Proton beam radiotherapy for anal and rectal cancers
Methods
This review was conducted using the Preferred Reporting Items for Systematic Reviews and Meta-Analyses (PRISMA) guidelines (1). Eligibility criteria included published work evaluating treatment plans and clinical outcomes of proton radiation therapy for anal or rectal cancer. Sources of information for this review included PubMed and EMBASE, those found in references from the major articles identified, and articles known to the authors. The searches were conducted to identify any and all articles addressing clinical outcomes of proton radiotherapy for anal or rectal neoplasms in adults with the following headings: protons, proton radiation therapy, proton beam therapy (PBT), pencil-beam, intensity-modulated PBT (IMPT), anal, anal cancer, rectal, or rectal cancer. Due to the limited amount of overall data, search terms were not restricted by publication year or number of patients. Searches were completed by October 4th, 2018.
Based on the initial searches, a total of 575 articles and abstracts were identified. Care was taken to ensure that the inclusion criteria were sufficiently broad. To avoid missing potentially relevant publications, sources were excluded by individual screening rather than the initial database search. After duplicates were removed and the papers were independently screened for the described criteria, a further 528 studies were excluded. Articles without specific assessment of dosimetry or clinically relevant outcomes (e.g., survival, toxicity) of proton radiation therapy (RT) for anorectal cancers in adults were excluded. Of the 47 publications remaining, 17 review articles and 30 original investigations (15 published articles, 11 abstracts, and 4 case reports) were found to have sufficient focus and relevance to be incorporated into the review.
Rationale
PBT is increasingly used for the treatment of pediatric, central nervous system, skull base, and head and neck tumors. Today, 28 proton beam centers are in operation, with 23 in development. Whether PBT can play a similarly important role in the multidisciplinary treatment of anorectal cancer is under active investigation.
PBT is a form of external beam radiotherapy that affords excellent dose distributions due to the near-total absence of exit dose. It gradually transfers energy to tissues and culminates in the Bragg peak at the end of the beam path. Protons have a finite range based on their initial energy, and doses distal to the Bragg peak are essentially nonexistent for PBT. In contrast, photons gradually lose their energy as they continue beyond the target. Radiation plans can spread out the Bragg peak to conform to the tumor volume using generally 1–3 treatment fields, whereas multiple beam orientations are needed for photons to minimize doses to organs at risk (OARs) (2). Given the minimal difference in biological effect between both modalities, protons have drawn interest as a method to spare adjacent OARs while delivering tumoricidal doses and increasing the therapeutic ratio. Since PBT has been largely untested in gastrointestinal (GI) malignancies, recent studies have aimed to address whether the theoretical reduced dose to OARs translates to a decline in acute and long-term toxicities, such as secondary malignancies.
Indications
Rectal cancer
Over the past decades, the role of RT in the management of rectal cancer has evolved. Even after consideration of current advances in surgical technique, RT and chemotherapy still improve sphincter preservation, local control, and survival (3-9). In the United States, standard of care for stage II or III rectal adenocarcinoma includes neoadjuvant chemoradiation followed by total mesorectal excision and adjuvant FOLFOX for 4– 6 months. Preoperative radiation is typically given at doses of 50.4 Gy in 28 fractions alongside concurrent 5-fluorouracil (5-FU) or capecitabine-based chemotherapy (4). While supported by randomized data, short-course radiation (5 Gy ×5 fractions), to date, has not been widely adopted in the United States due to concerns for late toxicity (8,10). Despite standard of care, local control and survival rates are poor for unresectable T4 or cT3 tumors with lateral node or mesorectal fascia involvement (11).
Like surgery, radiation carries its own morbidity profile, with over 25% of patients developing grade 3+ acute toxicity during neoadjuvant treatment (4). The lifetime dose at which adverse effects of radiation outweigh clinical benefit is unknown. Studies have suggested that the amount of small bowel receiving 15 Gy predicts acute toxicity and that reduction of this low-dose bath improves patient-reported outcomes (12). With currently available photon-based plans, hematologic and GI toxicity, such as cramping and diarrhea, predominate (4). Additionally, 10–25% of patients with locally advanced rectal adenocarcinoma recur within or near the radiation field (4). In the setting of recurrence, surgery or reirradiation is often infeasible due to either the late effects of prior radiation on the surgical field or the cumulative effect of radiation on OARs. In this setting, surgery offers a 5-year survival rate of 17–36% and carries notable risks to quality of life, including sacral nerve damage with high sacral resection and the frequent need to create two stomas (13-16).
The ability to reduce toxicity to OARs could enable preoperative dose escalation which has been explored as a strategy to increase pathological complete response (CR) and clinical outcomes (17-20). In light of the emerging “watch and wait” era, protons may offer a feasible solution to increase clinical complete response (cCR) rates within the paradigm of a non-operative approach to rectal cancer. In cases where surgery is appropriate, dose escalation could result in downstaging, in turn increasing sphincter preservation rates and enabling removal of previously inextirpable tumors (4,21-24). Additionally, PBT theoretically may minimize acute and late toxicity to visceral organs, reducing long-term treatment-related sequelae from dose escalation.
Anal cancer
Definitive radiation combined with 5-FU and mitomycin-C (MMC) is the standard of care for local and locally advanced squamous cell carcinoma of the anal canal (SCCA), the predominant form of anal cancer (25). Recurrent or residual disease is managed with surgery after completing first-line chemoradiation. Radiation doses range from 30 Gy to greater than 60 Gy depending on tumor stage. In patients with early stage cancers, locoregional control is typically achieved and the 5-year survival rate is between 80% and 90%. Relapse-free survival and colostomy-free survival rates decline to 50–60% for advanced stage cancers (T3 or T4) (26-29).
Toxicity from chemoradiation remains a major challenge which prevents timely delivery and intensity of therapy. Hematologic toxicity predominates due to the combination of myelosuppressive 5-FU, MMC, and RT to the pelvis, which contains 40% of the body’s active marrow (30). The bone marrow compartment is sacrificed in order to achieve planning target volume (PTV) coverage and avoid small bowel. According to the Intergroup trial, 18% of patients develop grade 4 or 5 acute hematologic toxicity from standard of care treatment (31). This contrasts with the 6% rate of grade 3 or 4 hematologic toxicity seen in the German study for patients treated for rectal cancer (4). In addition, GI toxicity, dysuria, and late effects on sexual function occur, which negatively impact quality of life. Unlike other GI malignancies, anal cancer requires inclusion of the perianal skin and superficial lymph nodes in treatment plans, resulting in dermatitis and confluent skin breakdown (32,33).
The ability of precision therapy to reduce toxicity was demonstrated in RTOG 0529 which prospectively implemented dose-painted intensity modulated radiation therapy (IMRT) in a phase II trial design (34). In the study, grade 2 or higher hematologic toxicity and grade 3 or higher GI and dermatologic side effects were significantly reduced when compared to the standard arm of RTOG 9811. Acute morbidity was still common, however, with grade 2+ and 3+ hematologic toxicity seen in 73% and 58% of patients, respectively. Given the improvement of IMRT over 2 or 4-field 3D photon techniques, PBT may offer a similar integral benefit over conformal photon therapy, enabling further reduction in toxicity. Additionally, some studies have suggested that long-term outcomes are associated with time to completion of therapy and radiation dose (35-45). One can extrapolate that PBT’s side-effect profile may reduce the need for treatment breaks and allow for safe dose escalation, enabling improved clinical outcomes.
Clinical evidence
Rectal cancer
Data on the benefit of PBT for rectal cancer is derived primarily from preclinical planning studies for neoadjuvant treatment. Colaco et al. compared plans for 8 consecutive patients undergoing neoadjuvant chemoradiation for resectable (T2–T3) rectal cancer (46). PBT plans utilized a uniform 3-field approach with heavier weighting of the posterior field relative to the lateral fields, and Hounsfield units were overridden for the circumferential air-filled portion of the rectum. Protons significantly reduced pelvic bone marrow exposure at the V5–V20Gy levels and small bowel exposure at the V10Gy and V20Gy levels compared to IMRT and 3DCRT. Small bowel exposure at the V30Gy and V40Gy levels and urinary bladder exposure at the V40Gy level were also improved compared to 3DCRT.
This is consistent with work by Wolff et al. who retrospectively compared preoperative treatment plans using protons, RapidArc, IMRT, and 3DCRT in 25 patients with stage II or III rectal cancer (47). Dose-volume histogram (DVH)-analyses revealed significantly reduced doses to OARs including the small bowel, testes, and bladder using protons. In another study of 10 preoperative patients with T3 node negative disease, PBT achieved comparable dose heterogeneity and significant dose reductions to the femoral heads, sigmoid/colon, pelvic bone, bladder, bowel, and normal tissue compared to IMRT and 3DCRT (48). Notably, small bowel receiving 15 Gy was significantly reduced to 90 cc compared to 157 and 138 cc for 3DCRT and IMRT, respectively. For patients with residual disease, a case report of a 65-year-old patient treated at Massachusetts General Hospital following initial abdominoperineal resection (APR) for moderately differentiated rectal adenocarcinoma showed that protons reduced the dose of small bowel receiving 50 Gy by 50%, while still permitting 55 Gy to the target volume (49). Despite these encouraging findings, other reports suggest a more modest benefit using PBT. Cooper et al. found no difference when neoadjuvant 3DCRT and volumetric modulated arc therapy (VMAT) plans were compared to passive scatter PBT plans (45 Gy with 5.4 Gy boost) in 4 patients with resectable (T2–4,N0–2) disease (50). Differences in mean V10–V40Gy bowel volumes or V40–V50Gy bladder volumes were not significant, though the authors note a trend toward decreased exposure at the V10–V20Gy bowel levels using PBT.
For cases of very advanced, nonresectable (cT4) rectal cancers, data come from 2 Swedish planning studies. One group designed spot scanning PBT plans using two posterior beams and a peripheral boost to 62.5 Gy in 25 fractions (51). In line with Colaco and Wolff (46,47), protons achieved acceptable target coverage using a peripheral boost and significantly spared the small intestine in 5 out of 7 patients. Mean intestinal doses were 17.8 vs. 31.1 Gy for PBT and VMAT, respectively, suggesting that dose escalation may be possible with protons as a method for downstaging and to increase local control. Likewise, Isacsson and colleagues found that PBT significantly reduced the low-dose bath to the small bowel and other OARs in 6 patients with unresectable primary rectal adenocarcinoma when compared to photon plans (52). PBT plans used three beams to deliver a PTV boost and cover the primary tumor and adjacent lymph nodes. At a 5% normal tissue complication probability (NTCP), the absolute mean tumor control probability (TCP) increased by 14 percentage points, suggesting that PBT may be beneficial for bulky tumors.
Unfortunately, the majority of PBT studies in rectal cancer lack clinical toxicity data and it is therefore unclear whether statistically significant differences in dose distributions translate to meaningful differences in acute and late toxicity. Clinical toxicity data are derived primarily from Japanese retrospective reviews and case studies of patients with recurrent disease. One group retrospectively reviewed 13 consecutive patients who received 70 Gy PBT in 25 fractions for locally recurrent rectal cancer (LRRC) that was either unresectable or that patients had refused to remove surgically (53). At a median follow-up of approximately 3.5 years, the local control rate and median PFS were 46% and 414 days (range, 58–2,105 days), respectively. Grade 3 or 4 toxicity developed in one patient with urinary obstruction, and there were no treatment-related deaths. Similar findings were reported at the Hyogo Ion Beam Medical Center which treated 2 patients with PBT (74 Gy/37 F) for LRRC (stage IIIA). Both had previously received upfront surgery with curative intent. Grade 1 dermatitis occurred in 1 patient. At 2-year follow-up, 1 patient remains recurrence free while the other re-recurred and passed away 6 years after PBT (54). A third case study reports partial response and no adverse effects at 3-year follow up after an 85-year-old man was treated with 67.5 Gy in 25 fractions for a 28 mm solitary lymph node metastasis (55). The patient had previously undergone curative surgery for primary rectosigmoid cancer.
Consistent with this data, researchers at the University of Pennsylvania report an improved toxicity profile for PBT in patients with recurrent disease (56). Seven patients with a KPS of 60 who previously received 50.4 Gy of RT underwent double-scatter PBT using 1–3 treatment fields. Mean PBT dose was 61.2 Gy (range, 45–64.8 Gy) and the maximum total dose sum of radiation was 109.8 Gy (range, 95.4–151.2 Gy). At a median follow-up of 19 months, 57% of patients had a durable partial or complete radiographic response and 4 were still alive. Among the deceased, 2 had local recurrence and 1 had brain metastasis. Compared to VMAT, bowel exposure at the V10Gy and V20Gy levels was significantly reduced by 85% and 55%, respectively. Doses to 200 and 150 cm3 of bowel were also reduced. Grade 3 acute toxicity was observed in 3 patients (1 abdominal pain and 3 diarrhea) and small bowel obstruction developed in 2 patients.
Taken together these early findings suggest that PBT is both feasible and likely offers at least comparable outcomes to conventional therapies for rectal cancer. However, clinical toxicity data derives from limited studies of patients treated for recurrent disease. There remains a need for prospective PBT outcome studies in patients with primary disease.
Anal cancer
As seen with rectal cancer, a number of treatment-planning studies suggest improved dosimetry with PBT for anal cancer. In a study by Anand et al., scanning PBT plans reduced mean doses to bone marrow, bladder, small bowel, and genitalia by an average of 52%, 60%, 55.4%, and 97.6%, respectively, in 8 patients with SCCA (57). Bone marrow V10–V30Gy and small bowel V30Gy were also significantly reduced, suggesting that hematologic and GI toxicity may benefit from PBT. Bone marrow and small bowel doses also improved in another planning study of 4 patients with SCCA (58). Plans were designed for a prescribed dose of 64 Gy using 3- and 4-field PBT. The small bowel V40Gy was 667, 522, and 535 cc for VMAT, 3-field PBT, and 4-field PBT, respectively (P<0.05). 3- and 4-field PBT significantly reduced the mean doses to both femoral heads by more than 50%, however, sacral bone exposure at V30Gy and V40Gy was comparable due to the posteriorly arranged beams.
Results from studies of more advanced PBT techniques are emerging. At the University of Pennsylvania, pencil-beam scanning PBT plans via two posterior oblique fields were compared to 7-field IMRT plans in patients with T1–4 disease (59). Patients were prescribed 54 Gy to the primary tumor and 45 Gy to the uninvolved pelvic and inguinal lymph nodes. V15Gy for small bowel, a predictor for GI toxicity (60), was 81 and 151 cc for PBT and IMRT, respectively. The volume of irradiated small bowel was reduced for all values up to 35 Gy. Low dose radiation (30 Gy) to the pelvic bone marrow, femoral heads, genitalia, and bladder was significantly reduced. However, as reported by Kronborg et al., PBT increased the volume of exposed lumbosacral marrow between 20 and 45 Gy due to the posterior arrangement of beams. Another recent planning study from Wo and colleagues at Massachusetts General Hospital also reports good dosimetric outcomes with pencil-beam scanning PBT (Figure 1) (61). In addition to adequate PTV coverage, protons reduced small bowel, large bowel, bladder, femoral heads, and iliac crest volumes between V5–V35Gy. At higher doses, only the femoral heads (V40Gy), bladder (V40, V45, and V50Gy) and iliac crests (V40Gy) benefited from PBT. Consistent with this finding, Martin et al. observed a detrimental increase in bowel exposure at maximum doses using IMPT compared to IMRT (62). However, mean bone marrow dose benefited significantly (20.7 vs. 28.2 Gy, V10Gy 60.2% vs. 92.1%), suggesting that hematologic toxicity may be reduced with IMPT without sacrificing target coverage. In a modeling study, Meier et al. quantified this toxicity benefit (63). The authors’ analysis of IMPT plans found that mean bone marrow dose was significantly lower compared to VMAT (17.42 vs. 30.76 Gy). Based on NTCP modeling, they concluded that grade III or higher hematologic toxicity would be reduced from 40% to <5%. Consistent with the previous studies, they also observed sparing to small bowel (V30Gy and V35Gy), large bowel (V35Gy), and genitalia (V20Gy and V30Gy).
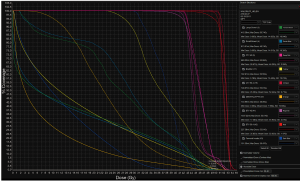
The majority of clinical data on toxicity from PBT comes from a limited number of studies of recurrent disease. One case report from Japan describes outcomes for a 70-year-old woman treated with protons (70 Gy/25 F) for recurrent stage IV anorectal cancer following an APR (64). At 1-year follow-up, there was CR and she remains disease-free 7 years after completing radiation. In a retrospective review, Giap et al. share their early experience with IMPT in 7 recurrent patients previously irradiated for rectal and anal cancer (65). Patients were treated with curative or palliative intent using 50–66 Gy in 1.8–2 Gy fractions prescribed to the GTV and CTV with 1–3 beams. Mean follow up was 18 months. Three patients had CR and 2 patients had partial response. Mean doses to the rectum, femoral heads, bladder, and bowel were 56, 12, 18, and 4 Gy, respectively. Only 1 grade 3 toxicity (ulceration) was reported. These limited studies offer a foundation for future longitudinal trials of protons in patients with recurrent disease.
More recently, an NCI sponsored prospective single arm pilot study reported outcomes of pencil-beam scanning PBT with concurrent 5-FU/MMC in patients with primary disease at Massachusetts General Hospital and the University of Pennsylvania (Figure 2) (66). Twenty-five patients with stage T1–4, N0–3 SCCA were included. Patients with T2N0 disease received 50.4 Gy to the primary tumor and 42 Gy for elective nodal coverage. 54 and 45 Gy were prescribed to the primary tumor and for elective nodal coverage, respectively, in patients with T3–4N0–3 disease. The median time for treatment and duration of breaks was 42 and 0 days, respectively. There were 2 deaths on study which were unrelated to radiation. At a median follow-up of 26 months, the cCR rate and 2-year disease-free survival rate were 88% and 80%, respectively. Toxicity outcomes were benchmarked to results from RTOG 0529 and 9811. Compared to 3D-CRT, grade 3+ hematologic and dermatologic toxicity were reduced 29% and 51%, respectively. Compared to DP-IMRT, grade 3+ hematologic toxicity decreased 24% while grade 3+ dermatologic toxicity increased 4%. Notably, grade 3+ GI toxicity was unchanged compared to 3D-CRT, but increased by 71% compared to DP-IMRT. While pencil-beam scanning PBT was deemed feasible, the benefit over IMRT was variable. The presence of underlying comorbidities such as inflammatory bowel disease in 2 patients and the study’s small sample size make it challenging to draw definitive conclusions. Long-term toxicity data in larger cohorts are needed to confidently assess the merits of PBT for primary SCCA.
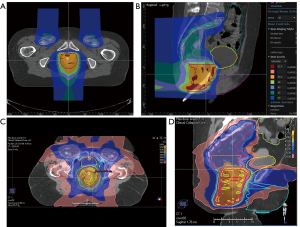
Future directions
To the best of our knowledge, there are no available prospective clinical reports on the use of PBT in the neoadjuvant treatment of rectal cancer. Promising preliminary data in the recurrent setting are available, though limited. A recent prospective study by Wo and colleagues reports early experience using pencil-beam scanning PBT in patients with primary SCCA. These small cohort investigations set the stage for larger prospective studies to investigate in vivo toxicity and tissue tolerance in both the neoadjuvant and recurrent settings. Assessing local control, acute and long-term toxicity, and survival using PBT combined with cytostatics is of particular importance.
Long-term studies of PBT for prostate and breast cancer predict minimal late toxicity and excellent disease control (67,68). In the case of anorectal cancer, PBT may reduce hematologic toxicity compared to IMRT, as suggested by Meier et al. (63). With 30–40% of patients recurring in 10 years and potentially requiring salvage chemotherapy, minimizing bone marrow exposure with PBT may be of substantial benefit (4,69). The decreased side-effect profile may permit safe multimodal therapy, including concurrent or sequential surgery and chemotherapy. As was shown with VMAT over IMRT, PBT may similarly shorten treatment time via fewer treatment breaks due to reduced acute toxicity to small bowel and bone marrow (58,70,71). Reduction in secondary malignancy has also been suggested by benchmark analyses in other cancers (72-74).
In addition to assessing acute toxicity and long-term outcomes, future research should investigate the possibility of dose escalation using PBT. A dose-response relationship was suggested by Allee et al. who found an 11% and 40% local failure rate in patients with microscopic residual disease treated post-operatively with >60 Gy and 50–60 Gy, respectively, for rectal cancer (75). The benefit of dose-escalation for anorectal cancer has been extrapolated from studies suggesting improved tumor response and prognosis in patients with high-grade acute organ toxicity (76,77). By minimizing doses to OARs, PBT may enable safe dose escalation.
In the future, advances in pencil-beam scanning and IMPT may further improve dosimetric advantages of protons (78). Early data show promising reductions to small bowel and bone marrow, suggesting that GI and hematologic toxicity may improve (58,59,61,62). Modeling by Meier et al. shows reduction in grade 3+ hematologic toxicity with IMPT, as implied by other treatment-planning studies (62,63). Long-term clinical studies will be needed to discern the incremental benefit offered by these advanced techniques relative to passive scatter PBT. In addition, planning studies must report the reproducibility of PTV coverage with changes in internal organ motion, bowel gas, patient positioning, and body contour. Proton range is dependent on tissue density and therefore undercoverage of tumor volume or overexposure of OARs may occur. Encouraging preliminary reports from the University of Pennsylvania show less than 2% decrement in target coverage using verification CT scans during pencil-beam scanning PBT (59,79). Minimally invasive interventions such as rectal catheters, dietary changes, and evaluation CTs should be further investigated as potential solutions to improve plan robustness.
Ultimately, any trial design for PBT in anorectal cancer will require a large sample size to discern a benefit. This stems from the multifactorial nature of current treatment paradigms and the modest toxicity improvement described in treatment-planning studies. Future trials must also include careful patient selection criteria. While the presented data indicate that PBT can reliably reproduce dosimetric quality of conventional photon-based plans and reduce doses to OARs, thoughtful selection of patients by tumor stage and patient characteristics will maximize the benefit of protons. For instance, protons may be ideally suited for younger patients, in whom the risk of secondary malignancies and other late complications is of primary concern. Patients with medically inoperable tumors or who are at particularly high risk for acute organ toxicity stand to benefit from PBT. Given the heterogeneity of tumors (e.g., nodal involvement, tumor extension, tumor location), trials must continue to investigate predictive markers that enable identification of patients most appropriate for protons. Lastly, trials should begin to incorporate cost-benefit analyses by including endpoints such as hospitalization rates and quality of life (80). The need for more patient-reported outcomes, in particular, is highlighted by Kronborg and colleagues who found weak agreement between patient and physician-scored toxicity (58).
Conclusions
We here report the currently available data on the use of PBT for rectal and anal cancer. GI malignancies present an exciting realm to actualize the benefit of precision therapy. Several reviews have attempted to summarize the data available (6,81-90). We offer an update to these reports and conclude that there is reason to be optimistic about PBT in select patient populations, especially as IMPT and pencil-beam scanning techniques become more prevalent. Decreased doses to bone marrow and bowel may improve tolerance of multimodal treatment and allow dose escalation, in turn improving clinical and patient-reported outcomes.
Acknowledgments
None.
Footnote
Conflicts of Interest: The authors have no conflicts of interest to declare.
Ethical Statement: The authors are accountable for all aspects of the work in ensuring that questions related to the accuracy or integrity of any part of the work are appropriately investigated and resolved.
References
- Moher D, Liberati A, Tetzlaff J, et al. Preferred reporting items for systematic reviews and meta-analyses: the PRISMA statement. Int J Surg 2010;8:336-41. [Crossref] [PubMed]
- Khan FM. Physics of Radiation Therapy Third Edition. The Journal of the American Medical Association. 2003.
- Randomised trial of surgery alone versus radiotherapy followed by surgery for potentially operable locally advanced rectal cancer. Medical Research Council Rectal Cancer Working Party. Lancet 1996;348:1605-10. [Crossref] [PubMed]
- Sauer R, Becker H, Hohenberger W, et al. Preoperative versus Postoperative Chemoradiotherapy for Rectal Cancer. N Engl J Med 2004;351:1731-40. [Crossref] [PubMed]
- Krook JE, Moertel CG, Gunderson LL, et al. Effective surgical adjuvant therapy for high-risk rectal carcinoma. N Engl J Med 1991;324:709-15. [Crossref] [PubMed]
- Verma V, Lin SH, Simone CB, et al. Clinical outcomes and toxicities of proton radiotherapy for gastrointestinal neoplasms: A systematic review. J Gastrointest Oncol 2016;7:644-64. [Crossref] [PubMed]
- Gastrointestinal Tumor Study Group. Prolongation of the disease-free interval in surgically treated rectal carcinoma. N Engl J Med 1985;312:1465-72. [Crossref] [PubMed]
- Kapiteijn E, Marijnen CA, Nagtegaal ID, et al. Preoperative radiotherapy combined with total mesorectal excision for resectable rectal cancer. N Engl J Med 2001;345:638-46. [Crossref] [PubMed]
- Frykholm GJ, Glimelius B, Påhlman L. Preoperative or postoperative irradiation in adenocarcinoma of the rectum: final treatment results of a randomized trial and an evaluation of late secondary effects. Dis Colon Rectum 1993;36:564-72. [Crossref] [PubMed]
- Påhlman L, Glimelius B. Pre- or postoperative radiotherapy in rectal and rectosigmoid carcinoma. Report from a randomized multicenter trial. Ann Surg 1990;211:187-95. [Crossref] [PubMed]
- Blomqvist L, Glimelius B. The “good”, the “bad”, and the “ugly” rectal cancers. Acta Oncol 2008;47:5-8. [Crossref] [PubMed]
- Chen RC, Mamon HJ, Ancukiewicz M, et al. Dose-volume effects on patient-reported acute gastrointestinal symptoms during chemoradiation therapy for rectal cancer. Int J Radiat Oncol Biol Phys 2012;83:e513-7. [Crossref] [PubMed]
- Ike H, Shimada H, Ohki S, et al. Outcome of total pelvic exenteration for locally recurrent rectal cancer. Hepatogastroenterology 2003;50:700-3. [PubMed]
- Nielsen MB, Rasmussen PC, Lindegaard JC, et al. A 10-year experience of total pelvic exenteration for primary advanced and locally recurrent rectal cancer based on a prospective database. Colorectal Dis 2012;14:1076-83. [Crossref] [PubMed]
- Bosman SJ, Vermeer TA, Dudink RL, et al. Abdominosacral resection: Long-term outcome in 86 patients with locally advanced or locally recurrent rectal cancer. Eur J Surg Oncol 2014;40:699-705. [Crossref] [PubMed]
- Moriya Y, Akasu T, Fujita S, et al. Total pelvic exenteration with distal sacrectomy for fixed recurrent rectal cancer. Surg Oncol Clin N Am 2005;14:225-38. [Crossref] [PubMed]
- Valentini V, Coco C, Picciocchi A, et al. Does downstaging predict improved outcome after preoperative chemoradiation for extraperitoneal locally advanced rectal cancer? A long-term analysis of 165 patients. Int J Radiat Oncol Biol Phys 2002;53:664-74. [Crossref] [PubMed]
- Kaminsky-Forrett MC, Conroy T, Luporsi E, et al. Prognostic implications of downstaging following preoperative radiation therapy for operable T3-T4 rectal cancer. Int J Radiat Oncol Biol Phys 1998;42:935-41. [Crossref] [PubMed]
- Berger C, Muret A, De , Garaud P, et al. Preoperative radiotherapy (RT) for rectal cancer: Predictive factors of tumor downstaging and residual tumor cell density (RTCD): prognostic implications. Int J Radiat Oncol Biol Phys 1997;37:619-27. [Crossref] [PubMed]
- Theodoropoulos G, Wise WE, Padmanabhan A, et al. T-level downstaging and complete pathologic response after preoperative chemoradiation for advanced rectal cancer result in decreased recurrence and improved disease-free survival. Dis Colon Rectum 2002;45:895-903. [Crossref] [PubMed]
- Frykholm G, Glimelius B, Påhlman L. Preoperative irradiation with and without chemotherapy (MFL) in the treatment of primarily non-resectable adenocarcinoma of the rectum. Results from two consecutive studies. Eur J Cancer Clin Oncol 1989;25:1535-41. [Crossref] [PubMed]
- Minsky BD, Cohen AM, Kemeny N, et al. Enhancement of radiation-induced downstaging of rectal cancer by fluorouracil and high-dose leucovorin chemotherapy. J Clin Oncol 1992;10:79-84. [Crossref] [PubMed]
- Wiltshire KL, Ward IG, Swallow C, et al. Preoperative radiation with concurrent chemotherapy for resectable rectal cancer: Effect of dose escalation on pathologic complete response, local recurrence-free survival, disease-free survival, and overall survival. Int J Radiat Oncol Biol Phys 2006;64:709-16. [Crossref] [PubMed]
- Mohiuddin M, Regine WF, John WJ, et al. Preoperative chemoradiation in fixed distal rectal cancer: Dose time factors for pathological complete response. Int J Radiat Oncol Biol Phys 2000;46:883-8. [Crossref] [PubMed]
- Nigro ND, Vaitkevicius VK, Considine B. Combined therapy for cancer of the anal canal: a preliminary report. Dis Colon Rectum 1974;17:354-6. [Crossref] [PubMed]
- Northover J, Glynne-Jones R, Sebag-Montefiore D, et al. Chemoradiation for the treatment of epidermoid anal cancer: 13-year follow-up of the first randomised UKCCCR Anal Cancer Trial (ACT I). Br J Cancer 2010;102:1123-8. [Crossref] [PubMed]
- Glynne-Jones R, Sebag-Montefiore D, Adams R, et al. “Mind the gap” - The Impact of variations in the duration of the treatment gap and overall treatment time in the first UK anal cancer trial (ACT I). Int J Radiat Oncol Biol Phys 2011;81:1488-94. [Crossref] [PubMed]
- Gunderson LL, Winter KA, Ajani JA, et al. Long-term update of US GI intergroup RTOG 98-11 Phase III trial for anal carcinoma: Survival, relapse, and colostomy failure with concurrent chemoradiation involving fluorouracil/mitomycin versus fluorouracil/cisplatin. J Clin Oncol 2012;30:4344-51. [Crossref] [PubMed]
- Gunderson LL, Moughan J, Ajani JA, et al. Anal carcinoma: Impact of TN category of disease on survival, disease relapse, and colostomy failure in US gastrointestinal intergroup RTOG 98-11 phase 3 trial. Int J Radiat Oncol Biol Phys 2013;87:638-45. [Crossref] [PubMed]
- Ellis RE. The distribution of active bone marrow in the adult. Phys Med Biol 1961;5:255-8. [Crossref] [PubMed]
- Flam M, John M, Pajak TF, et al. Role of mitomycin in combination with fluorouracil and radiotherapy, and of salvage chemoradiation in the definitive nonsurgical treatment of epidermoid carcinoma of the anal canal: Results of a phase III randomized intergroup study. J Clin Oncol 1996;14:2527-39. [Crossref] [PubMed]
- Das P, Cantor SB, Parker CL, et al. Long-term quality of life after radiotherapy for the treatment of anal cancer. Cancer 2010;116:822-9. [Crossref] [PubMed]
- Welzel G, Hägele V, Wenz F, et al. Quality of life outcomes in patients with anal cancer after combined radiochemotherapy. Strahlenther Onkol 2011;187:175-82. [Crossref] [PubMed]
- Kachnic LA, Winter K, Myerson RJ, et al. RTOG 0529: A phase 2 evaluation of dose-painted intensity modulated radiation therapy in combination with 5-fluorouracil and mitomycin-C for the reduction of acute morbidity in carcinoma of the anal canal. Int J Radiat Oncol Biol Phys 2013;86:27-33. [Crossref] [PubMed]
- Ghosn M, Kourie HR, Abdayem P, et al. Anal cancer treatment: Current status and future perspectives. World J Gastroenterol 2015;21:2294-302. [Crossref] [PubMed]
- Osborne MC, Maykel J, Johnson EK, et al. Anal squamous cell carcinoma: An evolution in disease and management. World J Gastroenterol 2014;20:13052-9. [Crossref] [PubMed]
- Dasgupta T, Rothenstein D, Chou JF, et al. Intensity-modulated radiotherapy vs. conventional radiotherapy in the treatment of anal squamous cell carcinoma: a propensity score analysis. Radiother Oncol 2013;107:189-94. [Crossref] [PubMed]
- Olivatto LO, Cabral V, Rosa A, et al. Mitomycin-C- or cisplatin-based chemoradiotherapy for anal canal carcinoma: Long-term results. Int J Radiat Oncol Biol Phys 2011;79:490-5. [Crossref] [PubMed]
- Ben-Josef E, Moughan J, Ajani JA, et al. Impact of overall treatment time on survival and local control in patients with anal cancer: A pooled data analysis of radiation therapy oncology group trials 87-04 and 98-11. J Clin Oncol 2010;28:5061-6. [Crossref] [PubMed]
- Allal AS, Mermillod B, Roth AD, et al. The impact of treatment factors on local control in T2-T3 anal carcinomas treated by radiotherapy with or without chemotherapy. Cancer 1997;79:2329-35. [Crossref] [PubMed]
- Weber DC, Kurtz JM, Allal AS. The impact of gap duration on local control in anal canal carcinoma treated by split-course radiotherapy and concomitant chemotherapy. Int J Radiat Oncol Biol Phys 2001;50:675-80. [Crossref] [PubMed]
- Graf R, Wust P, Hildebrandt B, et al. Impact of overall treatment time on local control of anal cancer treated with radiochemotherapy. Oncology 2003;65:14-22. [Crossref] [PubMed]
- Constantinou EC, Daly W, Fung CY, et al. Time-dose considerations in the treatment of anal cancer. Int J Radiat Oncol Biol Phys 1997;39:651-7. [Crossref] [PubMed]
- Widder J, Kastenberger R, Fercher E, et al. Radiation dose associated with local control in advanced anal cancer: Retrospective analysis of 129 patients. Radiother Oncol 2008;87:367-75. [Crossref] [PubMed]
- Huang K, Haas-Kogan D, Weinberg V, et al. Higher radiation dose with a shorter treatment duration improves outcome for locally carcinoma of anal canal. World J Gastroenterol 2007;13:895-900. [Crossref] [PubMed]
- Colaco RJ, Nichols RC, Huh S, et al. Protons offer reduced bone marrow, small bowel, and urinary bladder exposure for patients receiving neoadjuvant radiotherapy for resectable rectal cancer. J Gastrointest Oncol 2014;5:3-8. [PubMed]
- Wolff HA, Wagner DM, Conradi LC, et al. Irradiation with protons for the individualized treatment of patients with locally advanced rectal cancer: A planning study with clinical implications. Radiother Oncol 2012;102:30-7. [Crossref] [PubMed]
- Palmer M, Mok H, Ciura K, et al. Dose Reduction to Small Bowel and Other Relevant Structures in Rectal Carcinoma With Proton Therapy. Int J Radiat Oncol 2012;84:S846. [Crossref]
- Tatsuzaki H, Urie MM, Willett CG. 3-D comparative study of proton vs. x-ray radiation therapy for rectal cancer. Int J Radiat Oncol Biol Phys 1992;22:369-74. [Crossref] [PubMed]
- Cooper BT, Qu J, Chon BH, et al. Dosimetric Comparison of Proton Therapy, Volumetric Modulated Arc Therapy, and 3-D Conformal Radiation Therapy for the Treatment of Rectal Cancer: An Early Community Experience. Int J Radiat Oncol Biol Phys 2015;93:E176. [Crossref]
- Radu C, Norrlid O, Brændengen M, et al. Integrated peripheral boost in preoperative radiotherapy for the locally most advanced non-resectable rectal cancer patients. Acta Oncol 2013;52:528-37. [Crossref] [PubMed]
- Isacsson U, Montelius A, Jung B, et al. Comparative treatment planning between proton and X-ray therapy in locally advanced rectal cancer. Radiother Oncol 1996;41:263-72. [Crossref] [PubMed]
- Hamauchi S., Yamazaki K., Yasui H., et al. Safety and efficacy of proton-beam radiation therapy for patients with locally recurrent rectal cancer. Ann Oncol 2012;23:xi132-67. [Crossref]
- Mokutani Y, Yamamoto H, Uemura M, et al. Effect of particle beam radiotherapy on locally recurrent rectal cancer: Three case reports. Mol Clin Oncol 2015;3:765-9. [Crossref] [PubMed]
- Murata K, Kitahara T, Nushijima Y, et al. An 85-Year-Old Man with Lymph Node Metastasis of Recurrent Rectal Cancer Treated Using Proton Beam Therapy. Gan To Kagaku Ryoho 2016;43:1473-5. [PubMed]
- Berman AT, Both S, Sharkoski T, et al. Proton Reirradiation of Recurrent Rectal Cancer: Dosimetric Comparison, Toxicities, and Preliminary Outcomes. Int J Part Ther 2014;1:2-13. [Crossref]
- Anand A, Bues M, Rule WG, et al. Scanning proton beam therapy reduces normal tissue exposure in pelvic radiotherapy for anal cancer. Radiother Oncol 2015;117:505-8. [Crossref] [PubMed]
- Kronborg C, Serup-Hansen E, Lefevre A, et al. Prospective evaluation of acute toxicity and patient reported outcomes in anal cancer and plan optimization. Radiother Oncol 2018;128:375-9. [Crossref] [PubMed]
- Ojerholm E, Kirk ML, Thompson RF, et al. Pencil-beam scanning proton therapy for anal cancer: A dosimetric comparison with intensity-modulated radiotherapy. Acta Oncol 2015;54:1209-17. [Crossref] [PubMed]
- Fiorino C, Alongi F, Perna L, et al. Dose-Volume Relationships for Acute Bowel Toxicity in Patients Treated With Pelvic Nodal Irradiation for Prostate Cancer. Int J Radiat Oncol Biol Phys 2009;75:29-35. [Crossref] [PubMed]
- Wo JY, Depauw N, Symonifka J, et al. Dosimetric Analysis of Proton Pencil Beam Scanning Radiation Therapy Versus Dose Painted Intensity Modulated Radiation Therapy for Squamous Cell Carcinoma of the Anal Canal. Int J Radiat Oncol 2014;90:S398. [Crossref]
- Martin R, Williamson T, Yepes P, et al. Scientific Abstracts and Sessions. Med Phys 2018;45:e120-706. [Crossref]
- Meier T, Mascia A, Wolf E, et al. Modeling of High-Grade Hematologic Toxicity in Anal Cancer Patients Treated With Intensity Modulated Proton Therapy (IMPT) and Volumetric Modulated Arc Therapy (VMAT). Int J Radiat Oncol 2016;96:E145. [Crossref]
- Ie M, Yamaguchi T, Kinugasa Y, et al. Complete response of locally recurrent anorectal cancer to proton beam therapy alone--a case report. Gan To Kagaku Ryoho 2014;41:2623-5. [PubMed]
- Giap F, Lepage R, Dong L, et al. Intensity Modulated Proton Therapy for the Retreatment of Rectal and Anal Cancer. Int J Radiat Oncol 2017;99:E149. [Crossref]
- Wo JY, Plastaras JP, Yeap BY, et al. A Pilot Feasibility Study of Definitive Concurrent Chemoradiation with Pencil Beam Scanning Proton Beam in Combination with 5-Fluorouracil and Mitomycin-C for Carcinoma of the Anal Canal. J Clin Oncol 2018;36:733. [Crossref]
- Bush DA, Slater JD, Garberoglio C, et al. Partial breast irradiation delivered with proton beam: Results of a phase II trial. Clin Breast Cancer 2011;11:241-5. [Crossref] [PubMed]
- Nihei K, Ogino T, Onozawa M, et al. Multi-institutional phase II study of proton beam therapy for organ-confined prostate cancer focusing on the incidence of late rectal toxicities. Int J Radiat Oncol Biol Phys 2011;81:390-6. [Crossref] [PubMed]
- Sauer R, Liersch T, Merkel S, et al. Preoperative versus postoperative chemoradiotherapy for locally advanced rectal cancer: results of the German CAO/ARO/AIO-94 randomized phase III trial after a median follow-up of 11 years. J Clin Oncol 2012;30:1926-33. [Crossref] [PubMed]
- Stieler F, Wolff D, Lohr F, et al. A fast radiotherapy paradigm for anal cancer with volumetric modulated arc therapy (VMAT). Radiat Oncol 2009;4:48. [Crossref] [PubMed]
- Vieillot S, Azria D, Lemanski C, et al. Plan comparison of volumetric-modulated arc therapy (RapidArc) and conventional intensity-modulated radiation therapy (IMRT) in anal canal cancer. Radiat Oncol 2010;5:92. [Crossref] [PubMed]
- Simone CB, Kramer K, O’Meara WP, et al. Predicted rates of secondary malignancies from proton versus photon radiation therapy for stage I seminoma. Int J Radiat Oncol Biol Phys 2012;82:242-9. [Crossref] [PubMed]
- Fontenot JD, Lee AK, Newhauser WD. Risk of Secondary Malignant Neoplasms From Proton Therapy and Intensity-Modulated X-Ray Therapy for Early-Stage Prostate Cancer. Int J Radiat Oncol Biol Phys 2009;74:616-22. [Crossref] [PubMed]
- Schneider U, Lomax A, Pemler P, et al. The impact of IMRT and proton radiotherapy on secondary cancer incidence. Strahlenther Onkol 2006;182:647-52. [Crossref] [PubMed]
- Allee PE, Tepper JE, Gunderson LL, et al. Postoperative radiation therapy for incompletely resected colorectal carcinoma. Int J Radiat Oncol Biol Phys 1989;17:1171-6. [Crossref] [PubMed]
- Wolff HA, Raus I, Jung K, et al. High-grade acute organ toxicity as a positive prognostic factor in primary radiochemotherapy for anal carcinoma. Int J Radiat Oncol Biol Phys 2011;79:1467-78. [Crossref] [PubMed]
- Wolff HA, Bosch J, Jung K, et al. High-grade acute organ toxicity as positive prognostic factor in primary radio(chemo)therapy for locally advanced, inoperable head and neck cancer. Strahlenther Onkol 2010;186:262-8. [Crossref] [PubMed]
- Lomax A. Intensity modulation methods for proton radiotherapy. Phys Med Biol 1999;44:185-205. [Crossref] [PubMed]
- Dionisi F, Batra S, Kirk M, et al. Pencil Beam Scanning Proton Therapy in the Treatment of Rectal Cancer. Int J Radiat Oncol 2013;87:S341-2. [Crossref]
- Kerstiens J, Johnstone PAS. Proton therapy expansion under current United States reimbursement models. Int J Radiat Oncol Biol Phys 2014;89:235-40. [Crossref] [PubMed]
- Meyer J, Czito B, Yin FF, et al. Advanced radiation therapy technologies in the treatment of rectal and anal cancer: Intensity-modulated photon therapy and proton therapy. Clin Colorectal Cancer 2007;6:348-56. [Crossref] [PubMed]
- Julie DR, Goodman KA. Advances in the Management of Anal Cancer. Curr Oncol Rep 2016;18:20. [Crossref] [PubMed]
- Raldow AC, Hong TS. Will There Be a Clinically Significant Role for Protons in Patients With Gastrointestinal Malignancies? Semin Radiat Oncol 2018;28:125-30. [Crossref] [PubMed]
- Plastaras JP, Dionisi F, Wo JY. Gastrointestinal cancer: Nonliver proton therapy for gastrointestinal cancers. Cancer J 2014;20:378-86. [Crossref] [PubMed]
- Baskar R, Lee KA, Yeo R, et al. Cancer and radiation therapy: Current advances and future directions. Int J Med Sci 2012;9:193-9. [Crossref] [PubMed]
- Doyen J, Falk AT, Floquet V, et al. Proton beams in cancer treatments: Clinical outcomes and dosimetric comparisons with photon therapy. Cancer Treat Rev 2016;43:104-12. [Crossref] [PubMed]
- Allen AM, Pawlicki T, Dong L, et al. An evidence based review of proton beam therapy: The report of ASTRO’s emerging technology committee. Radiother Oncol 2012;103:8-11. [Crossref] [PubMed]
- Das P. Rectal cancer: Do protons have prospects? J Gastrointest Oncol 2014;5:1-2. [PubMed]
- Joye I, Haustermans K. Which Patients With Rectal Cancer Do Not Need Radiotherapy? Semin Radiat Oncol 2016;26:199-204. [Crossref] [PubMed]
- Hajj C, Goodman KA. Role of radiotherapy and newer techniques in the treatment of GI cancers. J Clin Oncol 2015;33:1737-44. [Crossref] [PubMed]