State-of-the-art molecular imaging in esophageal cancer management: implications for diagnosis, prognosis, and treatment
Introduction
An estimated 18,170 new cases of esophageal cancer will be diagnosed in 2014 and approximately 15,450 of these patients will die from the disease (1). Although outcomes are improved with the addition of neoadjuvant chemotherapy or neoadjuvant chemoradiation (CRT) to surgery alone, outcomes for locally advanced esophageal cancer remain poor (2). Local failure rates even with the addition of CRT exceed 50% (3,4) in locally advanced patients.
The era of personalized medicine has brought increasing awareness that variations in tumor biology drive tumor genesis, response to treatment, and long-term prognosis. The advent of molecular imaging techniques has resulted in improvements in esophageal cancer staging and treatment. Although 18F-fluorodeoxyglucose (18F-FDG) positron emission tomography (PET) is the most commonly studied and clinically used approach, early results using other molecular imaging techniques suggest that further improvements in esophageal cancer care are possible.
Molecular imaging agents
18F-FDG is the most commonly used agent for PET imaging. However, its sensitivity for very small tumors is low, and uptake is dependent on oxygen supply and glycolysis (5). Choline-derivatives, such as 11C-choline, 18F-fluoroethylcholine, and 18F-fluorocholine have been investigated because of their more selective uptake in the mediastinum (6,7). Choline is a precursor in the biosynthesis of phosphatidylcholine, which is a major phospholipid constituent of the cell membrane; consequently, choline uptake is proportional to the rate of cell division. One advantage is that normal tissues, such as brain, lung, heart, bone, and skeletal muscle, have very low uptake of positron-labeled cholines. The more pronounced uptake in malignant mediastinal adenopathy is more striking when compared against low uptake in the lung, heart, and mediastinum. Another benefit of choline-derivatives is the rapid clearance of radiolabeled-choline from the blood after intravenous administration, allowing for quick initiation of PET imaging, as soon as 2-3 min after radiotracer injection (6). Tian et al. compared 11C-choline PET with 18F-FDG PET in 38 patients with various tumors and found a high correlation in differentiation between malignant and benign lesion uptake (8). These researchers also observed differences in imaging acquisition timing, with PET performed 5 min after injection of 11C-choline and 40 min after injection of 18F-FDG. One important logistic limitation of 11C-choline is a short half-life (20 min), which limits its use to facilities with an onsite cyclotron (8). 18F-fluorocholine has a longer lived isotope, with a half-life of 110 min and has shown encouraging results with high tumor-to-background contrast within minutes of injection (9). The relatively low uptake of choline in normal brain tissue allows for good delineation of disease in the brain, and patients can be scanned within 20 min after intravenous injection. However, normal uptake of choline in the liver may potentially obscure identification of metastatic disease below the diaphragm (9).
L-[3-18F]-α-methyltyrosine (18F-FAMT) is an amino acid tracer developed for PET imaging. 18F-FAMT is accumulated in tumor cells via an amino acid transport system, LAT-1, which plays an important role in cellular proliferation and is widely expressed in cancers, particularly in squamous cell carcinoma (SCC) (10). In oral SCC, uptake of 18F-FAMT has been significantly correlated with LAT-1 expression, cell proliferation, maximal tumor size, and disease stage and is more specific for malignancy than 18F-FDG (10). In a study of 21 patients with esophageal SCC, 18F-FAMT demonstrated lower sensitivity for lymph node staging than 18F-FDG (40% and 47%, respectively) but significantly higher specificity (100% and 50%, respectively) (11). 18F-FAMT may also allow for better delineation of malignancy near the heart, because it does not show the intense cardiac physiologic uptake of 18F-FDG. Use of 18F-FAMT in conjunction with 18F-FDG in PET may help reduce false positives resulting from inflammation. Further studies are needed to establish the relationship between intensity of uptake and patient prognosis with 18F-FAMT.
18F-fluorothymidine (18F-FLT) is a pyrimidine analog that is phosphorylated by thymidine kinase 1, an enzyme within the salvage DNA synthesis pathway. The activity of thymidine kinase 1 and therefore uptake of 18F-FLT reflects cellular proliferation and is more specific than 18F-FDG for differentiating neoplasms from inflammation (12). In a study of 22 patients, both uptake and sensitivity for detection of lymph node metastatic disease were lower with 18F-FLT than 18F-FDG (75% and 83%, respectively), but specificity was higher (99% and 96%, respectively) (13). 18F-FLT has also shown promise in monitoring disease response to treatment (12). The major disadvantage of 18F-FLT is increased risk of false negative results when used alone. 18F-FDG remains the most widely used radiolabeled agent for staging and evaluating treatment response, but other molecular agents continue to be developed and assessed in clinical trials.
Diagnosis of premalignant esophageal lesions
The rate of esophageal cancer diagnosis, particularly in the lower esophagus and gastroesophageal junction (GEJ), has increased dramatically in recent years. One driver of this increase is the rising incidence of chronic gastroesophageal reflux disease (GERD), which leads to Barrett esophagus (BE). In BE, the normal stratified squamous epithelium of the esophagus is replaced by simple columnar epithelium with goblet cells. BE is associated with an increased risk of esophageal adenocarcinoma and is therefore considered a premalignant disease. Endoscopic surveillance is recommended for patients with BE in order to detect neoplastic changes at an early stage. Early detection of progression to malignancy can allow for more limited treatment and result in improved long-term outcomes.
Endoscopy alone for BE surveillance is not ideal because it cannot reliably detect regions of dysplasia. The flat appearance of dysplasia makes it difficult to visualize, despite the advantages accrued with narrow-band imaging, high-yield white-light endoscopy, and chromoendoscopy (14). Only a limited amount of tissue is evaluated with standard random biopsies in BE, allowing areas of dysplasia or invasive carcinoma to remain undetected (15).
Several molecular imaging techniques have been developed in an attempt to increase detection of subtle dysplastic changes within BE. Sturm et al. developed a peptide that binds to regions of esophageal high-grade dysplasia as well as adenocarcinoma (16). First-in-human results demonstrated that the peptide was not only safe but also appeared to effectively enhance identification of esophageal neoplasia. Confocal endomicroscopy in 25 patients was performed after topical administration of the peptide, resulting in a 3.8-fold higher fluorescence intensity in regions of high grade dysplasia and esophageal adenocarcinoma than in BE and normal squamous epithelium. This peptide may therefore allow for more directed and higher yield biopsies. Another technique, probe-based confocal microscopy, uses biophotonics to visualize cellular details during endoscopy (17). Its capabilities for real-time assessment could save significant time and cost associated with biopsy analysis. Other promising molecular imaging strategies include gene expression analysis of stem cell clones from BE biopsy specimens (18,19), targeted proteomics (20-22), and mass spectrometry (23,24).
Esophageal cancer staging
Before routine clinical use of 18F-FDG PET, computed tomography (CT) and endoscopic ultrasound (EUS) were the primary imaging modalities for esophageal cancer staging. These techniques have proven to be complementary; in many patients, CT is better able to determine tumor length and exclude invasion of adjacent structures whereas EUS can better determine the depth of invasion of the primary tumor and identify locoregional lymph node metastasis (25,26). A meta-analysis by Rösch et al. reported that the accuracy of EUS for staging the extent of primary tumor involvement was 89% (27).
In contrast to EUS, 18F-FDG PET is less successful in accurately determining the depth of invasion of the primary lesion (26). 18F-FDG PET does not clearly offer a significant benefit in nodal staging over EUS and CT (28). Significant 18F-FDG uptake in the primary lesion may obscure increased uptake in locoregional nodes (29). However, 18F-FDG PET is particularly useful as a complementary imaging tool for detecting distant metastases, which are quite common in patients with esophageal cancer (25,30-32). A study by Lowe et al. in 75 newly diagnosed esophageal cancer patients reported the respective sensitivity and specificity for distant metastases to be 81% and 91% for PET, 81% and 82% for CT, and 73% and 86% for EUS (26). A meta-analysis showed that the sensitivity and specificity for detecting distant metastases were 71% and 93%, respectively, for 18F-FDG PET and 52% and 91%, respectively, for CT (25). The superior ability of 18F-FDG PET in detection of occult distant metastasis during the initial staging process may provide sufficient evidence to avoid unnecessary surgery in up to 20% of patients (32,33). A multicenter prospective cohort study of 491 patients showed that PET/CT led to clinically significant changes in stage for 24% of patients (34). The American College of Surgeons Oncology Group Z0060 trial prospectively evaluated the utility of 18F-FDG PET after standard staging workup by randomizing 262 potentially resectable esophageal cancer patients after CT to either 18F-FDG PET or no PET imaging (35). 18F-FDG PET identified biopsy-proven distant metastasis not detected by CT in 4.8% of patients who proceeded to surgery. An additional 9.5% of patients had PET-detected metastases that were not biopsy-proven. Of note, PET/CT coregistration was not performed in this trial.
Integrated PET/CT has higher sensitivity and specificity for tumor staging than 18F-FDG PET alone (36). In fused scans, the CT has two main purposes. The first is to serve as an attenuation map to correct for the fact that photons originating from deeper structures are more highly attenuated that those originating closer to the skin surface. This correction is essential not only to improve image quality but to allow for accurate quantitative measurements of metabolic activity performed using the standardized uptake value (SUV). The SUV is the ratio of metabolic activity (Bq/mL) in the region of interest to the decay-corrected activity of injected 18F-FDG (Bq/g). The second purpose is to provide anatomic and structural reference data that complements the metabolic findings on PET imaging, fusing form (anatomic) and function (metabolic) information.
18F-FLT may offer significant imaging advantages over 18F-FDG for esophageal cancer staging with PET. One of the primary disadvantages of 18F-FDG is its nonspecific uptake within benign lesions, which may result in inappropriate upstaging of patients (37). 18F-FLT has higher uptake in proliferating tumors and better discrimination between malignant and benign lesions, as shown in both in vitro and in vivo studies (38). Han et al. compared the abilities of 18F-FLT and 18F-FDG PET in detection of regional lymph node metastasis in 22 patients with SCC of the esophagus using pathologic findings (13). Only three false-positive nodes were found using 18F-FLT, whereas 18F-FDG PET identified 14. The sensitivity and specificity of 18F-FLT PET were 74% and 99%, respectively, and of 18F-FDG PET were 83% and 96%, respectively. However, 18F-FLT may result in a higher rate of false-negative results, as suggested by van Westreenen et al. (39). Additional work is needed to evaluate the benefits of 18F-FLT in esophageal cancer staging, and 18F-FDG remains the current agent of choice.
Pretreatment 18F-FDG PET and prognosis
18F-FDG PET is not only useful for staging but may be effective in determining prognosis prior to treatment. The first report of 18F-FDG PET in prognosis for esophageal cancer was in 1998, when Fukunaga et al. reported that patients with tumor SUV >7 had poorer outcomes (40). This correlation between higher maximum SUV (SUVmax) and worse overall and disease-free survival (OS and DFS, respectively) has since been supported by numerous studies (41-46). A literature review by Omloo et al. reported that 12 of 15 studies included in their analysis showed that pretreatment SUV is a predictor for survival in univariate analysis (46). However, only 2 studies showed that this significance persisted in multivariate analysis. Furthermore, it is unclear whether SUVmax is an independent prognostic factor when compared with tumor stage (46,47). Although pretreatment SUV may be prognostic, a wide range of SUVmax thresholds have been reported as being significant. For example, significant survival differences were shown by Rizk et al. (41), who used a SUVmax threshold of 4.5, whereas Cerfolio and Bryant suggested 6.6 as an ideal threshold (42). Better characterization of SUVmax thresholds in this disease setting is needed to better evaluate and apply the prognostic utility of this PET parameter.
The majority of 18F-FDG PET studies define therapeutic response by quantifying the SUVmax of the tumor (Table 1). However, this metric does not account for the significant heterogeneity of 18F-FDG tumor uptake or account for the fact that many tumors have both malignant and nonmalignant components. Spatial 18F-FDG PET features such as tumor volume (57), tumor shape (58), and texture features (59) have been suggested to be more informative than SUVmax. Investigators also have evaluated metabolic tumor volume (MTV), or the volume of tumor with increased glycolytic activity above a specified SUV threshold, because it includes both anatomic tumor burden and metabolic information. Just as no standardized thresholds are agreed upon for SUVmax, various MTV definitions have been used; thus, it is difficult to compare studies and evaluate the usefulness of MTV. Emerging data suggest that MTV may be a significant predictor for survival, and perhaps may be more powerful than SUVmax. In 2010, Hyun et al. were the first to report the use of MTV in 151 esophageal cancer patients, most with SCC (60). Although SUVmax and MTV were each significant predictors of survival in univariate analysis, only MTV was significant in multivariate analysis, along with T and M stage. Chen et al. recently studied 90 patients with locally advanced SCC of the esophagus who received definitive CRT and underwent a pretreatment 18F-FDG PET scan (61). These researchers reported that MTV 20% (tumor volume with at least 20% of SUVmax) >40 mL was the only significant predictor of survival in multivariate analysis. They also evaluated MTV2.5 (tumor volume with SUVmax ≥2.5), which was not significant. Another 18F-FDG PET parameter is total lesion glycolysis (TLG), defined as the MTV multiplied by the mean SUV (SUVmean). Larger TLG values are believed to reflect increased amounts of hypoxia resulting from larger amounts of tumor being in glycolysis. Although data are limited with respect to TLG and esophageal cancer, a recent report by Li et al. suggests that TLG may be a useful prognostic factor (62).
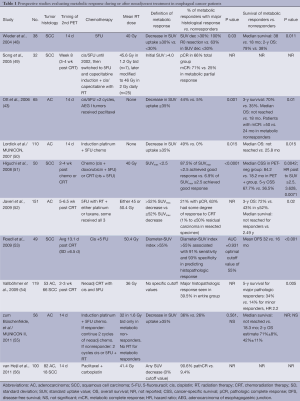
Full table
Current literature suggests that these pretreatment 18F-FDG PET parameters are promising prognostic factors, but further validation is warranted. If these parameters are to become widely used in the clinic, standardization is critical (63).
18F-FDG PET for radiation treatment planning
Gross disease must be accurately delineated in esophageal cancer patients who receive radiation therapy. This is particularly important when highly conformal radiation delivery techniques, such as intensity-modulated radiation therapy (IMRT), are used. Clearly distinguishing primary tumor from normal esophagus is challenging with CT alone. Using the assumption that the primary esophageal tumor volume identified by EUS was accurate, CT was found to routinely underestimate or overestimate the proximal and/or distal extent of the tumor by several centimeters (64). Thus, investigators have looked to 18F-FDG PET to aid in more accurately defining the gross tumor volume (GTV) for esophageal cancer patients. Incorporation of 18F-FDG PET has proven useful in radiation planning for other disease sites, such as lung (65,66), lymphoma (67), and head and neck (68).
The impact of 18F-FDG PET on radiation treatment planning for esophageal cancer has been evaluated retrospectively and prospectively (69-71). Leong et al. studied 21 esophageal cancer patients scheduled to receive definitive CRT (69). Two GTVs were contoured, one using CT alone (GTV-CT) and another using both PET and CT. When the contours were compared, a portion of PET-avid disease was excluded in 69% of the GTV-CTs, which would have led to a “geographic miss” in 31% of patients. As expected, the proximal and distal extents differed in the majority of patients. However, the radiographic tumor extent was not confirmed pathologically. Moureau-Zabotto et al. also prospectively evaluated the use of 18F-FDG PET in radiation treatment planning in 34 esophageal cancer patients (70). Compared to CT alone, the addition of PET resulted in a GTV decrease in 35% of patients and a GTV increase in 21%. Changes to GTV also influenced dose-volume histograms of neighboring organs. The total lung volume receiving at least 20 Gy changed in nearly 75% of patients, including 12 with dose reductions and 13 with dose increases. The total volume of heart receiving at least 36 Gy increased in 11 patients and decreased in 12 patients. This trial did not correlate pathologic tumor extent with radiographic tumor extent.
Although limited information is available about the use of 18F-FLT PET for esophageal radiation planning, a study by Han et al. suggests that 18F-FLT PET can be used to accurately define the GTV and may allow for decreased dose to normal organs (13). A particular strength of this study is that GTV delineation using 18F-FLT and 18F-FDG PET was validated against pathologic findings. Various normal tissue parameters, such as mean lung dose and mean heart dose, were improved using 18F-FLT PET. The authors noted that 18F-FLT PET should be used cautiously for esophageal radiation planning until these findings have been validated.
18F-FDG PET for treatment response assessment
Treatment for esophageal cancer, similar to that for other solid malignancies of the thorax, depends on the stage of the malignancy at the time of diagnosis. Although patients with stage IV disease do not benefit from surgical resection, studies have shown that most patients with stages 0-III esophageal cancer will benefit from surgical intervention. The timing of surgical intervention and schedule of associated chemotherapy and radiation will vary depending on the stage of disease. For example, those with stage 0 esophageal cancer or stage I esophageal cancer with a T1 lesion (no invasion of muscularis propia) often undergo surgery as a first-line treatment. However, a survival benefit and lower recurrence rates have been shown in those with more locally advanced disease who respond to concurrent chemotherapy and radiation therapy prior to surgical resection (72-75). Local failure rates after CRT can exceed 50% (3,4). Nonresponders are exposed to the toxic side effects of CRT therapy while appropriate surgical therapy is delayed (76-78). Therefore, it is important to be able to differentiate responders from nonresponders early during treatment so that future management can be optimal for each patient.
Invasive, minimally invasive, and noninvasive methods are available to assess treatment response. Endoscopic biopsy is limited in this effort, because it samples only the most superficial layers of mucosa; thus, biopsy may miss superficial in situ tumor as a result of sampling error, and cannot accurately determine the presence of residual nodal disease. In one large study of 118 patients with negative endoscopic biopsy after neoadjuvant therapy, only 37 patients (31.4%) demonstrated a complete pathologic response after esophagectomy (79). Similar findings were seen in a smaller study with 52 patients with negative endoscopic biopsies, 40 of whom (77%) had residual disease at resection (80). Given these limitations of biopsy, noninvasive methods have been used to help assess for residual disease after neoadjuvant therapy.
Multiple noninvasive or minimally invasive imaging procedures are used to evaluate treatment response after neoadjuvant therapy and include CT, EUS, and 18F-FDG PET. Although CT is an important tool in evaluating treatment response in many thoracic malignancies, its sensitivity (33-55%) and specificity (50-71%) in esophageal cancer after treatment are relatively poor (81). This is likely the result of the infiltrative growth pattern of esophageal cancers, which makes accurate measurements difficult, especially when tumors are small or extend into the stomach. This can limit the ability to assess response using the Response Evaluation Criteria in Solid Tumors criteria (82). Assessing treatment response can become even more challenging in the setting of radiation therapy, where inflammation, edema, and scarring can be difficult to differentiate from residual esophageal disease (Figure 1) (83). In addition, because many newer cancer therapies are cytostatic instead of cytocidal, good tumor response may occur without a major reduction in tumor size (84).
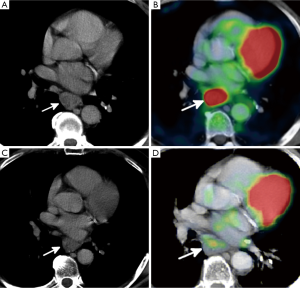
By providing information on the metabolic activity of tumor cells, 18F-FDG PET has become a powerful tool in assessment of treatment response in malignancies throughout the body. In one large meta-analysis assessing the performance of 18F-FDG PET after CRT in patients with esophageal cancer, sensitivities and specificities of PET ranged from 71% to 100% and 55% to 100%, respectively (81).
Given that the metabolic change on PET imaging is an important indicator of tumor response, new criteria were created to refine and validate quantitative approaches to monitoring PET tumor response (84). PET Response Criteria in Solid Tumors (PERCIST) is used to evaluate tumor response through quantitative assessment of changes in metabolic activity. The primary measurement of metabolic activity is the SUV, which is calculated by dividing metabolic activity by the injected dose and body weight. For PERCIST criteria, the SUV is corrected for lean body mass (SUL), because this metric is less susceptible to variations in the patient’s body weight (85). Metabolic response using PERCIST criteria is determined by assessing changes in the peak SUL, measured by drawing a spherical region of interest 1.2 cm in diameter (which correlates to a voxel size of 1 cc) over the area of greatest uptake in the tumor. It is important to note that the peak SUL measurement on repeat imaging may be placed on a different area within a tumor or in a different lesion altogether when assessing tumor response (57).
Based on changes in peak SUL, PERCIST defines four categories of treatment response. In complete metabolic response, the metabolic uptake in all lesions is less than the average SUL of liver and equal to normal surrounding tissue SUL (Figure 2). Partial metabolic response is defined as a >30% decrease in peak SUL (Figures 1 and 2). Progressive metabolic disease is defined as is >30% increase in the peak SUL. Stable metabolic disease occurs when PET findings do not meet any of these criteria. Recent studies have shown that PERCIST criteria are an independent predictor of survival in those with advanced esophageal cancer (82).
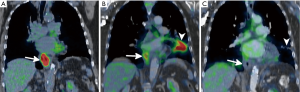
Although PET imaging is a strong noninvasive tool for assessment of treatment response in patients with esophageal cancer, it is by no means infallible. Increased 18F-FDG uptake can be seen in any process that leads to an increased metabolic rate, such as infection or inflammation (Figure 2). Because radiation therapy leads to direct esophageal injury, subsequent inflammation and ulceration will often demonstrate increased uptake on PET imaging and can be mistaken for residual tumor (Figure 1) (86,87). Because radiation esophagitis usually begins 2 weeks after initiation of therapy and is more common with higher radiation doses, evaluating treatment response within the first 2 weeks of treatment, before esophagitis has had time to develop, may be more accurate and less prone to false-positive findings (88).
The accuracy of PET imaging can be limited by respiratory motion artifact, which is greatest at the level of the diaphragm (Figure 3), and has been reported to occur in up to 84% of patients undergoing PET/CT (89,90) This can lead to quantitative inaccuracies in the calculation of SUVmax by up to 50%, which can lead to misalignment of 2-4.5 cm between the CT and PET (91). These artifacts can be counterbalanced by incorporating respiratory-gated CT imaging or volume-average CT imaging (88,89,91,92).
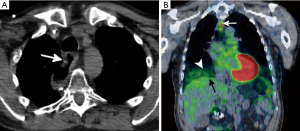
PET is also limited because metabolic response is determined by assessing only a small focal area with the most intense tracer uptake. However, this fails to evaluate the entire tumor; recent studies have emerged suggesting that spatial PET/CT features, including tumor volume, tumor shape, total glycolytic volume, and spatial patterns, are more informative than the traditional response measure of SUVmax in various tumors (41,93).
18F-FDG PET and response during treatment
Many patients with locally advanced esophageal cancer are referred to neoadjuvant therapy with either chemotherapy alone or CRT because of the potential OS benefit (2). However, it is well recognized that individual patient response to neoadjuvant therapy is variable, and it has been suggested that only 40-50% of patients will have a significant response to neoadjuvant therapy (94). Therefore, some patients may experience treatment-related toxicity without any significant benefit. Individualizing treatment to maximize treatment effect and minimize toxicity using noninvasive parameters would be ideal, and attempts have been made to correlate findings on 18F-FDG PET with clinical and pathologic outcomes.
Weber et al. published data in 2001 suggesting that locally advanced esophageal cancer patients receiving neoadjuvant chemotherapy could be stratified into metabolic responders and nonresponders based on 18F-FDG PET response and that this differentiation was directly correlated to disease control and survival (95). Patients underwent 18F-FDG PET imaging prior to treatment and 14 days after starting cisplatin-based chemotherapy. A dramatic difference in tracer uptake was seen in responders (54%) and nonresponders (15%), and the authors proposed an optimal cutoff value to be 35% reduction in initial 18F-FDG uptake. This cutoff was later prospectively validated by Ott et al. (43). Significantly more resected patients who were metabolic responders had either histopathologically complete or subtotal tumor regression than those who were not responders (53% and 5%, respectively). Metabolic response also predicted for longer time to disease progression (P=0.01) and longer overall survival (P=0.04).
Wieder et al. performed a similar assessment in 27 patients with esophageal SCC who underwent 18F-FDG PET imaging at baseline and 14 days after initiation of neoadjuvant therapy (48). Unlike the study by Weber et al., patients in this study received neoadjuvant radiation therapy in addition to chemotherapy, and the definition of metabolic response was slightly different (≥30% decrease in SUV uptake). Similar to the results of the study by Weber et al., early metabolic responders had improved survival (P=0.011) and significant histopathologic response was more common than in nonresponders (44% and 21%, respectively; P=0.0055). It is important to be aware that radiation therapy can induce inflammation that may cause false overestimation of true uptake in actual tumor during treatment (96). Preclinical data suggest that 18F-FLT PET may allow for better differentiation between inflammation and residual tumor during neoadjuvant therapy (97,98).
The phase II MUNICON (metabolic response evaluation for individualisation of neoadjuvant chemotherapy in esophageal and esophagogastric adenocarcinoma) trial evaluated the feasibility of using early 18F-FDG PET response to guide therapy (50). Patients classified as metabolic responders, defined by a ≥35% reduction in metabolic activity between pretreatment imaging and imaging performed 14 days after initiation of therapy, continued with neoadjuvant therapy prior to surgery. However, metabolic nonresponders proceeded directly to surgery in an attempt to spare these patients from chemotherapy-related toxicity. After a median follow-up of 2.3 years, median event-free survival (EFS) and OS in the nonresponders were 14.1 and 25.8 months, respectively. For metabolic responders, median event-free survival was 29.7 months and median OS was not reached, both of which were significantly higher than for metabolic nonresponders. Significant pathologic treatment effect (<10% residual tumor) was noted in 58% of responders, whereas no such effect was seen in the nonresponders. Metabolic responders who also achieved a major histologic response had significantly higher EFS [hazards ratio (HR) 3.03 (1.28-7.16); P=0.006] and OS [HR 4.55 (1.37-15.04); P=0.004] than the 21 metabolic responders who did not achieve a significant histologic response.
The MUNICON II trial was devised to determine whether metabolic nonresponders would have improved outcomes with the addition of salvage neoadjuvant CRT when <35% decrease in SUV uptake was identified on an 18F-FDG PET scan obtained at day 14 (55). Metabolic nonresponders switched from neoadjuvant chemotherapy to concurrent cisplatin and 5-fluorouracil and radiation therapy (32 Gy in 1.6-Gy fractions given twice a day). Metabolic responders continued to receive neoadjuvant chemotherapy for 3 months prior to surgical resection and did not receive radiation therapy. The primary endpoint was to increase the margin negative resection (R0) rate for metabolic nonresponders from 74% to 94%. Although R0 resections were obtained in 82% of the metabolic responders and 70% of the metabolic nonresponders, the primary endpoint was not met. One-year progression-free survival was higher among responders than nonresponders (74% and 57%, respectively; P=0.035). Median OS was lower in the nonresponders than in the whole group (18.3 and 38.3 months, respectively), and the median OS had not been reached for responders. The authors noted that although the radiation dose of 32 Gy was relatively low, a major histopathologic response was observed in 26% of the metabolic nonresponders who underwent CRT. As mentioned previously, none of the metabolic nonresponders in the first MUNICON trial had a major histopathologic response after chemotherapy alone, raising the possibility of cell killing as a factor in a subset of patients who received CRT. The authors concluded that salvage neoadjuvant CRT led to local remissions in a select group of patients; however, systemic disease continued to influence clinical outcomes and survival.
A recent Cancer and Leukemia Group B 80302 phase II trial (NCT00316862) is looking at whether giving induction chemotherapy of cisplatin and irinotecan followed by CRT therapy will have any influence on pathologic complete response rate at time of surgery. One of the secondary objectives is to evaluate for potential response or progression of disease during induction chemotherapy with 18F-FDG PET. Thus, patients will receive 18F-FDG PET imaging at baseline, 15-19 days after the start of induction chemotherapy, and within 7 days before beginning chemoradiotherapy. The results of this study are not yet available, but could potentially provide additional information about treatment response related to pathologic response.
18F-FDG PET and response after treatment
Although assessment of response during treatment is promising, the utility of posttreatment 18F-FDG PET imaging has been more thoroughly studied. Most of these studies are single-institution retrospective reports with fairly small patient numbers, but they collectively suggest that uptake on 18F-FDG PET after neoadjuvant treatment is associated with long-term outcomes and histopathologic outcomes (99-102) (Table 2). A recently published systematic review of 26 studies including 1,544 esophageal and GEJ cancer patients who received neoadjuvant therapy suggested that posttreatment 18F-FDG PET can effectively predict long-term outcomes (63). In fact, the pooled HR for complete metabolic response compared to no response was 0.51 for OS (95% CI, 0.4-0.64; P<0.0001) and 0.47 for DFS (95% CI, 0.38-0.57; P<0.0001).
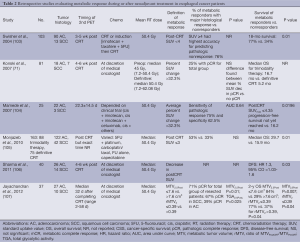
Full table
As previously discussed, investigators have questioned whether 18F-FDG PET metrics other than SUVmax are more useful for evaluating treatment response after neoadjuvant therapy. A recent study from the University of Maryland extracted comprehensive spatial-temporal 18F-FDG PET features from pre- and post-CRT PET scans in an attempt to predict pathologic tumor response in 20 esophageal patients (4). An area under receiver operating characteristic curve (AUC) value was used to quantify the ability of each feature to predict pathologic tumor response. In addition to SUVmax decline, two PET intensity features (mean SUV decline and skewness) and three PET texture features (inertia, correlation, and cluster prominence) were significant predictors of pathologic response. These novel PET features either had the same or higher AUCs than SUVmax. Recent data published by the same group using a support vector machine and logistic regression models suggest that these spatial-temporal 18F-FDG PET features may more accurately predict pathologic tumor response when combined with conventional PET/CT measures and clinical parameters (93).
When a patient undergoes esophagectomy, PET/CT is often used to monitor for recurrent or metastatic disease. Local disease recurrence most commonly occurs near the anastomotic site and may be a subtle finding on CT alone (Figure 3). In addition, PET can often detect distant metastatic disease, which can occur in 8-17% of patients on restaging imaging, before disease becomes apparent on standard anatomic imaging (83,88). Although disease recurrence after esophagectomy has a poor prognosis, therapy can be tailored toward palliation to improve patient symptoms and quality of life. Those who develop local or distal disease may be candidates for palliative therapy, including CRT, if adjuvant therapy has not been previously administered.
Conclusions
Advances in molecular imaging have led to dramatic improvements in care for esophageal cancer patients, ranging from diagnosis at an earlier and more manageable stage to altering treatment based on the degree of treatment response. Although 18F-FDG PET is the most widely used molecular imaging technique, its optimal utilization in esophageal cancer management is still unclear. The Cancer and Leukemia Group B 80302 trial may provide addition information about how to best incorporate 18F-FDG PET at various time points in the treatment of esophageal cancer.
Acknowledgements
The authors would like to thank Dr. Nancy Knight, Ph.D. for assisting with the preparation of this manuscript.
Disclosure: The authors declare no conflict of interest.
References
- American Cancer Society. Cancer Facts and Figures 2014. Available online: www.cancer.org/research/cancerfactsstatistics/cancerfactsfigures2014/
- Gebski V, Burmeister B, Smithers BM, et al. Survival benefits from neoadjuvant chemoradiotherapy or chemotherapy in oesophageal carcinoma: a meta-analysis. Lancet Oncol 2007;8:226-34. [PubMed]
- Stahl M, Stuschke M, Lehmann N, et al. Chemoradiation with and without surgery in patients with locally advanced squamous cell carcinoma of the esophagus. J Clin Oncol 2005;23:2310-7. [PubMed]
- Tan S, Kligerman S, Chen W, et al. Spatial-temporal [18F]FDG-PET features for predicting pathologic response of esophageal cancer to neoadjuvant chemoradiation therapy. Int J Radiat Oncol Biol Phys 2013;85:1375-82. [PubMed]
- Kobori O, Kirihara Y, Kosaka N, et al. Positron emission tomography of esophageal carcinoma using (11)C-choline and (18)F-fluorodeoxyglucose: a novel method of preoperative lymph node staging. Cancer 1999;86:1638-48. [PubMed]
- DeGrado TR, Baldwin SW, Wang S, et al. Synthesis and evaluation of 18F-labeled choline analogs as oncologic PET tracers. J Nucl Med 2001;42:1805-14. [PubMed]
- Hara T. 18F-fluorocholine: a new oncologic PET tracer. J Nucl Med 2001;42:1815-7. [PubMed]
- Tian M, Zhang H, Higuchi T, et al. Oncological diagnosis using (11)C-choline-positron emission tomography in comparison with 2-deoxy-2-[(18)F] fluoro-D-glucose-positron emission tomography. Mol Imaging Biol 2004;6:172-9. [PubMed]
- Kwee SA, DeGrado TR, Talbot JN, et al. Cancer imaging with fluorine-18-labeled choline derivatives. Semin Nucl Med 2007;37:420-8. [PubMed]
- Nobusawa A, Kim M, Kaira K, et al. Diagnostic usefulness of 18F-FAMT PET and L-type amino acid transporter 1 (LAT1) expression in oral squamous cell carcinoma. Eur J Nucl Med Mol Imaging 2013;40:1692-700. [PubMed]
- Sohda M, Kato H, Suzuki S, et al. 18F-FAMT-PET is useful for the diagnosis of lymph node metastasis in operable esophageal squamous cell carcinoma. Ann Surg Oncol 2010;17:3181-6. [PubMed]
- Yue J, Chen L, Cabrera AR, et al. Measuring tumor cell proliferation with 18F-FLT PET during radiotherapy of esophageal squamous cell carcinoma: a pilot clinical study. J Nucl Med 2010;51:528-34. [PubMed]
- Han D, Yu J, Zhong X, et al. Comparison of the diagnostic value of 3-deoxy-3-18F-fluorothymidine and 18F-fluorodeoxyglucose positron emission tomography/computed tomography in the assessment of regional lymph node in thoracic esophageal squamous cell carcinoma: a pilot study. Dis Esophagus 2012;25:416-26. [PubMed]
- Subramanian V, Ragunath K. Advanced endoscopic imaging: a review of commercially available technologies. Clin Gastroenterol Hepatol 2014;12:368-76.e1.
- Chandra S, Gorospe EC, Leggett CL, et al. Barrett’s esophagus in 2012: updates in pathogenesis, treatment, and surveillance. Curr Gastroenterol Rep 2013;15:322. [PubMed]
- Sturm MB, Joshi BP, Lu S, et al. Targeted imaging of esophageal neoplasia with a fluorescently labeled peptide: first-in-human results. Sci Transl Med 2013;5:184ra61.
- Templeton A, Hwang JH. Confocal microscopy in the esophagus and stomach. Clin Endosc 2013;46:445-9. [PubMed]
- von Holzen U, Enders GH. A surprise cell of origin for Barrett's esophagus. Cancer Biol Ther 2012;13:588-91. [PubMed]
- Franks I. Barrett esophagus: New insights into the stem cell organization of Barrett esophagus. Nat Rev Gastroenterol Hepatol 2012;9:125. [PubMed]
- Boja ES, Rodriguez H. Mass spectrometry-based targeted quantitative proteomics: achieving sensitive and reproducible detection of proteins. Proteomics 2012;12:1093-110. [PubMed]
- Picotti P, Aebersold R. Selected reaction monitoring-based proteomics: workflows, potential, pitfalls and future directions. Nat Methods 2012;9:555-66. [PubMed]
- Liebler DC, Zimmerman LJ. Targeted quantitation of proteins by mass spectrometry. Biochemistry 2013;52:3797-806. [PubMed]
- Liu YW, Aciego SM, Wanamaker AD Jr, et al. A high-throughput system for boron microsublimation and isotope analysis by total evaporation thermal ionization mass spectrometry. Rapid Commun Mass Spectrom 2013;27:1705-14. [PubMed]
- Su Y, Zhu Y, Fang Q. A multifunctional microfluidic droplet-array chip for analysis by electrospray ionization mass spectrometry. Lab Chip 2013;13:1876-82. [PubMed]
- van Vliet EP, Heijenbrok-Kal MH, Hunink MG, et al. Staging investigations for oesophageal cancer: a meta-analysis. Br J Cancer 2008;98:547-57. [PubMed]
- Lowe VJ, Booya F, Fletcher JG, et al. Comparison of positron emission tomography, computed tomography, and endoscopic ultrasound in the initial staging of patients with esophageal cancer. Mol Imaging Biol 2005;7:422-30. [PubMed]
- Rösch T. Endosonographic staging of esophageal cancer: a review of literature results. Gastrointest Endosc Clin N Am 1995;5:537-47. [PubMed]
- van Westreenen HL, Westerterp M, Bossuyt PM, et al. Systematic review of the staging performance of 18F-fluorodeoxyglucose positron emission tomography in esophageal cancer. J Clin Oncol 2004;22:3805-12. [PubMed]
- Rice TW. Clinical staging of esophageal carcinoma. CT, EUS, and PET. Chest Surg Clin N Am 2000;10:471-85. [PubMed]
- Flanagan FL, Dehdashti F, Siegel BA, et al. Staging of esophageal cancer with 18F-fluorodeoxyglucose positron emission tomography. AJR Am J Roentgenol 1997;168:417-24. [PubMed]
- Block MI, Patterson GA, Sundaresan RS, et al. Improvement in staging of esophageal cancer with the addition of positron emission tomography. Ann Thorac Surg 1997;64:770-6; discussion 776-7. [PubMed]
- Walker AJ, Spier BJ, Perlman SB, et al. Integrated PET/CT fusion imaging and endoscopic ultrasound in the pre-operative staging and evaluation of esophageal cancer. Mol Imaging Biol 2011;13:166-71. [PubMed]
- Weber WA, Ott K. Imaging of esophageal and gastric cancer. Semin Oncol 2004;31:530-41. [PubMed]
- You JJ, Wong RK, Darling G, et al. Clinical utility of 18F-fluorodeoxyglucose positron emission tomography/computed tomography in the staging of patients with potentially resectable esophageal cancer. J Thorac Oncol 2013;8:1563-9. [PubMed]
- Meyers BF, Downey RJ, Decker PA, et al. The utility of positron emir ssion tomography in staging of potentially operable carcinoma of the thoracic esophagus: results of the American College of Surgeons Oncology Group Z0060 trial. J Thorac Cardiovasc Surg 2007;133:738-45. [PubMed]
- Erasmus JJ, Munden RF. The role of integrated computed tomography positron-emission tomography in esophageal cancer: staging and assessment of therapeutic response. Semin Radiat Oncol 2007;17:29-37. [PubMed]
- Strauss LG. Fluorine-18 deoxyglucose and false-positive results: a major problem in the diagnostics of oncological patients. Eur J Nucl Med 1996;23:1409-15. [PubMed]
- Yamamoto Y, Nishiyama Y, Ishikawa S, et al. Correlation of 18F-FLT and 18F-FDG uptake on PET with Ki-67 immunohistochemistry in non-small cell lung cancer. Eur J Nucl Med Mol Imaging 2007;34:1610-6. [PubMed]
- van Westreenen HL, Cobben DC, Jager PL, et al. Comparison of 18F-FLT PET and 18F-FDG PET in esophageal cancer. J Nucl Med 2005;46:400-4. [PubMed]
- Fukunaga T, Okazumi S, Koide Y, et al. Evaluation of esophageal cancers using fluorine-18-fluorodeoxyglucose PET. J Nucl Med 1998;39:1002-7. [PubMed]
- Rizk N, Downey RJ, Akhurst T, et al. Preoperative 18[F]-fluorodeoxyglucose positron emission tomography standardized uptake values predict survival after esophageal adenocarcinoma resection. Ann Thorac Surg 2006;81:1076-81. [PubMed]
- Cerfolio RJ, Bryant AS. Maximum standardized uptake values on positron emission tomography of esophageal cancer predicts stage, tumor biology, and survival. Ann Thorac Surg 2006;82:391-4; discussion 394-5. [PubMed]
- Ott K, Weber WA, Lordick F, et al. Metabolic imaging predicts response, survival, and recurrence in adenocarcinomas of the esophagogastric junction. J Clin Oncol 2006;24:4692-8. [PubMed]
- Pan L, Gu P, Huang G, et al. Prognostic significance of SUV on PET/CT in patients with esophageal cancer: a systematic review and meta-analysis. Eur J Gastroenterol Hepatol 2009;21:1008-15. [PubMed]
- Westerterp M, Sloof GW, Hoekstra OS, et al. 18FDG uptake in oesophageal adenocarcinoma: linking biology and outcome. J Cancer Res Clin Oncol 2008;134:227-36. [PubMed]
- Omloo JM, Westerterp M, Sloof GW, et al. The value of positron emission tomography in the diagnosis and treatment of oesophageal cancer Ned Tijdschr Geneeskd 2008;152:365-70. [PubMed]
- Choi JY, Janq HJ, Shim YM, et al. 18F-FDG PET in patients with esophageal squamous cell carcinoma undergoing curative surgery: prognostic implications. J Nucl Med 2004;45:1843-50. [PubMed]
- Wieder HA, Brücher BL, Zimmermann F, et al. Time course of tumor metabolic activity during chemoradiotherapy of esophageal squamous cell carcinoma and response to treatment. J Clin Oncol 2004;22:900-8. [PubMed]
- Song SY, Kim JH, Ryu JS, et al. FDG-PET in the prediction of pathologic response after neoadjuvant chemoradiotherapy in locally advanced, resectable esophageal cancer. Int J Radiat Oncol Biol Phys 2005;63:1053-9. [PubMed]
- Lordick F, Ott K, Krause BJ, et al. PET to assess early metabolic response and to guide treatment of adenocarcinoma of the oesophagogastric junction: the MUNICON phase II trial. Lancet Oncol 2007;8:797-805. [PubMed]
- Higuchi I, Yasuda T, Yano M, et al. Lack of fludeoxyglucose F 18 uptake in posttreatment positron emission tomography as a significant predictor of survival after subsequent surgery in multimodality treatment for patients with locally advanced esophageal squamous cell carcinoma. J Thorac Cardiovasc Surg 2008;136:205-12. [PubMed]
- Javeri H, Xiao L, Rohren E, et al. The higher the decrease in the standardized uptake value of positron emission tomography after chemoradiation, the better the survival of patients with gastroesophageal adenocarcinoma. Cancer 2009;115:5184-92. [PubMed]
- Roedl JB, Halpern EF, Colen R, et al. Metabolic tumor width parameters as determined on PET/CT predict disease-free survival and treatment response in squamous cell carcinoma of the esophagus. Mol Imaging Biol 2009;11:54-60. [PubMed]
- Vallböhmer D, Hölscher AH, Dietlein M, et al. [18F]-Fluorodeoxyglucose-positron emission tomography for the assessment of histopathologic response and prognosis after completion of neoadjuvant chemoradiation in esophageal cancer. Ann Surg 2009;250:888-94. [PubMed]
- zum Büschenfelde CM, Herrmann K, Schuster T, et al. 18F-FDG PET-guided salvage neoadjuvant radiochemotherapy of adenocarcinoma of the esophagogastric junction: the MUNICON II trial. J Nucl Med 2011;52:1189-96. [PubMed]
- van Heijl M, Omloo JM, van Berge Henegouwen MI, et al. Fluorodeoxyglucose positron emission tomography for evaluating early response during neoadjuvant chemoradiotherapy in patients with potentially curable esophageal cancer. Ann Surg 2011;253:56-63. [PubMed]
- Wahl RL, Jacene H, Kasamon Y, et al. From RECIST to PERCIST: Evolving considerations for PET response criteria in solid tumors. J Nucl Med 2009;50:122S-50S. [PubMed]
- El Naqa I, Grigsby P, Apte A, et al. Exploring feature-based approaches in PET images for predicting cancer treatment outcomes. Pattern Recognit 2009;42:1162-71. [PubMed]
- Tixier F, Le Rest CC, Hatt M, et al. Intratumor heterogeneity characterized by textural features on baseline 18F-FDG PET images predicts response to concomitant radiochemotherapy in esophageal cancer. J Nucl Med 2011;52:369-78. [PubMed]
- Hyun SH, Choi JY, Shim YM, et al. Prognostic value of metabolic tumor volume measured by 18F-fluorodeoxyglucose positron emission tomography in patients with esophageal carcinoma. Ann Surg Oncol 2010;17:115-22. [PubMed]
- Chen SW, Hsieh TC, Ding HJ, et al. Pretreatment metabolic tumor volumes to predict the short-term outcome of unresectable locally advanced squamous cell carcinoma of the esophagus treated with definitive chemoradiotherapy. Nucl Med Commun 2014;35:291-7. [PubMed]
- Li YM, Lin Q, Zhao L, et al. Pre-treatment metabolic tumor volume and total lesion glycolysis are useful prognostic factors for esophageal squamous cell cancer patients. Asian Pac J Cancer Prev 2014;15:1369-73. [PubMed]
- Schollaert P, Crott R, Bertrand C, et al. A systematic review of the predictive value of (18)FDG-PET in esophageal and esophagogastric junction cancer after neoadjuvant chemoradiation on the survival outcome stratification. J Gastrointest Surg 2014;18:894-905. [PubMed]
- Thomas E, Crellin A, Harris K, et al. The role of endoscopic ultrasound (EUS) in planning radiotherapy target volumes for oesophageal cancer. Radiother Oncol 2004;73:149-51. [PubMed]
- Bradley J, Thorstad WL, Mutic S, et al. Impact of FDG-PET on radiation therapy volume delineation in non-small-cell lung cancer. Int J Radiat Oncol Biol Phys 2004;59:78-86. [PubMed]
- Giraud P, Grahek D, Montravers F, et al. CT and (18)F-deoxyglucose (FDG) image fusion for optimization of conformal radiotherapy of lung cancers. Int J Radiat Oncol Biol Phys 2001;49:1249-57. [PubMed]
- Lee YK, Cook G, Flower MA, et al. Addition of 18F-FDG-PET scans to radiotherapy planning of thoracic lymphoma. Radiother Oncol 2004;73:277-83. [PubMed]
- Geets X, Daisne JF, Gregoire V, et al. Role of 11-C-methionine positron emission tomography for the delineation of the tumor volume in pharyngo-laryngeal squamous cell carcinoma: comparison with FDG-PET and CT. Radiother Oncol 2004;71:267-73. [PubMed]
- Leong T, Everitt C, Yuen K, et al. A prospective study to evaluate the impact of FDG-PET on CT-based radiotherapy treatment planning for oesophageal cancer. Radiother Oncol 2006;78:254-61. [PubMed]
- Moureau-Zabotto L, Touboul E, Lerouge D, et al. Impact of computed tomography (CT) and 18F-deoxyglucose positron emission tomography (FDG-PET) image fusion for conformal radiotherapy in esophageal carcinoma Cancer Radiother 2005;9:152-60. [PubMed]
- Konski A, Doss M, Milestone B, et al. The integration of 18-fluoro-deoxy-glucose positron emission tomography and endoscopic ultrasound in the treatment-planning process for esophageal carcinoma. Int J Radiat Oncol Biol Phys 2005;61:1123-8. [PubMed]
- Fiorica F, di Bona D, Schepis F, et al. Preoperative chemoradiotherapy for oesophageal cancer: a systematic review and meta-analysis. Gut 2004;53:925-30. [PubMed]
- Jin HL, Zhu H, Ling TS, et al. Neoadjuvant chemoradiotherapy for resectable esophageal carcinoma: a meta-analysis. World J Gastroenterol 2009;15:5983-91. [PubMed]
- Urba SG, Orringer MB, Turrisi A, et al. Randomized trial of preoperative chemoradiation versus surgery alone in patients with locoregional esophageal carcinoma. J Clin Oncol 2001;19:305-13. [PubMed]
- Meredith KL, Weber JM, Turaga KK, et al. Pathologic response after neoadjuvant therapy is the major determinant of survival in patients with esophageal cancer. Ann Surg Oncol 2010;17:1159-67. [PubMed]
- Ancona E, Ruol A, Santi S, et al. Only pathologic complete response to neoadjuvant chemotherapy improves significantly the long term survival of patients with resectable esophageal squamous cell carcinoma: final report of a randomized, controlled trial of preoperative chemotherapy versus surgery alone. Cancer 2001;91:2165-74. [PubMed]
- Dittrick GW, Weber JM, Shridhar R, et al. Pathologic nonresponders after neoadjuvant chemoradiation for esophageal cancer demonstrate no survival benefit compared with patients treated with primary esophagectomy. Ann Surg Oncol 2012;19:1678-84. [PubMed]
- Hsu PK, Chien LI, Huang CS, et al. Comparison of survival among neoadjuvant chemoradiation responders, non-responders and patients receiving primary resection for locally advanced oesophageal squamous cell carcinoma: Does neoadjuvant chemoradiation benefit all? Interact Cardiovasc Thorac Surg 2013;17:460-6. [PubMed]
- Sarkaria IS, Rizk NP, Bains MS, et al. Post-treatment endoscopic biopsy is a poor-predictor of pathologic response in patients undergoing chemoradiation therapy for esophageal cancer. Ann Surg 2009;249:764-7. [PubMed]
- Yang Q, Cleary KR, Yao JC, et al. Significance of post-chemoradiation biopsy in predicting residual esophageal carcinoma in the surgical specimen. Dis Esophagus 2004;17:38-43. [PubMed]
- Westerterp M, van Westreenen HL, Reitsma JB, et al. Esophageal cancer: CT, endoscopic US, and FDG PET for assessment of response to neoadjuvant therapy--systematic review. Radiology 2005;236:841-51. [PubMed]
- Yanagawa M, Tatsumi M, Miyata H, et al. Evaluation of response to neoadjuvant chemotherapy for esophageal cancer: PET response criteria in solid tumors versus response evaluation criteria in solid tumors. Journal of nuclear medicine: official publication J Nucl Med 2012;53:872-80. [PubMed]
- Kim TJ, Kim HY, Lee KW, et al. Multimodality assessment of esophageal cancer: preoperative staging and monitoring of response to therapy. RadioGraphics 2009;29:403-21. [PubMed]
- Tirkes T, Hollar MA, Tann M, et al. Response criteria in oncologic imaging: review of traditional and new criteria. RadioGraphics 2013;33:1323-41. [PubMed]
- Sugawara Y, Zasadny KR, Neuhoff AW, et al. Reevaluation of the standardized uptake value for FDG: variations with body weight and methods for correction. Radiology 1999;213:521-5. [PubMed]
- Arslan N, Miller TR, Dehdashti F, et al. Evaluation of response to neoadjuvant therapy by quantitative 2-deoxy-2-[18F]fluoro-D-glucose with positron emission tomography in patients with esophageal cancer. Mol Imaging Biol 2002;4:301-10. [PubMed]
- Erasmus JJ, Munden RF, Truong MT, et al. Preoperative chemo-radiation-induced ulceration in patients with esophageal cancer: a confounding factor in tumor response assessment in integrated computed tomographic-positron emission tomographic imaging. J Thorac Oncol 2006;1:478-86. [PubMed]
- Bruzzi JF, Munden RF, Truong MT, et al. PET/CT of esophageal cancer: its role in clinical management. RadioGraphics 2007;27:1635-52. [PubMed]
- Chi PC, Mawlawi O, Luo D, et al. Effects of respiration-averaged computed tomography on positron emission tomography/computed tomography quantification and its potential impact on gross tumor volume delineation. Int J Radiat Oncol Biol Phys 2008;71:890-9. [PubMed]
- Osman MM, Cohade C, Nakamoto Y, et al. Respiratory motion artifacts on PET emission images obtained using CT attenuation correction on PET-CT. Eur J Nucl Med Mol Imaging 2003;30:603-6. [PubMed]
- Pan T, Mawlawi O, Nehmeh SA, et al. Attenuation correction of PET images with respiration-averaged CT images in PET/CT. J Nucl Med 2005;46:1481-7. [PubMed]
- Nehmeh SA, Erdi YE, Pan T, et al. Quantitation of respiratory motion during 4D-PET/CT acquisition. Med Phys 2004;31:1333-8. [PubMed]
- Zhang H, Tan S, Chen W, et al. Modeling pathologic response of esophageal cancer to chemoradiation therapy using spatial-temporal 18F-FDG PET features, clinical parameters, and demographics. Int J Radiat Oncol Biol Phys 2014;88:195-203. [PubMed]
- Krause BJ, Herrmann K, Wieder H, et al. 18F-FDG PET and 18F-FDG PET/CT for assessing response to therapy in esophageal cancer. J Nucl Med 2009;50:89S-96S. [PubMed]
- Weber WA, Ott K, Becker K, et al. Prediction of response to preoperative chemotherapy in adenocarcinomas of the esophagogastric junction by metabolic imaging. J Clin Oncol 2001;19:3058-65. [PubMed]
- Rosenberg R, Herrmann K, Gertler R, et al. The predictive value of metabolic response to preoperative radiochemotherapy in locally advanced rectal cancer measured by PET/CT. Int J Colorectal Dis 2009;24:191-200. [PubMed]
- Chao KS. Functional imaging for early prediction of response to chemoradiotherapy: 3'-deoxy-3'-18F-fluorothymidine positron emission tomography--a clinical application model of esophageal cancer. Semin Oncol 2006;33:S59-63. [PubMed]
- Apisarnthanarax S, Alauddin MM, Mourtada F, et al. Early detection of chemoradioresponse in esophageal carcinoma by 3'-deoxy-3'-3H-fluorothymidine using preclinical tumor models. Clin Cancer Res 2006;12:4590-7. [PubMed]
- Brücher BL, Weber W, Bauer M, et al. Neoadjuvant therapy of esophageal squamous cell carcinoma: response evaluation by positron emission tomography. Ann Surg 2001;233:300-9. [PubMed]
- Flamen P, Van Cutsem E, Lerut A, et al. Positron emission tomography for assessment of the response to induction radiochemotherapy in locally advanced oesophageal cancer. Ann Oncol 2002;13:361-8. [PubMed]
- Downey RJ, Akhurst T, Ilson D, et al. Whole body 18FDG-PET and the response of esophageal cancer to induction therapy: results of a prospective trial. J Clin Oncol 2003;21:428-32. [PubMed]
- Kim MK, Ryu JS, Kim SB, et al. Value of complete metabolic response by (18)F-fluorodeoxyglucose-positron emission tomography in oesophageal cancer for prediction of pathologic response and survival after preoperative chemoradiotherapy. Eur J Cancer 2007;43:1385-91. [PubMed]
- Swisher SG, Maish M, Erasmus JJ, et al. Utility of PET, CT, and EUS to identify pathologic responders in esophageal cancer. Ann Thorac Surg 2004;78:1152-60. [PubMed]
- Mamede M. FDG-PET/CT tumor segmentation-derived indices of metabolic activity to assess response to neoadjuvant therapy and progression-free survival in esophageal cancer: correlation with histopathology results. Am J Clin Oncol 2007;30:377-88. [PubMed]
- Monjazeb AM, Riedlinger G, Aklilu M, et al. Outcomes of patients with esophageal cancer staged with [18F]fluorodeoxyglucose positron emission tomography (FDG-PET): can postchemoradiotherapy FDG-PET predict the utility of resection? J Clin Oncol 2010;28:4714-21. [PubMed]
- Sharma NK, Silverman JS, Li T, et al. Decreased Posttreatment SUV on PET Scan Is Associated With Improved Local Control in Medically Inoperable Esophageal Cancer. Gastrointest Cancer Res 2011;4:84-9. [PubMed]
- Jayachandran P, Pai RK, Quon A, et al. Postchemoradiotherapy positron emission tomography predicts pathologic response and survival in patients with esophageal cancer. Int J Radiat Oncol Biol Phys 2012;84:471-7. [PubMed]