Proton beam therapy for liver cancers
Introduction
Primary and secondary malignancies of the liver represent a common and increasing cause of morbidity and mortality worldwide (1). Although surgical management offers the highest probability of long-term disease control and cure, the majority of patients are not appropriate for surgery due to advanced tumor burden and/or poor liver function. Therefore, non-surgical management is indicated for most patients with cancers of the liver. Non-surgical treatment options include systemic therapy, thermal ablation, chemoembolization, radioembolization, and external beam radiation therapy (EBRT). While there are few prospective comparative data to guide clinical decision making with respect to these options, there is increasing evidence that EBRT should be routinely considered for some liver cancer patients (2-7).
Liver EBRT with definitive doses was avoided in the era of 2-dimensional planning and delivery due to poor high dose conformity and consequentially a high risk of severe and potentially fatal radiation induced liver disease (RILD) (8,9). Major technological improvements in imaging, motion management, treatment planning, and dose conformity have allowed patients with liver cancer to safely and effectively receive EBRT with either X-rays or protons (10-12).
Herein we review the rationale, supporting clinical data, limitations, and future implications of proton beam therapy (PBT) in the treatment of primary and secondary liver malignancies.
Rationale for proton therapy
Compared to an X-ray beam in which dose exponentially decreases distal to the intended target, a proton beam has a finite range determined by its energy and deposits the majority of its dose over a short distance at the Bragg peak (13). Thus, there is essentially no exit dose in a proton beam, whereas a large volume of normal tissue receives low to moderate dose distal to the target with X-rays (Figure 1) (14).
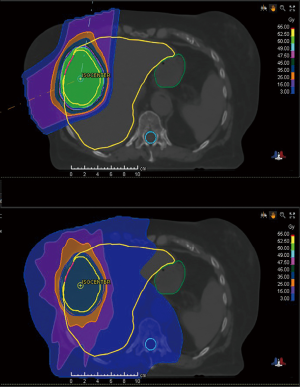
As a parallel functioning organ, the liver is tolerant of high dose to partial volumes, but not to the entire organ (15-17). The importance of minimizing the mean dose to uninvolved liver has been well understood for many years although the significance of low liver dose has more recently become recognized (18). In fact, even <10–15 Gy to increasing volumes of uninvolved liver has been shown as an important predictor of RILD (18,19).
For patients with HCC, the primary rationale for PBT is a reduction in the risk of RILD, especially those with more advanced tumor burden and/or worse liver function (20-23). Reducing normal liver dose with PBT can also facilitate tumor dose escalation, which may benefit some patients with unresectable intrahepatic cholangiocarcinoma or liver metastases as will be further discussed below (24,25). Although PBT may achieve sparing of other organs including the bowel, stomach, lung, and heart compared to the use of x-rays, these differences are not likely to be clinically meaningful for the majority of patients with liver cancer.
Clinical outcomes for HCC
PBT has been studied extensively for HCC in Japan where there is a high prevalence of the disease (26). A majority of the published literature has come from the University of Tsukuba, which began treating HCC patients with PBT in 1985 (27). Initial results in 162 HCC patients treated between 1985–1998 with various hypofractionated PBT schedules to a median 72 GyE in 16 fractions were encouraging (28). Only 3 or 4 fractions per week were initially delivered because of limited access to the beam, although 5 fractions per week later became standard. The median tumor size was 3.8 cm (range, 1.5–14.5 cm) and 51.6% of patients had multiple (up to 3) tumors that were encompassed in a single treatment field. The 5-year local control (LC) was 86.9% with no significant difference for tumors <5 vs. >5 cm. The 5-year overall survival (OS) was 23.5% and there was no grade 3 or higher toxicity despite only 50.6% of patients having CP-A cirrhosis.
While many dosing regimens were initially used, 3 have been preferred for many years at University of Tsukuba based on the location of the target: 66 GyE in 10 fractions for peripheral tumors, 72.6 GyE in 22 fractions if ≤2 cm from the porta hepatis, and 77 GyE in 35 fractions if ≤2 cm from gastrointestinal (GI) structures (29). In an analysis of 266 patients treated between 2001–2007 using this dosing strategy, the 5-year LC was 81% and did not differ based on fractionation schedule. There was a larger percentage of CP-A patients (76%) than in the previously mentioned study, which may have contributed to the higher 5-year OS of 48%. At least grade 3 toxicity was infrequent despite treating tumors in proximity to radiosensitive normal organs like bowel, demonstrating the appropriateness of using lower dose per fraction for central targets.
Fukuda et al. recently reported long-term outcomes (median follow-up 55 months) in previously untreated HCC patients from University of Tsukuba using tumor location-adapted dosing (30). The median tumor size was 3.9 cm (range, 1–13.5 cm) and about 25% of patients had multiple tumors. The 5-year LC for patients with stage 0/A, B, and C was 94%, 87%, and 75%, respectively. The 5-year OS was 69%, 66%, and 25%, respectively. Interestingly, the outcomes achieved with PBT for stage 0/A patients are similar to those achieved with partial hepatectomy or percutaneous ablation (31).
Favorable long-term PBT results have been achieved not only at University of Tsukuba, but also at other institutions in Japan, Korea, and the United States (Table 1). In most studies, 5-year LC was approximately 80–95% despite including tumors ranging from about 4–7 cm, which are larger than those typically represented in SBRT studies (28,30,34,36,38). Five-year OS varied from about 20–70%, reflecting a wide range of patient and tumor characteristics including liver function and tumor burden. Most patients in these studies had CP-A cirrhosis (~70–90%), similar to the SBRT literature. In 2005, investigators from Chiba reported that 80% complete radiographic response and 2-year LC 96% was achieved in 30 patients after a median 76 GyE in 20 fractions (32). A retrospective analysis from the Hyogo Ion Beam Medical Center in 242 patients treated with 8 PBT protocols (52.8–84 GyE in 4– 38 fractions) reported 5-year LC 90% and 5-year OS 38%. In 2011, Bush et al. (6) from Loma Linda University published results of a phase 2 trial that prescribed 63 GyE in 15 fractions to 76 patients with mean tumor size 5.5 cm. The majority had either CP-B (47%) or CP-C (24%) cirrhosis although liver transplantation was performed in 18 patients (24%). Pathologic complete response was found in 33% and 39% had only microscopic residual disease. No RILD was reported.
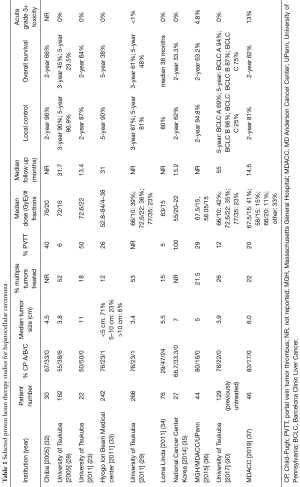
Full table
A 15-fraction approach was studied in a multi-institutional U.S. phase 2 trial that included both HCC (53%) and IHC (47%); median tumor size was 5.7 cm. The prescription dose was 67.50 GyE for peripheral tumors and 58.05 GyE ≤2 cm from the porta hepatis. The 2-year LC was excellent (95%) and only 3.6% developed a worse CP score after PBT (36). Researchers from M.D. Anderson Cancer Center recently reported a retrospective analysis of 46 patients treated with various fractionation schemes, including 15-fraction regimens of 58 and 67.5 Gy (56%). They noted a dose-response effect suggested by a median overall survival improvement from 15.8 to 49.9 months for patients treated with BED ≥90 GyE (P=0.037) (37). Higher BED remained significant (P=0.023) as an independent predictor of survival on multivariate analysis.
Although the data demonstrating safety and efficacy of PBT for HCC are well-established, it remains poorly defined how HCC patients should be optimally selected for PBT over SBRT in the absence of randomized data. Recently published retrospective data from Massachusetts General Hospital reported that PBT may improve OS compared to ablative XRT by decreasing the probability of post-treatment liver decompensation, even among CP-A patients (39). Two-year OS for PBT patients was 59.1% compared to 28.6% for XRT patients. The ongoing NRG Oncology GI-003 randomized trial will hopefully provide guidance on the benefit of PBT over XRT (NCT03186898).
HCC patients who are not ideal candidates for SBRT due to a high probability of RILD may be better suited for PBT (22,23,40-42). Sugahara et al. treated 22 patients with median 11 cm HCC (range, 10–14 cm) to a median 72.6 GyE in 22 fractions (range, 47.3–89.1 GyE in 10– 35 fractions) and achieved 2-year LC of 87% (23) with no grade 3 or higher toxicity. A study of 19 patients with CP-C cirrhosis prescribed a median 72 GyE in 16 fractions (range 50–84 GyE in 10–24 fractions) reported no worsening of CP score or grade 3+ toxicity; 74% actually experienced an improvement in CP score (22). LC was 95% with median follow up of 17 months and 2-year OS was 42%. Elderly patients can also achieve excellent outcomes from PBT; Hata et al. reported 3-year LC 100% and 3-year OS 62% in 21 patients with median age 81 years (range, 80–85 years) and none experienced grade 3 or higher toxicity (40). Hata et al. also showed that PBT was feasible and effective to treat portal vein tumor thrombus (PVTT) (41).
Several dosimetric studies have been performed that provide guidance on which patients may achieve the greatest clinical benefit from PBT. Toramatsu et al. evaluated the normal tissue complication probability for RILD based on the same prescription dose over varying tumor sizes and demonstrated that for tumors larger than 6.8 cm, the risk of RILD was significantly higher for SBRT compared to PBT (43). A study from the University of Pennsylvania concluded that PBT could be advantageous for tumors >3 cm in the center or dome of the liver or for >5 cm tumors regardless of location (21).
Although PBT will almost certainly deliver reduced liver dose in many instances in comparison to SBRT, there are many clinical factors such as baseline liver function that influence the probability of RILD. As such, best clinical judgment should be used to determine whether a certain reduction in liver dose achieved with PBT is clinically relevant in the context of other clinical factors.
Clinical outcomes for cholangiocarcinoma
Although the cornerstone of treatment for patients with unresectable cholangiocarcinoma is systemic therapy, long-term clinical outcomes may be greatly improved with the addition of EBRT in selected patients without distant metastatic disease (44-46). Moreover, in recent years, the use of ablative radiation dose has been shown to be a significant prognostic factor for both LC and OS (24,47). Several studies have demonstrated that dose-escalated PBT can achieve favorable LC and OS without significant toxicity, even for larger tumors.
Several retrospective studies from Japan showed the feasibility of PBT for cholangiocarcinoma. In 2014, Makita et al. examined the clinical outcomes of PBT in 28 patients with advanced unresectable cholangiocarcinoma with median tumor size 5.2 cm (range, 2–17.5 cm) (47). The median PBT dose was 68.2 GyE delivered in 2–3.2 Gy fractions (median BED10 =75.8 Gy). Although the overall 1-year LC was 67.7%, results were improved for patients who received a BED10 >70 Gy versus BED10 <70 Gy (1-year LC 83.1% vs. 22.2%, respectively). Only 1 patient had acute grade 3 toxicity (cholangitis) while 3 experienced late grade 3 toxicity (2 cholangitis, 1 common bile duct stenosis). In 2015, Ohkawa et al. published similar outcomes in 20 patients with unresectable intrahepatic cholangiocarcinoma (IHC) prescribed a medial total dose of 72.6 GyE in 22 fractions (BED10=96.56 Gy) (48). Among the patients treated with curative intent (n=12), the LC at 1, 2 and 3 years was 80%, 60% and 60%, respectively, while OS at 1, 2 and 3 years was 82%, 61% and 38%. No grade 3 or higher toxicity was observed.
Additional evidence supporting dose escalation for cholangiocarcinoma was reported from MD Anderson Cancer Center. Tao et al. published a retrospective dose response analysis in 79 patients treated with either protons or photons for unresectable IHC (24). The median tumor size was 7.9 cm (range, 2.2–17 cm) and median follow-up was 33 months (range, 11–93 months). The prescription dose varied between 35–100 Gy (median 58.05 Gy) in 3–30 fractions. The prescription dose was the single most significant prognostic factor for both LC and OS. Patients receiving BED >80.5 Gy had improved 3-year LC (78% vs. 45%; P=0.04) and 3-year OS (73% vs. 38%; P=0.017). No significant treatment-related toxicity was reported.
The use of ablative dose is also supported by prospective data. Hong et al. (36) reported in 2016 outcomes of a phase 2 clinical trial of dose-escalated hypofractionated PBT for patients with unresectable IHC (n=37), HCC (n=44), or mixed IHC/HCC (n=2). Single or multinodular tumors (up to three) were permitted. The median tumor size among IHC patients was 6 cm (range, 2.2–10.9 cm). The prescription dose was 67.5 GyE for peripheral tumors (>2 cm from porta hepatis) and 58.05 GyE for central tumors, all delivered in 15 fractions. With median follow-up of 19.5 months, the 2-year LC among the IHC cohort was 94.1% and 2-year OS was 46.5%. Grade 3 toxicity occurred in only 4 patients (4.8%).
Proton therapy for liver metastases
Although SBRT is well-established for treatment of relatively small or limited number of liver metastases, prescribing high doses to large tumors while also meeting normal organ constraints may not always be feasible (5,25,49). PBT may be indicated to treat such patients with advanced tumor burden given that there is strong evidence that BED10 >100 Gy is required to obtain long-term LC of liver metastases (25) . Although the PBT literature for liver metastases is limited, the available studies collectively demonstrate that ablative PBT can safely achieve durable LC even among patients with larger liver metastases (50-56).
Investigators from University of Tsukuba evaluated PBT for liver metastases from various primary tumor sites (54). Fukumitsu et al. published a retrospective study of 133 patients with liver metastases from predominantly colorectal, pancreatic, and breast cancers (54). Liver-only metastases were present in 61% of patients. The median tumor size was 4 cm although tumors up to 18 cm were treated. The most frequent dose schedule was 72.6 GyE delivered in 22 fractions (BED10=96.56 Gy). LC at 2 and 5 years was 66% and 53%, and OS rates were 46% and 25%, respectively. Seven of 8 patients with lesions >10 cm had no local recurrence or significant toxicity. More recent studies from University of Tsukuba have reported excellent LC with minimal toxicity especially for >5 cm metastases from gastric (52) and breast (53) cancers.
Investigators from MD Anderson Cancer Center evaluated PBT as a salvage therapy for bilateral colorectal metastases not amenable to second-stage hepatectomy (55). Right hemiliver ablative PBT was delivered to 5 patients with a prescription dose of 67.5 GyE in 15 fractions (BED10=97.9 Gy), 100 GyE in 25 fractions (BED10=140 Gy), or 70 Gy in 28 fractions (BED10=89.6 Gy). All patients achieved LC with the exception of one who received a lesser BED10 (89.6 Gy).
In 2017 Hong et al. published results of a phase 1/2 study of proton-based SBRT for liver metastases (56). In this trial, 89 patients with different primaries, predominantly colorectal, were evaluated. Patients had no or limited extrahepatic disease. Up to 4 tumors were permitted and there was no upper limit on tumor size, but at least 800 mL of uninvolved liver was required. Median tumor size was 2.5 cm (range, 0.5–11.9 cm) and in 23 patients, the tumor diameter was at least 6 cm. Median prescribed radiation dose was 40 GyE (range, 30–50 GyE) delivered in 5 fractions (BED10 72 Gy) although the authors acknowledge that higher doses could have been prescribed, which likely would have improved tumor control. LC rates at 1 and 3 years were 71.9% and 61.2%. For ≥6 cm tumors 1-year LC was 73.9%. Median overall survival was 18.1 months for the whole group. There were no grade 3–5 toxicities.
Challenges and limitations of liver PBT
There are several important treatment planning and delivery concerns that should be considered for any patient receiving liver PBT. Although proton beams have a distinct advantage of finite range with little to no exit dose beyond the target, the exact range of a proton beam is uncertain. This range uncertainty is related to inaccuracies in Hounsfield Unit value determination obtained from CT simulation scans. To address this uncertainty, margin is added to target regions to ensure that the proton beam does not terminate until it completely traverses the desired target (57). As a consequence, high dose conformality is sacrificed to enhance precision.
The range of a proton beam is highly influenced by changes in tissue electron density within the beam path. Variation in tissue electron density can have dramatic effects on proton beams either ranging beyond the target and delivering unintended dose to normal tissue or being stopped early and not delivering the intended dose to the target. In contrast, the same electron density changes would have relatively little effect on an X-ray beam. Therefore, it is important to consider strategies ensuring highly reproducible patient setup, robust treatment planning methods such repainting, 4-dimensional robust optimization, and selecting beam angles that minimize delivering protons through highly variable soft tissue regions (e.g., bowel).
Range uncertainty concerns are further complicated by the liver being a moving target, especially because of the large difference in lower electron density of air in the lung and the higher electron density of the liver. Motion management strategies to either temporarily eliminate or at least keep respiratory motion to a minimum should be strongly considered for treating liver cancers with PBT. Respiratory gating began at University of Tsukuba in 1992 and is still used today (58). Other techniques used to account for respiratory motion include abdominal compression, breath-hold and 4-dimensional (4D) planning (59).
The vast majority of PBT outcomes for liver cancers have been reported using passive scattering (PS), although pencil beam scanning (PBS) is becoming increasingly used. Although PBS offers superior dose conformality, it is more susceptible to differences in tissue electron density and therefore is inherently less robust than PS. Nonetheless, robust PBS plans can be achieved provided that motion-robust planning and motion management techniques are used (60,61). In fact, a recent study from Germany showed that 4D-optimized PBS plans provided better sparing of organs at risk than those created with PS (61).
Conclusions
The dosimetric advantage of PBT over XRT in sparing uninvolved liver from low and moderate doses clearly translates into clinically meaningful benefit for some patients with liver cancer. PBT for HCC has been evaluated over several decades with low rates of toxicity and excellent long-term LC even in patients with large tumors. Based on these outcomes, PBT receives the highest level of support (Group 1 recommendation) in the 2017 ASTRO Proton Beam Therapy Model Policy (62). For patients with intrahepatic cholangiocarcinoma and liver metastases, favorable tumor control, survival, and toxicity outcomes have been demonstrated after ablative PBT; however, additional research is needed to better understand the role of such therapy in the context of other liver-directed therapies.
While the potential benefit from PBT for liver cancers, especially HCC, is largely undisputed, there is a lack of guidance about optimal patient selection for PBT. All patients with liver cancer are not expected to benefit from PBT compared to XRT or other liver-directed therapies. As such, identifying patient subgroups that are most appropriate for PBT should be a priority in future research.
Acknowledgments
None.
Footnote
Conflicts of Interest: The authors have no conflicts of interest to declare.
Ethical Statement: The authors are accountable for all aspects of the work in ensuring that questions related to the accuracy or integrity of any part of the work are appropriately investigated and resolved.
References
- Bray F, Ferlay J, Soerjomataram I, et al. Global cancer statistics 2018: Globocan estimates of incidence and mortality worldwide for 36 cancers in 185 countries. CA Cancer J Clin 2018;68:394-424. [Crossref] [PubMed]
- Klein J, Dawson LA. Hepatocellular carcinoma radiation therapy: Review of evidence and future opportunities. Int J Radiat Oncol Biol Phys 2013;87:22-32. [Crossref] [PubMed]
- Jackson WC, Tao Y, Mendiratta-Lala M, et al. Comparison of stereotactic body radiation therapy and radiofrequency ablation in the treatment of intrahepatic metastases. Int J Radiat Oncol Biol Phys 2018;100:950-8. [Crossref] [PubMed]
- Sapir E, Tao Y, Schipper MJ, et al. Stereotactic body radiation therapy as an alternative to transarterial chemoembolization for hepatocellular carcinoma. Int J Radiat Oncol Biol Phys 2018;100:122-30. [Crossref] [PubMed]
- Robin TP, Raben D, Schefter TE. A contemporary update on the role of stereotactic body radiation therapy (sbrt) for liver metastases in the evolving landscape of oligometastatic disease management. Semin Radiat Oncol 2018;28:288-94. [Crossref] [PubMed]
- Bush DA, Smith JC, Slater JD, et al. Randomized clinical trial comparing proton beam radiation therapy with transarterial chemoembolization for hepatocellular carcinoma: Results of an interim analysis. Int J Radiat Oncol Biol Phys 2016;95:477-82. [Crossref] [PubMed]
- Jiang W, Zeng ZC. Is it time to adopt external beam radiotherapy in the nccn guidelines as a therapeutic strategy for intermediate/advanced hepatocellular carcinoma? Oncology 2013;84 Suppl 1:69-74. [Crossref] [PubMed]
- Phillips R, Karnofsky DA, Hamilton LD, et al. Roentgen therapy of hepatic metastases. Am J Roentgenol Radium Ther Nucl Med 1954;71:826-34. [PubMed]
- Lawrence TS, Robertson JM, Anscher MS, et al. Hepatic toxicity resulting from cancer treatment. Int J Radiat Oncol Biol Phys 1995;31:1237-48. [Crossref] [PubMed]
- Yu Y, Feng M. Radiotherapy for hepatocellular carcinoma. Semin Radiat Oncol 2018;28:277-87. [Crossref] [PubMed]
- Koay EJ, Owen D, Das P. Radiation-induced liver disease and modern radiotherapy. Semin Radiat Oncol 2018;28:321-31. [Crossref] [PubMed]
- Apisarnthanarax S, Bowen SR, Combs SE. Proton beam therapy and carbon ion radiotherapy for hepatocellular carcinoma. Semin Radiat Oncol 2018;28:309-20. [Crossref] [PubMed]
- Engelsman M, Schwarz M, Dong L. Physics controversies in proton therapy. Semin Radiat Oncol 2013;23:88-96. [Crossref] [PubMed]
- Wang X, Krishnan S, Zhang X, et al. Proton radiotherapy for liver tumors: Dosimetric advantages over photon plans. Med Dosim 2008;33:259-67. [Crossref] [PubMed]
- Dawson LA, Normolle D, Balter JM, et al. Analysis of radiation-induced liver disease using the lyman ntcp model. Int J Radiat Oncol Biol Phys 2002;53:810-21. [Crossref] [PubMed]
- Dawson LA, Ten Haken RK. Partial volume tolerance of the liver to radiation. Semin Radiat Oncol 2005;15:279-83. [Crossref] [PubMed]
- Pan CC, Kavanagh BD, Dawson LA, et al. Radiation-associated liver injury. Int J Radiat Oncol Biol Phys 2010;76:S94-100. [Crossref] [PubMed]
- Velec M, Haddad CR, Craig T, et al. Predictors of liver toxicity following stereotactic body radiation therapy for hepatocellular carcinoma. Int J Radiat Oncol Biol Phys 2017;97:939-46. [Crossref] [PubMed]
- Mizumoto M, Okumura T, Hashimoto T, et al. Evaluation of liver function after proton beam therapy for hepatocellular carcinoma. Int J Radiat Oncol Biol Phys 2012;82:e529-35. [Crossref] [PubMed]
- Dawson LA. Protons or photons for hepatocellular carcinoma? Let's move forward together. Int J Radiat Oncol Biol Phys 2009;74:661-3. [Crossref] [PubMed]
- Gandhi SJ, Liang X, Ding X, et al. Clinical decision tool for optimal delivery of liver stereotactic body radiation therapy: Photons versus protons. Pract Radiat Oncol 2015;5:209-18. [Crossref] [PubMed]
- Hata M, Tokuuye K, Sugahara S, et al. Proton beam therapy for hepatocellular carcinoma patients with severe cirrhosis. Strahlenther Onkol 2006;182:713-20. [Crossref] [PubMed]
- Sugahara S, Oshiro Y, Nakayama H, et al. Proton beam therapy for large hepatocellular carcinoma. Int J Radiat Oncol Biol Phys 2010;76:460-6. [Crossref] [PubMed]
- Tao R, Krishnan S, Bhosale PR, et al. Ablative radiotherapy doses lead to a substantial prolongation of survival in patients with inoperable intrahepatic cholangiocarcinoma: A retrospective dose response analysis. J Clin Oncol 2016;34:219-26. [Crossref] [PubMed]
- Ohri N, Tome WA, Mendez Romero A, et al. Local control after stereotactic body radiation therapy for liver tumors. Int J Radiat Oncol Biol Phys 2018. [Epub ahead of print]. [Crossref] [PubMed]
- Ferlay J, Colombet M, Soerjomataram I, et al. Estimating the global cancer incidence and mortality in 2018: Globocan sources and methods. Int J Cancer 2019;144:1941-53. [Crossref] [PubMed]
- Tsujii H, Tsuji H, Inada T, et al. Clinical results of fractionated proton therapy. Int J Radiat Oncol Biol Phys 1993;25:49-60. [Crossref] [PubMed]
- Chiba T, Tokuuye K, Matsuzaki Y, et al. Proton beam therapy for hepatocellular carcinoma: A retrospective review of 162 patients. Clin Cancer Res 2005;11:3799-805. [Crossref] [PubMed]
- Mizumoto M, Okumura T, Hashimoto T, et al. Proton beam therapy for hepatocellular carcinoma: A comparison of three treatment protocols. Int J Radiat Oncol Biol Phys 2011;81:1039-45. [Crossref] [PubMed]
- Fukuda K, Okumura T, Abei M, et al. Long-term outcomes of proton beam therapy in patients with previously untreated hepatocellular carcinoma. Cancer Sci 2017;108:497-503. [Crossref] [PubMed]
- Gravante G, Overton J, Sorge R, et al. Radiofrequency ablation versus resection for liver tumours: An evidence-based approach to retrospective comparative studies. J Gastrointest Surg 2011;15:378-87. [Crossref] [PubMed]
- Kawashima M, Furuse J, Nishio T, et al. Phase ii study of radiotherapy employing proton beam for hepatocellular carcinoma. J Clin Oncol 2005;23:1839-46. [Crossref] [PubMed]
- Komatsu S, Fukumoto T, Demizu Y, et al. Clinical results and risk factors of proton and carbon ion therapy for hepatocellular carcinoma. Cancer 2011;117:4890-904. [Crossref] [PubMed]
- Bush DA, Hillebrand DJ, Slater JM, et al. High-dose proton beam radiotherapy of hepatocellular carcinoma: Preliminary results of a phase II trial. Gastroenterology 2004;127:S189-93. [Crossref] [PubMed]
- Lee SU, Park JW, Kim TH, et al. Effectiveness and safety of proton beam therapy for advanced hepatocellular carcinoma with portal vein tumor thrombosis. Strahlenther Onkol 2014;190:806-14. [Crossref] [PubMed]
- Hong TS, Wo JY, Yeap BY, et al. Multi-institutional phase II study of high-dose hypofractionated proton beam therapy in patients with localized, unresectable hepatocellular carcinoma and intrahepatic cholangiocarcinoma. J Clin Oncol 2016;34:460-8. [Crossref] [PubMed]
- Chadha AS, Gunther JR, Hsieh CE, et al. Proton beam therapy outcomes for localized unresectable hepatocellular carcinoma. Radiother Oncol 2019;133:54-61. [Crossref] [PubMed]
- Kim DY, Park JW, Kim TH, et al. Risk-adapted simultaneous integrated boost-proton beam therapy (sib-pbt) for advanced hepatocellular carcinoma with tumour vascular thrombosis. Radiother Oncol 2017;122:122-9. [Crossref] [PubMed]
- Sanford NN, Pursley J, Noe B, et al. Protons versus Photons for Unresectable Hepatocellular Carcinoma: Liver Decompensation and Overall Survival. Int J Radiat Oncol Biol Phys 2019;105:64-72. [Crossref] [PubMed]
- Hata M, Tokuuye K, Sugahara S, et al. Proton beam therapy for aged patients with hepatocellular carcinoma. Int J Radiat Oncol Biol Phys 2007;69:805-12. [Crossref] [PubMed]
- Hata M, Tokuuye K, Sugahara S, et al. Proton beam therapy for hepatocellular carcinoma with portal vein tumor thrombus. Cancer 2005;104:794-801. [Crossref] [PubMed]
- Hata M, Tokuuye K, Sugahara S, et al. Proton beam therapy for hepatocellular carcinoma with limited treatment options. Cancer 2006;107:591-8. [Crossref] [PubMed]
- Toramatsu C, Katoh N, Shimizu S, et al. What is the appropriate size criterion for proton radiotherapy for hepatocellular carcinoma? A dosimetric comparison of spot-scanning proton therapy versus intensity-modulated radiation therapy. Radiat Oncol 2013;8:48. [Crossref] [PubMed]
- Yamashita S, Koay EJ, Passot G, et al. Local therapy reduces the risk of liver failure and improves survival in patients with intrahepatic cholangiocarcinoma: A comprehensive analysis of 362 consecutive patients. Cancer 2017;123:1354-62. [Crossref] [PubMed]
- Lee J, Yoon WS, Koom WS, et al. Efficacy of stereotactic body radiotherapy for unresectable or recurrent cholangiocarcinoma: A meta-analysis and systematic review. Strahlenther Onkol 2019;195:93-102. [Crossref] [PubMed]
- Gkika E, Hallauer L, Kirste S, et al. Stereotactic body radiotherapy (sbrt) for locally advanced intrahepatic and extrahepatic cholangiocarcinoma. BMC Cancer 2017;17:781. [Crossref] [PubMed]
- Makita C, Nakamura T, Takada A, et al. Clinical outcomes and toxicity of proton beam therapy for advanced cholangiocarcinoma. Radiat Oncol 2014;9:26. [Crossref] [PubMed]
- Ohkawa A, Mizumoto M, Ishikawa H, et al. Proton beam therapy for unresectable intrahepatic cholangiocarcinoma. J Gastroenterol Hepatol 2015;30:957-63. [Crossref] [PubMed]
- Rusthoven KE, Kavanagh BD, Cardenes H, et al. Multi-institutional phase i/ii trial of stereotactic body radiation therapy for liver metastases. J Clin Oncol 2009;27:1572-8. [Crossref] [PubMed]
- Petersen JB, Lassen Y, Hansen AT, et al. Normal liver tissue sparing by intensity-modulated proton stereotactic body radiotherapy for solitary liver tumours. Acta Oncol 2011;50:823-8. [Crossref] [PubMed]
- Ahmed KA, Caudell JJ, El-Haddad G, et al. Radiosensitivity differences between liver metastases based on primary histology suggest implications for clinical outcomes after stereotactic body radiation therapy. Int J Radiat Oncol Biol Phys 2016;95:1399-404. [Crossref] [PubMed]
- Fukumitsu N, Okumura T, Takizawa D, et al. Proton beam therapy for liver metastases from gastric cancer. J Radiat Res 2017;58:357-62. [Crossref] [PubMed]
- Fukumitsu N, Okumura T, Numajiri H, et al. Follow-up study of liver metastasis from breast cancer treated by proton beam therapy. Mol Clin Oncol 2017;7:56-60. [Crossref] [PubMed]
- Fukumitsu N, Okumura T, Takizawa D, et al. Proton beam therapy for metastatic liver tumors. Radiother Oncol 2015;117:322-7. [Crossref] [PubMed]
- Colbert LE, Cloyd JM, Koay EJ, et al. Proton beam radiation as salvage therapy for bilateral colorectal liver metastases not amenable to second-stage hepatectomy. Surgery 2017;161:1543-8. [Crossref] [PubMed]
- Hong TS, Wo JY, Borger DR, et al. Phase ii study of proton-based stereotactic body radiation therapy for liver metastases: Importance of tumor genotype. J Natl Cancer Inst 2017;109:djx031. [Crossref] [PubMed]
- McGowan SE, Burnet NG, Lomax AJ. Treatment planning optimisation in proton therapy. Br J Radiol 2013;86:20120288. [Crossref] [PubMed]
- Inada T, Tsuji H, Hayakawa Y, et al. Proton irradiation synchronized with respiratory cycle. Nihon Igaku Hoshasen Gakkai Zasshi 1992;52:1161-7. [PubMed]
- Bert C, Durante M. Motion in radiotherapy: Particle therapy. Phys Med Biol 2011;56:R113-44. [Crossref] [PubMed]
- Poulsen PR, Eley J, Langner U, et al. Efficient interplay effect mitigation for proton pencil beam scanning by spot-adapted layered repainting evenly spread out over the full breathing cycle. Int J Radiat Oncol Biol Phys 2018;100:226-34. [Crossref] [PubMed]
- Pfeiler T, Ahmad Khalil D, Ayadi M, et al. Motion effects in proton treatments of hepatocellular carcinoma-4d robustly optimised pencil beam scanning plans versus double scattering plans. Phys Med Biol 2018;63:235006. [Crossref] [PubMed]
- Astro model policy for proton beam therapy. 2017. Available online: https://www.astro.org/uploadedFiles/_MAIN_SITE/Daily_Practice/Reimbursement/Model_Policies/Content_Pieces/ASTROPBTModelPolicy.pdf