I, Breen S, et al. Dosimetric comparison of IMRT vs. 3D conformal radiotherapy in the treatment of cancer of the cervical esophagus. Radiother Oncol 2008;89:287-91. [Crossref] [PubMed]
- Wang J, Palmer M, Bilton SD, et al. Comparing Proton Beam to Intensity Modulated Radiation Therapy Planning in Esophageal Cancer. Int J Particle Ther 2015;1:866-77. [Crossref]
- Zhang X. Four-Dimensional Computed Tomography-Based Treatment Planning for Intensity-Modulated Radiation Therapy and Proton Therapy for Distal Esophageal Cancer. Int J Radiat Oncol Biol Phys 2008;72:278-87. [Crossref] [PubMed]
- Welsh J, Gomez D, Palmer MB, et al. Intensity-Modulated Proton Therapy Further Reduces Normal Tissue Exposure During Definitive Therapy for Locally Advanced Distal Esophageal Tumors: A Dosimetric Study. Int J Radiat Oncol Biol Phys 2011;81:1336-42. [Crossref] [PubMed]
- Grosshans D, Boehling NS, Palmer M, et al. Improving cardiac dosimetry: Alternative beam arrangements for intensity modulated radiation therapy planning in patients with carcinoma of the distal esophagus. Pract Radiat Oncol 2012;2:41-5. [Crossref] [PubMed]
- Kole TP, Aghayere O, Kwah J, et al. Comparison of heart and coronary artery doses associated with intensity-modulated radiotherapy versus three-dimensional conformal radiotherapy for distal esophageal cancer. Int J Radiat Oncol Biol Phys 2012;83:1580-6. [Crossref] [PubMed]
- Shiraishi Y, Xu C, Yang J, et al. Dosimetric comparison to the heart and cardiac substructure in a large cohort of esophageal cancer patients treated with proton beam therapy or Intensity-modulated radiation therapy. Radiother Oncol 2017;125:48-54. [Crossref] [PubMed]
- Mizumoto M, Sugahara S, Nakayama H, et al. Clinical results of proton-beam therapy for locoregionally advanced esophageal cancer. Strahlenther Onkol 2010;186:482-8. [Crossref] [PubMed]
- Ishikawa H, Hashimoto T, Moriwaki T, et al. Proton beam therapy combined with concurrent chemotherapy for esophageal cancer. Anticancer Res 2015;35:1757-62. [PubMed]
- Lin SH, Komaki R, Liao Z, et al. Proton beam therapy and concurrent chemotherapy for esophageal cancer. Int J Radiat Oncol Biol Phys 2012;83:e345-51. [Crossref] [PubMed]
- Lin SH, Merrell KW, Shen J, et al. Multi-institutional analysis of radiation modality use and postoperative outcomes of neoadjuvant chemoradiation for esophageal cancer. Radiother Oncol 2017;123:376-81. [Crossref] [PubMed]
- Xi M, Cai X, Liao Z, et al. Comparative Outcomes After Definitive Chemoradiotherapy Using Proton Beam Therapy Versus Intensity Modulated Radiation Therapy for Esophageal Cancer: A Retrospective, Single-Institutional Analysis. Int J Radiat Oncol Biol Phys 2017;99:667-76. [Crossref] [PubMed]
- Hobbs BP, Thall PF, Lin SH. Bayesian Group Sequential Clinical Trial Design using Total Toxicity Burden and Progression-Free Survival. J R Stat Soc Ser C Appl Stat 2016;65:273-97. [Crossref] [PubMed]
- Lohr F, Dobler B, Mai S, et al. Optimization of dose distributions for adjuvant locoregional radiotherapy of gastric cancer by IMRT. Strahlenther Onkol 2003;179:557-63. [Crossref] [PubMed]
- Milano MT, Garofalo MC, Chmura SJ, et al. Intensity-modulated radiation therapy in the treatment of gastric cancer: early clinical outcome and dosimetric comparison with conventional techniques. Br J Radiol 2006;79:497-503. [Crossref] [PubMed]
- Dahele M, Skinner M, Schultz B, et al. Adjuvant radiotherapy for gastric cancer: A dosimetric comparison of 3-dimensional conformal radiotherapy, tomotherapy and conventional intensity modulated radiotherapy treatment plans. Med Dosim 2010;35:115-21. [Crossref] [PubMed]
- Dionisi F, Avery S, Lukens JN, et al. Proton therapy in adjuvant treatment of gastric cancer: planning comparison with advanced x-ray therapy and feasibility report. Acta Oncol 2014;53:1312-20. [Crossref] [PubMed]
- Shibuya S, Takase Y, Aoyagi H, et al. Definitive proton beam radiation therapy for inoperable gastric cancer: a report of two cases. Radiat Med 1991;9:35-40. [PubMed]
- Pan CC, Kavanagh BD, Dawson LA, et al. Radiation-associated liver injury. Int J Radiat Oncol Biol Phys 2010;76:S94-100. [Crossref] [PubMed]
- Kavanagh BD, Pan CC, Dawson LA, et al. Radiation dose-volume effects in the stomach and small bowel. Int J Radiat Oncol Biol Phys 2010;76:S101-7. [Crossref] [PubMed]
- Dawson LA, Kavanagh BD, Paulino AC, et al. Radiation-associated kidney injury. Int J Radiat Oncol Biol Phys 2010;76:S108-15. [Crossref] [PubMed]
- Petersen JB, Lassen Y, Hansen AT, et al. Normal liver tissue sparing by intensity-modulated proton stereotactic body radiotherapy for solitary liver tumours. Acta Oncologica 2011;50:823-8. [Crossref] [PubMed]
- Wang X, Krishnan S, Zhang X, et al. Proton radiotherapy for liver tumors: dosimetric advantages over photon plans. Med Dosim 2008;33:259-67. [Crossref] [PubMed]
- Tsujii H, Tsuji H, Inada T, et al. Clinical results of fractionated proton therapy. Int J Radiat Oncol Biol Phys 1993;25:49-60. [Crossref] [PubMed]
- Matsuzaki Y, Osuga T, Saito Y, et al. A new, effective, and safe therapeutic option using proton irradiation for hepatocellular carcinoma. Gastroenterology 1994;106:1032-41. [Crossref] [PubMed]
- Mizumoto M, Okumura T, Hashimoto T, et al. Proton beam therapy for hepatocellular carcinoma: a comparison of three treatment protocols. Int J Radiat Oncol Biol Phys 2011;81:1039-45. [Crossref] [PubMed]
- Sugahara S, Oshiro Y, Nakayama H, et al. Proton beam therapy for large hepatocellular carcinoma. Int J Radiat Oncol Biol Phys 2010;76:460-6. [Crossref] [PubMed]
- Sugahara S, Nakayama H, Fukuda K, et al. Proton-beam therapy for hepatocellular carcinoma associated with portal vein tumor thrombosis. Strahlenther Onkol 2009;185:782-8. [Crossref] [PubMed]
- Kawashima M, Furuse J, Nishio T, et al. Phase II study of radiotherapy employing proton beam for hepatocellular carcinoma. J Clin Oncol 2005;23:1839-46. [Crossref] [PubMed]
- Fukumitsu N, Sugahara S, Nakayama H, et al. A prospective study of hypofractionated proton beam therapy for patients with hepatocellular carcinoma. Int J Radiat Oncol Biol Phys 2009;74:831-6. [Crossref] [PubMed]
- Bush DA, Kayali Z, Grove R, et al. The safety and efficacy of high-dose proton beam radiotherapy for hepatocellular carcinoma: a phase 2 prospective trial. Cancer 2011;117:3053-9. [Crossref] [PubMed]
- Hong TS, Wo JY, Yeap BY, et al. Multi-Institutional Phase II Study of High-Dose Hypofractionated Proton Beam Therapy in Patients With Localized, Unresectable Hepatocellular Carcinoma and Intrahepatic Cholangiocarcinoma. J Clin Oncol 2016;34:460-8. [Crossref] [PubMed]
- Bush DA, Smith JC, Slater JD, et al. Randomized Clinical Trial Comparing Proton Beam Radiation Therapy with Transarterial Chemoembolization for Hepatocellular Carcinoma: Results of an Interim Analysis. Int J Radiat Oncol Biol Phys 2016;95:477-82. [Crossref] [PubMed]
- Qi WX, Fu S, Zhang Q, et al. Charged particle therapy versus photon therapy for patients with hepatocellular carcinoma: A systematic review and meta-analysis. Radiother Oncol 2015;114:289-95. [Crossref] [PubMed]
- Ohkawa A, Mizumoto M, Ishikawa H, et al. Proton beam therapy for unresectable intrahepatic cholangiocarcinoma. J Gastroenterol Hepatol 2015;30:957-63. [Crossref] [PubMed]
- Makita C, Nakamura T, Takada A, et al. Clinical outcomes and toxicity of proton beam therapy for advanced cholangiocarcinoma. Radiat Oncol 2014;9:26. [Crossref] [PubMed]
- Fukumitsu N, Okumura T, Takizawa D, et al. Proton beam therapy for metastatic liver tumors. Radiother Oncol 2015;117:322-7. [Crossref] [PubMed]
- Fukumitsu N, Okumura T, Numajiri H, et al. Follow-up study of liver metastasis from breast cancer treated by proton beam therapy. Mol Clin Oncol 2017;7:56-60. [Crossref] [PubMed]
- Mondlane G, Gubanski M, Lind PA, et al. Comparative study of the calculated risk of radiation-induced cancer after photon- and proton-beam based radiosurgery of liver metastases. Phys Med 2017;42:263-70. [Crossref] [PubMed]
- Colbert LE, Cloyd JM, Koay EJ, et al. Proton beam radiation as salvage therapy for bilateral colorectal liver metastases not amenable to second-stage hepatectomy. Surgery 2017;161:1543-8. [Crossref] [PubMed]
- Fukumitsu N, Okumura T, Takizawa D, et al. Proton beam therapy for liver metastases from gastric cancer. J Radiat Res 2017;58:357-62. [PubMed]
- Wolff RA, Evans DB, Gravel DM, et al. Phase I trial of gemcitabine combined with radiation for the treatment of locally advanced pancreatic adenocarcinoma. Clin Cancer Res 2001;7:2246-53. [PubMed]
- Talamonti MS, Catalano PJ, Vaughn DJ, et al. Eastern Cooperative Oncology Group Phase I trial of protracted venous infusion fluorouracil plus weekly gemcitabine with concurrent radiation therapy in patients with locally advanced pancreas cancer: a regimen with unexpected early toxicity. J Clin Oncol 2000;18:3384-9. [Crossref] [PubMed]
- Blackstock AW, Bernard SA, Richards F, et al. Phase I trial of twice-weekly gemcitabine and concurrent radiation in patients with advanced pancreatic cancer. J Clin Oncol 1999;17:2208-12. [Crossref] [PubMed]
- Thompson RF, Mayekar SU, Zhai H, et al. A dosimetric comparison of proton and photon therapy in unresectable cancers of the head of pancreas. Med Phys 2014;41. [Crossref] [PubMed]
- Ding X, Dionisi F, Tang S, et al. A comprehensive dosimetric study of pancreatic cancer treatment using three-dimensional conformal radiation therapy (3DCRT), intensity-modulated radiation therapy (IMRT), volumetric-modulated radiation therapy (VMAT), and passive-scattering and modulated-scanning proton therapy (PT). Med Dosim 2014;39:139-45. [Crossref] [PubMed]
- Nichols RC Jr, Huh SN, Prado KL, et al. Protons offer reduced normal-tissue exposure for patients receiving postoperative radiotherapy for resected pancreatic head cancer. Int J Radiat Oncol Biol Phys 2012;83:158-63. [Crossref] [PubMed]
- Zurlo A, Lomax A, Hoess A, et al. The role of proton therapy in the treatment of large irradiation volumes: a comparative planning study of pancreatic and biliary tumors. Int J Radiat Oncol Biol Phys 2000;48:277-88. [Crossref] [PubMed]
- Radiation-induced emesis: a prospective observational multicenter Italian trial. The Italian Group for Antiemetic Research in Radiotherapy. Int J Radiat Oncol Biol Phys 1999;44:619-25. [Crossref] [PubMed]
- Hong TS, Ryan DP, Borger DR, et al. A phase 1/2 and biomarker study of preoperative short course chemoradiation with proton beam therapy and capecitabine followed by early surgery for resectable pancreatic ductal adenocarcinoma. Int J Radiat Oncol Biol Phys 2014;89:830-8. [Crossref] [PubMed]
- Nichols RC Jr, George TJ, Zaiden RA Jr, et al. Proton therapy with concomitant capecitabine for pancreatic and ampullary cancers is associated with a low incidence of gastrointestinal toxicity. Acta Oncol 2013;52:498-505. [Crossref] [PubMed]
- Terashima K, Demizu Y, Hashimoto N, et al. A phase I/II study of gemcitabine-concurrent proton radiotherapy for locally advanced pancreatic cancer without distant metastasis. Radiother Oncol 2012;103:25-31. [Crossref] [PubMed]
- Takatori K, Terashima K, Yoshida R, et al. Upper gastrointestinal complications associated with gemcitabine-concurrent proton radiotherapy for inoperable pancreatic cancer. J Gastroenterol 2014;49:1074-80. [Crossref] [PubMed]