Proton beam radiotherapy for pancreas cancer
Introduction
Pancreatic adenocarcinoma remains a challenging malignancy to treat with a grim prognosis. In 2018, there were an estimated 55,000 new cases in the United States and nearly 500,000 worldwide (1,2). The annual number of deaths from pancreatic carcinoma nearly equals the annual incidence (2,3). Nevertheless, there has been incremental progress in treatment outcomes over the past several decades. The combination of surgery, chemotherapy, and radiotherapy has led to advances in our understanding of the disease and has improved the outcomes for patients with pancreatic carcinoma. During the past 4 decades, the role of radiotherapy in the treatment paradigm for pancreatic carcinoma has been varied and controversial. Early randomized trials of the Gastrointestinal Tumor Study Group (GITSG) showed overall survival benefits with combined chemotherapy and radiotherapy (CRT) for locally advanced (unresectable) pancreatic cancer (LAPC) compared to either chemotherapy or radiotherapy alone (4,5). Investigators of the Eastern Cooperative Oncology Group study ECOG E4201 compared induction chemotherapy with gemcitabine to CRT with gemcitabine followed by consolidation with gemcitabine for locally advanced pancreatic cancer. The results indicated a 2-month median survival improvement for CRT (6). In contrast, LAP07 was a large, international contemporary study evaluating chemotherapy (with gemcitabine) alone versus induction chemotherapy (with gemcitabine) followed by CRT (with capecitabine) for locally advanced pancreatic carcinoma (7). While there was a strong trend towards an improvement in progression-free survival in the CRT arm, there was no difference in overall survival (7). Trials evaluating the survival benefit for postoperative radiotherapy have also come with mixed results. Investigators of the GITSG 91-73 trial showed an overall survival benefit for CRT in the postoperative setting (8). However, subsequent trials in Europe demonstrated conflicting outcomes. The results of EORTC 40891 showed a strong trend toward improved survival with postoperative CRT, although it did not reach statistical significance (9). Investigators of ESPAC-1 demonstrated a survival benefit to postoperative chemotherapy but no benefit to CRT (10). Despite early trials showing a benefit to CRT for locally advanced and resectable pancreatic cancer, these recent studies have cast uncertainty on the survival benefits of radiotherapy. In the postoperative setting, the data have been more conclusive in demonstrating a survival benefit with adjuvant chemotherapy compared to no adjuvant therapy (10,11). Meanwhile, advances in chemotherapy regimens have led to improved tumor responses and survival outcomes (12-14). It is expected that with improved systemic control as a result of more effective chemotherapies, the local-regional control benefits of CRT will have a greater impact on overall patient outcomes. Furthermore, with continued improvements in the delivery techniques and modalities of radiotherapy, treatment is expected to become more tolerable and amenable to intensification to improve disease control. Proton radiotherapy is one such modality that enables significantly improved radiation dose distributions and is being explored as a means to improve treatment tolerance and enable treatment intensification in an effort to improve outcomes. Herein, we will review the theoretical foundation and clinical evidence in support of proton radiotherapy as part of the management of pancreatic carcinoma as well as discuss ongoing and future studies.
Rationale for radiotherapy
The high risk of local-regional recurrence of pancreatic carcinoma forms the rationale for CRT as part of its management. Resection margin status and lymph node involvement are known risk factors for local-regional recurrence (15,16). Contemporary studies report positive postoperative margins rates of 35–60% and lymph node metastases are present in ~75–80% (12,16). Following surgery alone, ~40–70% of patients experience a local-regional recurrence (17,18). Importantly, the pattern of failure for pancreatic cancer varies depending on which treatment modalities are utilized. Patients receiving chemotherapy alone have significantly higher rates of local-regional failure, while those receiving CRT have reduced rates of local-regional recurrence, but a higher incidence of distant failures (7,16). With adjuvant chemotherapy, there remains a nearly 50% risk of a local-regional recurrence (12,19). In contrast, contemporary studies show rates of local-regional recurrence following CRT for both operable and inoperable cases of ~30%, whereas metastatic progression is ~70% (7,20). Combining optimal selection and timing of treatment modalities will likely improve outcomes for this malignancy. As more efficacious chemotherapy regimens achieve improved systemic disease control, the role of CRT for local-regional control will become paramount. The recently reported Dutch PREOPANC-1 Trial showed a survival benefit with preoperative CRT and adjuvant chemotherapy (gemcitabine) compared to surgery and adjuvant chemotherapy for resectable disease (21). More effective chemotherapy regimens than those in the PREOPANC trial are already improving survival outcomes (22). These systemic therapy advances combined with other improvements in local-regional control will ultimately be required to further improve disease-free and overall survival outcomes.
Radiotherapy advances
With advances in radiation treatment planning and delivery techniques, CRT for pancreatic cancer has become significantly more tolerable. Intensity-modulated radiotherapy (IMRT) was initially used for the treatment of pancreatic cancer in the early 2000s. IMRT enables highly conformal radiation dose delivery with an improved ability to avoid nearby organs at risk (small bowel/duodenum, kidneys, etc.) compared to 3-dimensional conformal radiotherapy (3DCRT). Early series confirmed the safety and efficacy of IMRT in both the adjuvant and definitive settings (23,24). Subsequent series have demonstrated reduced acute and late gastrointestinal (GI) toxicity using IMRT compared to 3DCRT in the adjuvant and postoperative settings (25,26). A large systematic review including over 1,000 patients compared treatment-related toxicity between 3DCRT and IMRT. When comparing 3DCRT and IMRT, the investigators of the study observed ≥ grade 3 nausea and vomiting in 13.4% and 7.8%, and ≥ grade 3 diarrhea in 11.6% versus 2.0%, respectively (27). With reduced toxicity through IMRT, investigators have explored radiation dose intensification to achieve ablative doses that may improve local control and disease outcomes (28-30). RTOG 1201 was a phase II randomized trial comparing standard dose or dose-escalated radiotherapy with concurrent gemcitabine/nab-paclitaxel to gemcitabine/nab-paclitaxel alone for locally advanced pancreatic cancer. The study was closed early due to slow accrual.
Rationale for proton radiotherapy
The use of particle radiotherapy utilizing protons has been pursued in an effort to further improve the therapeutic ratio of preoperative, adjuvant, and definitive CRT. In proton radiotherapy, the nucleus of a hydrogen atom is accelerated to high speeds (i.e., high energy) by a particle accelerator such as a synchrotron or cyclotron. In contrast to photons (X-rays), protons possess both charge and mass. Relative to electrons, which is another charged particle commonly utilized for radiotherapy, protons possess 1,836-fold greater mass. Their mass and charge impart protons with unique and beneficial radiotherapeutic characteristics that enable focused delivery of the radiation energy to the target, while avoiding radiation dose deposition to surrounding normal tissue. The dosimetric benefit of proton over photon (and electron)-based radiotherapy derives from these physical properties of the proton beam. As the proton beam reaches the end of its path, most of the proton energy is deposited at a depth determined by the energy of the beam. The high-energy deposition at the end of the proton beam path is termed the “Bragg peak”. Beyond the Bragg peak there is little or no additional dose deposited (i.e., “exit dose”). A summation of proton energies comprises the proton beam that is directed at a target. This summation is termed the “spread-out Bragg peak” and enables the delivery of highly conformal proton beams of which most of the energy is delivered within the target volume. By contrast, with X-ray (photon) radiation delivery (including IMRT), most of the total energy (integral dose) is delivered outside of the target volume due to both entrance and exit dose. Another difference between protons and photons is their relative biological effectiveness (RBE) compared to the physical dose of radiation (or, the amount of absorbed energy). Photons have a 1:1 ratio of RBE to physical dose (Gray), while the RBE of the proton beam is generally considered to be 1.1 and the proton dose is often presented as GyRBE. GyRBE for protons is calculated by multiplying the physical dose (Gray) by 1.1. This enables effective comparison of biological dosing between the two treatment modalities.
As with photon-based radiotherapy, there are varying techniques for proton planning and delivery. In passive-scattering proton therapy (PS) (also known as double-scattering) the proton beam is shaped in the lateral directions with the use of a physical block known as an aperture. The proximal and distal beams are shaped using a compensator to alter the depth of beam penetration as necessary to conform to the target. Pencil-beam scanning (PBS), on the other-hand, uses a magnetically guided narrow proton beam to scan across the target. PBS delivers protons to the target volume through the accumulation of layers of protons delivered throughout the depth of the target. PBS enables improved control of dose delivery at the proximal aspects of the beam and can provide improved conformality of high-dose regions. PBS delivery has enabled the development of intensity-modulated proton therapy (IMPT) (Figure 1). Treatment planning for PS proton therapy resembles that for 3DCRT in that it is done via forward treatment planning, while PBS/IMPT is akin to IMRT and utilizes inverse planning.
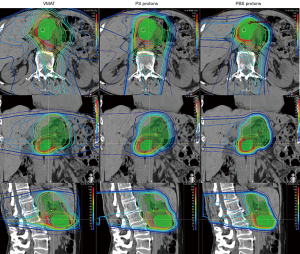
The dosimetric advantage of proton radiotherapy
An early radiation treatment planning study from Zurlo et al. compared two cases of locally advanced pancreatic head lesions planned with 3DCRT, IMRT, and PBS (31). The authors used each technique to generate plans to treat the primary tumor and regional lymphatics to 50 GyRBE followed by a 20–25 GyRBE boost to the primary tumor. Uniform dose constraints were applied to each modality. In both cases, only PBS was able to deliver the total dose (including the boost) while meeting the organ-at-risk (OAR) constraints. When planned to 50 GyRBE without the boost, only IMRT and PBS were able to deliver the prescription dose while meeting the OAR constraints; however, PBS demonstrated lower doses to the liver, bowel, and kidneys (31).
A recent study compared PBS proton planning and double-scattered proton planning for unresectable pancreatic cancer. Chuong et al. retrospectively generated PBS plans on 11 patients previously treated on a prospective phase 2 trial at The University of Florida (Jacksonville, FL, USA) with double-scattered proton radiotherapy to 59.4 CGE to the primary tumor (excluding elective lymph nodes) (32). The PBS treatment planners were blinded to the PS plans. PBS achieved improved target coverage, with the median PTV volume receiving the prescription dose of 97% versus 95.1% for double-scattered protons. PBS also achieved improved dose homogeneity (decreased hot spots) within the target volume compared to PS. Additionally, PBS achieved statistically lower median duodenal V59.4 CGE (37.4% vs. 40.4%) and lower stomach median V59.4 CGE (0.01% vs. 0.1%). A similar study conducted by Thompson et al. compared 13 patients planned to 55 GyRBE at 2.2 GyRBE per fraction with PS, PBS, and IMRT with unresectable cancer of the pancreatic head. They showed similar reductions in the low and moderate doses to the stomach and small bowel with protons (PBS or double scattering), with no benefit to protons in the high-dose range and perhaps an incremental benefit to IMRT (33). Similar to the Chuong et al. study, there were small but statistically significant benefits to PBS over PS in lowering the dose to the liver, small bowel, and duodenum.
In the postoperative setting, Nichols et al. conducted a dosimetric comparison between double-scattered protons and IMRT with optimized planning conducted at separate institutions with planners blinded to each other’s plans (34). Target volumes and planning goals were the same for each institution. Standard postoperative doses were utilized, delivering 45 GyRBE to the tumor bed and regional lymphatics and a 5.4-GyRBE boost to the tumor bed. Proton and IMRT planning equally covered the target volumes, while proton planning dramatically reduced the median V20 to the small bowel and stomach compared to IMRT: 15.4% vs. 47.0% and 2.3% vs. 20.0%, respectively. The kidney dose was also lowered with protons compared to IMRT (34).
Clinical outcomes using proton therapy
To date, there are no randomized data comparing proton and photon radiotherapy for pancreatic cancer. It is unlikely that such a trial will ever be seen to completion. The current evidence to support the use of proton radiotherapy comes from dosimetric studies (see above), single-institutional retrospective series, and single-arm prospective studies. Below is a summary of the current body of clinical evidence for proton therapy in the management of pancreatic carcinoma (Tables 1 and 2).
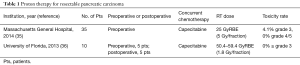
Full table
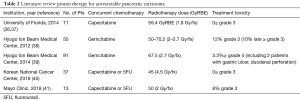
Full table
Resectable disease
A phase I feasibility study of short-course preoperative CRT for pancreatic head adenocarcinoma was completed by investigators at Massachusetts General Hospital (MGH; Boston, MA) (42). Hong et al. reported on 15 patients with localized, resectable disease treated under a dose-escalation schema beginning with 30 GyRBE in 10 fractions followed by several dose schemas consisting of 25 GyRBE in 5 fractions with progressively shorter delivery intervals (12 days followed by 11, 9, and then 5 consecutive treatment days). The rationale for this approach was to improve surgical outcomes and local control while shortening the duration that patients were without full-dose chemotherapy. Treatment volumes included the primary tumor and elective lymph nodes. Capecitabine at 1,650 mg/m2 was given twice daily for 2 weeks starting on the first day of radiotherapy. Six cycles of postoperative gemcitabine was recommended. No dose-limiting toxicities occurred. No delays in surgery occurred due to treatment toxicity. Four patients did not undergo resection: 3 due to metastatic progression and 1 due to unresectable disease at exploration. Nine of 11 patients had an R0 resection and 10 of 11 had positive lymph nodes. Six grade 3 acute toxicities occurred in 4 patients, including biliary obstruction (n=2), elevated total bilirubin (n=2), shoulder pain, and infection. Eleven patients remained alive with a median follow-up of 12 months. One patient experienced a local recurrence (with synchronous distant metastases).
In the phase 2 study that followed their feasibility study, investigators at MGH reported on an additional 35 patients treated with preoperative protons (25 GyRBE in 5 fractions) and concurrent capecitabine (35). The primary endpoint was to demonstrate a rate of ≥ grade 3 toxicity <20%. Surgery was performed 1 to 6 weeks after CRT. Six cycles of postoperative gemcitabine was recommended. A total of 37 patients (from the phase I/II studies) underwent surgical resection with 84% having negative surgical margins. Local-regional control was achieved in 84% of patients. Among the 35 patients included in the phase 2 study, 2 experienced grade 3 toxicity, including colitis and chest wall pain. Therefore, the study met its primary endpoint with a 4.1% rate of grade 3 toxicity. There were no grade 4 or 5 events. The study indicated that short-course proton radiotherapy with concurrent chemotherapy (encompassing the primary and elective lymphatics) was safe and well-tolerated, and resulted in excellent local control and R0 resection rates without delaying patient surgery.
Investigators at the University of Florida reported on 10 patients treated with passively scattered proton radiotherapy with concurrent capecitabine (1,000 mg/m2 BID) either preoperatively (n=5) or postoperatively (n=5) for borderline resectable pancreatic or ampullary adenocarcinoma (36). Radiotherapy doses ranged from 50.4 to 59.4 GyRBE. The primary focus of this study was on the tolerability of proton radiation with concurrent chemotherapy. With a median follow-up of 11 months, no patient experienced ≥ grade 3 toxicity during or after treatment. One patient experienced grade 2 GI toxicity, including abdominal pain and vomiting during CRT. Of note, this patient was treated with a field arrangement including an anterior field, which delivered a portion of the dose through the small bowel. The institutional approach for proton field arrangements for pancreatic carcinoma was subsequently modified to include posterior oblique fields and a lower weighted right lateral oblique field. Following this treatment planning modification, no further episodes of ≥ grade 2 toxicity occurred (36).
Investigators from the University of Pennsylvania (Philadelphia, PA, USA) presented an abstract analyzing toxicity in their series of patients treated with postoperative radiotherapy for pancreatic carcinoma (43). They compared 38 patients treated with proton radiotherapy and concurrent fluorouracil (5-FU) or capecitabine to 67 patients treated with photon radiotherapy (3D conformal or IMRT) plus the same chemotherapy. The median radiation dose was higher in the proton cohort (54 versus 50.4 GyRBE). Despite the higher postoperative radiation dose in the proton cohort, they reported 5% acute grade 3 GI toxicity in the proton cohort compared to 18% in the photon cohort. There was also a statistically significant increase in the number of photon patients compared to proton patients requiring 3 or more hospitalizations (43).
Unresectable disease
Investigators at the University of Florida published their series of 11 patients treated with CRT for unresectable pancreatic carcinoma (36,37). Patients were treated with passively scattered protons and concurrent capecitabine (1,000 mg/m2 BID). The radiotherapy dose delivered was 59.4 GyRBE in 1.8 GyRBE/fraction. Lymph node basins were not electively treated. The median overall follow-up was 14 months and the median follow-up for surviving patients was 23 months. One patient (9%) experienced grade 2 fatigue during CRT and no patient experienced acute ≥ grade 3 GI toxicity (36,37).
In a subsequent report, investigators at the University of Florida reported on the surgical outcomes of 6 patients from a series of 15 patients with unresectable pancreatic carcinoma (including the initial 11 from the series above) treated with concurrent proton radiotherapy (59.4 GyRBE) and concurrent capecitabine (44). The post-CRT radiographic response among the 6 patients warranted surgical exploration. Five patients underwent a pancreaticoduodenectomy and the sixth was found to have intra-abdominal metastases and therefore did not undergo surgery. The study evaluated surgical details including resection type (open vs. laparoscopic), length of surgery, blood loss, surgical complications, intensive care unit stay, and postoperative readmissions. Surgical outcomes were consistent with published historical benchmarks. This small series provided early evidence of the feasibility of high-dose preoperative CRT with proton therapy with respect to subsequent surgical resection (44).
Investigators at the Hyogo Ion Beam Medical Center (Tatsuno, Hyogo Prefecture, Japan) reported on 50 patients with locally advanced pancreatic cancer treated on 3 prospective trials utilizing proton radiotherapy and concurrent gemcitabine (800 mg/m2) (38). Treatment planning included gross disease and elective primary lymph node basins (celiac, SMA, paraaortic). The first two trials selected patients based on the relationship between the primary tumor and critical GI structures. Group P-1 included 5 patients with GI-adjacent LAPC treated with 50 GyRBE in 25 fractions and P-2 included 5 patients with non-GI adjacent LAPC treated with 70.2 GyRBE in 26 fractions. After completing accrual to P-1 and P-2, 40 patients (regardless of the relationship of the primary tumor to GI structures) were enrolled on P-3, which delivered 45 GyRBE to the entire PTV volume and 67.5 GyRBE in 25 fractions to gross disease (excluding the stomach and duodenum) using a field-within-a-field technique. All but 6 patients (12%) completed CRT as planned. 6 six patients who did not complete the planned course of CRT experienced grade 3 hematologic and/or GI toxicities. Late ≥ grade 3 toxicity included 5 patients (10%) with gastric ulcer, one of whom experienced a fatal hemorrhage. Other late severe toxicities included grade 3 anorexia and fatigue (38). With a median follow-up of 12.5 months, the 1-year rate of freedom from local-progression was 81.7% and overall survival was 76.8%.
The same group reported on the late GI toxicity of a subsequent series of 91 patients treated with the same aggressive regimen including 67.5 GyRBE (25 fractions) of proton radiotherapy with concurrent gemcitabine (800 mg/m2) given on days 1, 7, and 15 (39). With 10 months of follow-up, 1 patient (1.1%) experienced a grade 4 gastric ulcer and 2 patients (2.2%) experienced grade 5 toxicity, 1 from a bleeding gastric ulcer and 1 from a duodenal perforation (in the setting of a metal biliary stent) (39).
Investigators from the Korean National Cancer Center (Gyeonggi-do, South Korea) reported on 37 patients with locally advanced pancreas adenocarcinoma treated with passively scattered, hypofractionated proton radiotherapy delivering 30 GyRBE with a simultaneous integrated boost to the gross tumor to 45 GyRBE in 10 fractions. Most patients received concurrent chemotherapy (84%) with capecitabine or 5-FU (40). Two patients went to surgery after CRT and had R0 resections. With a median follow-up of 16.7 months, 5.4% developed local only failure, while 43.2% experienced local recurrence in addition to distant failure. Grade 2 anorexia (8.1%), vomiting (5.4%), and stomatitis (2.7%) were the only GI acute toxicities. No ≥ grade 3 acute or late radiation-related or GI toxicities were observed (40).
Investigators from the Mayo Clinic (Rochester, MN, USA) recently published their initial retrospective review of 13 patients treated with multifield, optimized IMPT with concurrent chemotherapy for borderline or unresectable pancreatic adenocarcinoma (41). Patients were treated with an initial target including the gross tumor volume and elective lymph nodes to 45 GyRBE (1.8 Gy/fx) with a simultaneous integrated boost to the gross tumor volume to 50 GyRBE (2 Gy/fraction) utilizing posterior and posterior oblique fields. Concurrent chemotherapy consisted of capecitabine (850 mg/m2 BID) or infusional 5-FU (225 mg/m2). One patient (8%) experienced grade 3 acute GI toxicity. All patients completed assessments for health-related quality of life outcomes using the Functional Assessment of Cancer Therapy- Hepatobiliary questionnaire. There were no significant differences in observed health-related quality of life between the pre- and post-CRT in their cohort (41).
Investigators from the Nagoya Proton Therapy Center in Japan presented data in abstract form of a prospective study of concurrent CRT utilizing hypofractionated proton radiotherapy and concurrent S-1 chemotherapy for locally advanced pancreatic cancer (45). Eighteen patients received 40 GyRBE in 2-GyRBE fractions to the clinical target volume with a concomitant boost to the gross tumor of 60 GyRBE in 3 GyRBE per fraction. With a median follow-up of 12 months, the 1-year local control and overall survival rates were 100% and 80%, respectively. One patient experienced a liver abscess and cholangitis, which the authors attributed to a hepaticogastrostomy procedure and a second patient experienced cholangitis after placement of a biliary stent. A third patient underwent endoscopic clipping for a grade 3 gastric ulcer at 4 months after CRT (45).
Future directions
Although the data are limited, the available studies utilizing proton radiotherapy for pancreatic carcinoma are very encouraging and support the need for additional trials to fully explore the benefits of this technology. Existing data indicate that proton radiotherapy combined with chemotherapy in the preoperative, adjuvant, and definitive settings is extremely well-tolerated and allows for the possibility of dose intensification. Several ongoing trials are investigating hypofractionated dose-escalated proton radiotherapy in combination with chemotherapy for unresectable/marginally resectable disease. Investigators at the University of Florida have a trial underway delivering 63 GyRBE in 2.25 GyRBE/fraction to gross disease while electively treating the high-risk nodal areas to 40.5 GyRBE with concurrent capecitabine (1,000 mg orally twice-daily). Investigators at the University of Maryland (Baltimore, MD, USA) are conducting a phase I/II study of dose-escalated proton therapy with concurrent nab-pacliaxel and gemcitabine in locally advanced pancreatic cancer. The study delivers 67.5 GyRBE in 4.5 GyRBE/fraction utilizing IMPT. The primary aim is to determine the maximum tolerated dose of gemcitabine and nab-paclitaxel with concurrent radiotherapy. Investigators at the University of Pennsylvania are completing a phase I trial for borderline/unresectable disease which involves dose escalation of nab-paclitaxel concurrent with combined proton/photon-based radiotherapy to determine the maximum tolerated dose (MTD) of chemotherapy. The second phase of the study will deliver nab-paclitaxel at the MTD concurrent with escalated doses of radiotherapy. These ongoing studies will provide important data detailing combinations of increasingly tumoricidal and radiosensitizing agents with more ablative doses of radiotherapy.
Improvements in proton planning and delivery techniques are helping to realize the full potential of proton radiotherapy. Given the limited number of proton facilities, many of the advances and supporting technologies seen over the past three decades for photon-based radiotherapy have not been as rapidly adapted to protons as has been the case with photons (e.g., advanced image guidance, arc therapy). With increasing interest, decreasing costs, and expanding numbers of proton facilities, the planning and delivery techniques for proton radiotherapy are enjoying rapid advances. IMPT utilizing pencil-beam scanning has been a major step forward in improving dosimetry at the proximal proton beam and improving conformality for irregular/complex targets. Advances such as CT-based image guidance improve the delivery of proton therapy (45). Work is also underway to explore the possibility of MRI-guided proton therapy as has recently become available for photon radiotherapy (46,47). Spot-scanning proton arc therapy (all known as SPArc) is in development and may help improve proton dosimetry and treatment efficiency compared to IMPT (48). Respiratory gating is also being used to improve delivery and better manage the impact of variable tissue densities and respiratory motion on proton range (45).
Conclusions
Proton radiotherapy for pancreatic carcinoma is effective and very well-tolerated in the pre- and postoperative settings. By helping reduce radiotherapy treatment-related toxicity, proton therapy can facilitate radiation dose escalation and/or chemotherapy intensification to improve outcomes. Importantly, well-tolerated radiotherapy can provide the radiotherapeutic benefits in local-regional control without the risk of chemotherapy or surgical delays. Negotiating the relationship between CRT for local-regional control and chemotherapy for systemic control will be increasingly important as outcomes continue to improve. With better systemic control and chemotherapy driving improvements in overall survival, the role of local-regional control will become increasingly salient in the management of pancreatic carcinoma. Proton therapy offers a promising approach to deliver well-tolerated radiotherapy to improve local-regional control and improve outcomes in pancreatic carcinoma.
Acknowledgments
Lovingly dedicated to the amazing life of Hal Isaacson and all of our patients who inspire us to do better.
Footnote
Conflicts of Interest: The authors have no conflicts of interest to declare.
Ethical Statement: The authors are accountable for all aspects of the work in ensuring that questions related to the accuracy or integrity of any part of the work are appropriately investigated and resolved.
References
- Maraldo MV, Giusti F, Vogelius IR, et al. Cardiovascular disease after treatment for Hodgkin's lymphoma: an analysis of nine collaborative EORTC-LYSA trials. Lancet Haematol 2015;2:e492-502. [Crossref] [PubMed]
- Siegel RL, Miller KD, Jemal A. Cancer statistics, 2018. CA Cancer J Clin 2018;68:7-30. [Crossref] [PubMed]
- Bray F, Ferlay J, Soerjomataram I, et al. Global cancer statistics 2018: GLOBOCAN estimates of incidence and mortality worldwide for 36 cancers in 185 countries. CA Cancer J Clin 2018;68:394-424. [Crossref] [PubMed]
- Treatment of locally unresectable carcinoma of the pancreas: comparison of combined-modality therapy (chemotherapy plus radiotherapy) to chemotherapy alone. Gastrointestinal Tumor Study Group. J Natl Cancer Inst 1988;80:751-5. [Crossref] [PubMed]
- Moertel CG, Frytak S, Hahn RG, et al. Therapy of locally unresectable pancreatic carcinoma: a randomized comparison of high dose (6000 rads) radiation alone, moderate dose radiation (4000 rads + 5-fluorouracil), and high dose radiation + 5-fluorouracil: The Gastrointestinal Tumor Study Group. Cancer 1981;48:1705-10. [Crossref] [PubMed]
- Loehrer PJ Sr, Feng Y, Cardenes H, et al. Gemcitabine alone versus gemcitabine plus radiotherapy in patients with locally advanced pancreatic cancer: an Eastern Cooperative Oncology Group trial. J Clin Oncol 2011;29:4105-12. [Crossref] [PubMed]
- Hammel P, Huguet F, van Laethem JL, et al. Effect of Chemoradiotherapy vs. Chemotherapy on Survival in Patients With Locally Advanced Pancreatic Cancer Controlled After 4 Months of Gemcitabine With or Without Erlotinib: The LAP07 Randomized Clinical Trial. JAMA 2016;315:1844-53. [Crossref] [PubMed]
- Kalser MH, Ellenberg SS. Pancreatic cancer. Adjuvant combined radiation and chemotherapy following curative resection. Arch Surg 1985;120:899-903. [Crossref] [PubMed]
- Klinkenbijl JH, Jeekel J, Sahmoud T, et al. Adjuvant radiotherapy and 5-fluorouracil after curative resection of cancer of the pancreas and periampullary region: phase III trial of the EORTC gastrointestinal tract cancer cooperative group. Ann Surg 1999;230:776-82; discussion 782-4. [Crossref] [PubMed]
- Neoptolemos JP, Stocken DD, Friess H, et al. A randomized trial of chemoradiotherapy and chemotherapy after resection of pancreatic cancer. N Engl J Med 2004;350:1200-10. [Crossref] [PubMed]
- Oettle H, Neuhaus P, Hochhaus A, et al. Adjuvant chemotherapy with gemcitabine and long-term outcomes among patients with resected pancreatic cancer: the CONKO-001 randomized trial. Jama 2013;310:1473-81. [Crossref] [PubMed]
- Neoptolemos JP, Palmer DH, Ghaneh P, et al. Comparison of adjuvant gemcitabine and capecitabine with gemcitabine monotherapy in patients with resected pancreatic cancer (ESPAC-4): a multicentre, open-label, randomised, phase 3 trial. Lancet 2017;389:1011-24. [Crossref] [PubMed]
- Conroy T, Desseigne F, Ychou M, et al. FOLFIRINOX versus gemcitabine for metastatic pancreatic cancer. N Engl J Med 2011;364:1817-25. [Crossref] [PubMed]
- Von Hoff DD, Ervin T, Arena FP, et al. Increased survival in pancreatic cancer with nab-paclitaxel plus gemcitabine. N Engl J Med 2013;369:1691-703. [Crossref] [PubMed]
- Van den Broeck A, Sergeant G, Ectors N, et al. Patterns of recurrence after curative resection of pancreatic ductal adenocarcinoma. Eur J Surg Oncol 2009;35:600-4. [Crossref] [PubMed]
- Groot VP, Rezaee N, Wu W, et al. Patterns, Timing, and Predictors of Recurrence Following Pancreatectomy for Pancreatic Ductal Adenocarcinoma. Ann Surg 2018;267:936-45. [Crossref] [PubMed]
- Tepper J, Nardi G, Sutt H. Carcinoma of the pancreas: review of MGH experience from 1963 to 1973. Analysis of surgical failure and implications for radiation therapy. Cancer 1976;37:1519-24. [Crossref] [PubMed]
- Griffin JF, Smalley SR, Jewell W, et al. Patterns of failure after curative resection of pancreatic carcinoma. Cancer 1990;66:56-61. [Crossref] [PubMed]
- Oettle H, Post S, Neuhaus P, et al. Adjuvant chemotherapy with gemcitabine vs. observation in patients undergoing curative-intent resection of pancreatic cancer: a randomized controlled trial. Jama 2007;297:267-77. [Crossref] [PubMed]
- Regine WF, Winter KA, Abrams RA, et al. Fluorouracil vs. gemcitabine chemotherapy before and after fluorouracil-based chemoradiation following resection of pancreatic adenocarcinoma: a randomized controlled trial. JAMA 2008;299:1019-26. [Crossref] [PubMed]
- Tienhoven GV, Versteiine E, Suker M, et al. Preoperative chemoradiotherapy versus immediate surgery for resectable borderline resectable pancreatic cancer (PREOPANC-1): A randomized, controlled, multicenter phase III trial. J Clin Oncol 2018;36:LBA4002. [Crossref]
- Conroy T, Hammel P, Hebbar M, et al. Unicancer GI PRODIGE 24/CCTG PA.6 trial: A multicenter international randomized phase III trial of adjuvant mFOLFIRINOX versus gemcitabine (gem) in patients with resected pancreatic ductal adenocarcinomas. J Clin Oncol 2018;36:LBA4001. [Crossref]
- Ben-Josef E, Shields AF, Vaishampayan U, et al. Intensity-modulated radiotherapy (IMRT) and concurrent capecitabine for pancreatic cancer. Int J Radiat Oncol Biol Phys 2004;59:454-9. [Crossref] [PubMed]
- Yovino S, Maidment BW 3rd, Herman JM, et al. Analysis of local control in patients receiving IMRT for resected pancreatic cancers. Int J Radiat Oncol Biol Phys 2012;83:916-20. [Crossref] [PubMed]
- Yovino S, Poppe M, Jabbour S, et al. Intensity-modulated radiation therapy significantly improves acute gastrointestinal toxicity in pancreatic and ampullary cancers. Int J Radiat Oncol Biol Phys 2011;79:158-62. [Crossref] [PubMed]
- Prasad S, Cambridge L, Huguet F, et al. Intensity modulated radiation therapy reduces gastrointestinal toxicity in locally advanced pancreas cancer. Pract Radiat Oncol 2016;6:78-85. [Crossref] [PubMed]
- Bittner MI, Grosu AL, Brunner TB. Comparison of toxicity after IMRT and 3D-conformal radiotherapy for patients with pancreatic cancer - a systematic review. Radiother Oncol 2015;114:117-21. [Crossref] [PubMed]
- Ben-Josef E, Schipper M, Francis IR, et al. A phase I/II trial of intensity modulated radiation (IMRT) dose escalation with concurrent fixed-dose rate gemcitabine (FDR-G) in patients with unresectable pancreatic cancer. Int J Radiat Oncol Biol Phys 2012;84:1166-71. [Crossref] [PubMed]
- Bouchard M, Amos RA, Briere TM, et al. Dose escalation with proton or photon radiation treatment for pancreatic cancer. Radiother Oncol 2009;92:238-43. [Crossref] [PubMed]
- Krishnan S, Chadha AS, Suh Y, et al. Focal Radiation Therapy Dose Escalation Improves Overall Survival in Locally Advanced Pancreatic Cancer Patients Receiving Induction Chemotherapy and Consolidative Chemoradiation. Int J Radiat Oncol Biol Phys 2016;94:755-65. [Crossref] [PubMed]
- Zurlo A, Lomax A, Hoess A, et al. The role of proton therapy in the treatment of large irradiation volumes: a comparative planning study of pancreatic and biliary tumors. Int J Radiat Oncol Biol Phys 2000;48:277-88. [Crossref] [PubMed]
- Chuong M, Badiyan SN, Yam M, et al. Pencil beam scanning versus passively scattered proton therapy for unresectable pancreatic cancer. J Gastrointest Oncol 2018;9:687-93. [Crossref] [PubMed]
- Thompson RF, Mayekar SU, Zhai H, et al. A dosimetric comparison of proton and photon therapy in unresectable cancers of the head of pancreas. Med Phys 2014;41:081711. [Crossref] [PubMed]
- Nichols RC Jr, Huh SN, Prado KL, et al. Protons offer reduced normal-tissue exposure for patients receiving postoperative radiotherapy for resected pancreatic head cancer. Int J Radiat Oncol Biol Phys 2012;83:158-63. [Crossref] [PubMed]
- Hong TS, Ryan DP, Borger DR, et al. A phase 1/2 and biomarker study of preoperative short course chemoradiation with proton beam therapy and capecitabine followed by early surgery for resectable pancreatic ductal adenocarcinoma. Int J Radiat Oncol Biol Phys 2014;89:830-8. [Crossref] [PubMed]
- Nichols RC Jr, George TJ, Zaiden RA Jr, et al. Proton therapy with concomitant capecitabine for pancreatic and ampullary cancers is associated with a low incidence of gastrointestinal toxicity. Acta Oncol 2013;52:498-505. [Crossref] [PubMed]
- Sachsman S, Nichols RC, Morris CG, et al. Proton Therapy and Concomitant Capecitabine for Non-Metastatic Unresectable Pancreatic Adenocarcinoma. Int J Part Ther 2014;1:692-701. [Crossref]
- Terashima K, Demizu Y, Hashimoto N, et al. A phase I/II study of gemcitabine-concurrent proton radiotherapy for locally advanced pancreatic cancer without distant metastasis. Radiother Oncol 2012;103:25-31. [Crossref] [PubMed]
- Takatori K, Terashima K, Yoshida R, et al. Upper gastrointestinal complications associated with gemcitabine-concurrent proton radiotherapy for inoperable pancreatic cancer. J Gastroenterol 2014;49:1074-80. [Crossref] [PubMed]
- Kim TH, Lee WJ, Woo SM, et al. Effectiveness and Safety of Simultaneous Integrated Boost-Proton Beam Therapy for Localized Pancreatic Cancer. Technol Cancer Res Treat 2018;17:1533033818783879. [Crossref] [PubMed]
- Jethwa KR, Tryggestad EJ, Whitaker TJ, et al. Initial experience with intensity modulated proton therapy for intact, clinically localized pancreas cancer: Clinical implementation, dosimetric analysis, acute treatment-related adverse events, and patient-reported outcomes. Adv Radiat Oncol 2018;3:314-21. [Crossref] [PubMed]
- Hong TS, Ryan DP, Blaszkowsky LS, et al. Phase I study of preoperative short-course chemoradiation with proton beam therapy and capecitabine for resectable pancreatic ductal adenocarcinoma of the head. Int J Radiat Oncol Biol Phys 2011;79:151-7. [Crossref] [PubMed]
- Woodhouse KD. Acute toxicity of proton versus photon adjuvant chemoradiation in the treatment of pancreatic cancer: A cohort study. Int J Radiat Oncol Biol Phys 2016;96:E208-9. [Crossref]
- Hitchcock KE, Nichols RC, Morris CG, et al. Feasibility of pancreatectomy following high-dose proton therapy for unresectable pancreatic cancer. World J Gastrointest Surg 2017;9:103-8. [Crossref] [PubMed]
- Hattori Y, Hirano A, Iwata H, et al. Image-Guided Hypofractionated Proton Therapy and Concurrent Chemotherapy for Inoperable Locally Advanced Pancreatic Cancer: Toxicities and Preliminary Outcomes. Int J Radiat Oncol Biol Phys 2017;99:E153. [Crossref]
- Moteabbed M, Schuemann J, Paganetti H. Dosimetric feasibility of real-time MRI-guided proton therapy. Med Phys 2014;41:111713. [Crossref] [PubMed]
- Padilla-Cabal F, Georg D, Fuchs H. A pencil beam algorithm for magnetic resonance image-guided proton therapy. Med Phys 2018;45:2195-204. [Crossref] [PubMed]
- Ding X, Li X, Zhang JM, et al. Spot-Scanning Proton Arc (SPArc) Therapy: The First Robust and Delivery-Efficient Spot-Scanning Proton Arc Therapy. Int J Radiat Oncol Biol Phys 2016;96:1107-16. [Crossref] [PubMed]