Non-operative therapies for colorectal liver metastases
Introduction
Metastatic disease to the liver is present at the time of diagnosis in 20% of patients with colorectal cancer and develops in an additional 40% over the course of their disease (1). In 30% of patients, the liver is the only site of metastatic disease. Liver resection offers the best chance of cure for patients with liver metastases with 5-year survivals of up to 50%. Unfortunately, only up to 25% of eligible patients undergo resection because of co-morbid conditions (1). First line chemotherapy with oxaliplatin or irinotecan and the addition of a biologic agent have increased median survival time to 18-21 months (2). However, after failing first line chemotherapy, the response rate to second line agents ranges from about 20-35% (3,4). For these reasons loco-regional or liver-directed therapies are significant for treatment of liver-predominant metastatic colorectal cancer (1). Liver-directed therapy may be administered with curative or palliative intent via open surgical, laparoscopic or image-guided percutaneous techniques. This review focuses only on non-operative techniques and their results.
Ablative therapies
Ablation of colorectal hepatic metastases is generally reserved for patients with disease confined to the liver. Ideally, patients should have three or fewer lesions in the liver with each lesion measuring ≤ three centimeters in diameter. Ablative therapies include radiofrequency ablation (RFA), microwave ablation, laser ablation and ultrasound ablation, all of which induce thermal damage to tissues. In contrast, cryoablation freezes tissue at temperatures ranging from –20 to –40 degrees centigrade that leads to cell death. Irreversible electroporation—an emerging technology that uses electrical energy to introduce pores in cellular membranes with resultant cellular destruction-is currently under investigation (5). In addition, percutaneous instillation of ethanol directly into a tumor is often performed in patients with hepatocellular carcinoma, though this technique is not yet frequently used in colorectal carcinoma.
Radiofrequency ablation (RFA)
RFA, the most extensively studied ablative technique for treatment of colorectal liver metastases, is similar in intent to surgical resection. Rapidly alternating electrical current produces ionic oscillations in bipolar water molecules, which then generate frictional heat (Figure 1). An electrode probe (Figure 2) is placed within the metastatic lesion, while grounding pads are placed on the lower extremities. Energy dispersion is greatest in the vicinity of the probe generating temperatures of >100 degrees centigrade. Cell death takes place after several minutes at 50 degrees centigrade and instantaneously at temperatures of >60 degrees centigrade. Current technology can produce lethal burns of >5 cm diameter with a single probe (Figure 3). Tissue destruction is dependent on heat conduction generated from the active elements of the probe; therefore, optimal lesions are <3 cm in diameter with incomplete destruction more likely with increasing lesion size. Impediments to effective RFA include lesion location adjacent to critical structures such as bowel or central bile ducts. Flowing blood in vessels >3 mm creates a heat sink effect, drawing heat away from the treated area. Tissue charring in the vicinity of the active elements creates an insulator, which can also limit heat conduction.
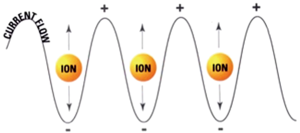
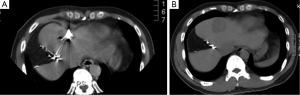
For small hepatocellular carcinomas, the results of RFA approach those of surgical resection with respect to recurrence, time to progression, and overall survival. For colorectal liver metastases, multiple retrospective case matched comparisons as well as meta-analyses are available, which compare surgical resection to RFA (6-8). An increased incidence of recurrence in the treated lesion (5-13%), decreased time to progression, and decreased median survival (1-year, –85%; 3-year, –36%, 5-year, –24%) are reported in patients undergoing RFA compared to surgical resection (7). In well-selected patients with 3 or fewer lesions less than 3 cm in diameter, 5-year survivals of up to 33% are reported (9). In patients who are not surgical candidates, RFA remains a valuable tool. It is increasingly used in conjunction with surgical resection to increase the number of surgical candidates. A study by Livraghi in 2003 demonstrated that RFA followed by incomplete ablation or recurrence in the ablative bed did not negatively affect subsequent surgical resection (10). The most important factor for local recurrence after RFA is tumor size, and larger lesions are at higher risk for local failure (Table 1).
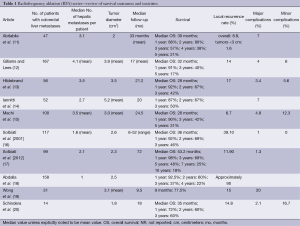
Full table
Microwave ablation
Microwave ablation relies on generation of electromagnetic radiation in the 900 to 2,450 MHz range from antennae placed within the treated lesion. Similar to RFA, ionic oscillations occur in response to oscillating electrical charge with generation of frictional heat (21). Microwave ablation offers a broader field of power density providing active heating up to 2 cm surrounding the antenna with less dependence on thermal conduction. This differs from RFA, where active generation of heat is only a few millimeters surrounding active elements and has a greater reliance on thermal conduction. Therefore, microwave ablation may lead to more uniform tissue heating with the ability to treat larger lesions using multiple antennae. There may also be less of a heat sink effect from flowing blood and less tissue charring. The elimination of the need for grounding pads, which carry an associated risk of skin burns, as well as shorter procedure times are additional advantages of microwave ablation (21).
Microwave ablation is being used increasingly as an open or laparoscopic procedure but also percutaneously under image guidance (21,22). While there are no randomized trials comparing it to RFA, certain advantages may exist. As with RFA, the incidence of recurrence in the treated lesion, as well as disease-free and overall survival favor surgical resection over microwave ablation. Recurrence rates following microwave ablation reported in observation studies and meta-analyses range from 5-13% with 1-, 3-, and 5-year survival rates of 73%, 30% and 16%, respectively (7). As with RFA, microwave ablation has been described in conjunction with surgical resection to achieve survival rates similar to resection alone (23) (Table 2).
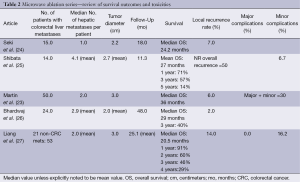
Full table
Cryoablation
Cryoablation is performed through the use of a probe within the lesion, where argon is infused, dropping tissue temperatures to –40 degrees centigrade and creating an ice ball of various sizes depending on probe configuration. Within the ice ball there are predictable thermal zones ranging from –40 degrees to 0 degrees centigrade. Tissue death occurs at –20 to –40 degrees centigrade. Advantages of cryoablation include ability to visualize the ice ball while using CT guidance and less procedure related pain. There are variable survival rates and high rates of complications (7). Disadvantages include the need for multiple probes and lack of a coagulative effect potentially leading to bleeding complications (28). The high rate of complications and fear of cryoshock has led to this technique falling out of favour as other safer and equally effective techniques have evolved (7). Currently cryoablation has been replaced by RFA and microwave ablation for ablative treatment within the liver (Table 3).
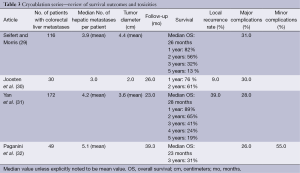
Full table
Embolization procedures
Locoregional therapies administered through the hepatic artery for the treatment of primary and metastatic hepatic cancer include bland particulate embolization, chemo-infusion, chemoembolization and radioembolization. The dual blood supply to the liver enhances the effectiveness of these techniques. Hepatic malignancies receive 80% of their blood supply from the hepatic artery. In contrast, the normal liver receives 80% of its blood supply from the portal vein with only 20% from the hepatic artery. Therefore, liver directed therapies through the hepatic artery preferentially target the neoplasm with relative sparing of normal liver parenchyma.
Bland embolization
Bland embolization utilizes inert particles of various sizes and composition to obstruct tumor microvasculature, leading to tumor infarction. While bland embolization is effective in the treatment of hepatocellular carcinoma and neuroendocrine tumors, it not frequently utilized for colorectal metastases.
Chemoinfusion
Chemoinfusion or delivery of chemotherapy directly into the hepatic artery results in high exposure of the liver to the chemotherapeutic agent. It can be delivered through catheters placed percutaneously into the hepatic artery or most commonly through pumps and catheters that are surgically implanted. Since placement of the hepatic artery infusion pump is most commonly placed via a surgical procedure, it will only be briefly discussed in this review. Floxuridine (FUDR) is the most commonly used drug and has a high first pass clearance by the liver, which enhances hepatic exposure and decreases systemic exposure. While it has high response rates (up to 85%), and demonstrates prolonged progression-free survival (up to 31 months), it is not clear that overall survival is improved over current first line chemotherapy. In addition it is plagued by catheter malfunction, arterial occlusion and hepatic toxicity including intra- and extra-hepatic bile duct damage (33,34). Its role after failure of first and second line chemotherapy as well as adjuvant therapy following liver resection also remains to be determined (35).
Chemoembolization
In contrast to simple infusion of chemotherapy through the hepatic artery, chemoembolization combines arterial obstruction with the delivery of chemotherapeutic agents. A commonly employed chemoembolization protocol combines the delivery of chemotherapeutic agents emulsified in ethiodized oil with particulate embolization. The ethiodized oil/chemotherapeutic mixture lodges distally within the hepatic arterioles and portal venules, trapping the agent in the tumor microvasculature. Bland embolization following the delivery of the ethiodized oil/chemotherapeutic mixture leads to stasis and increased contact time within the tumor, which increases local drug delivery while reducing systemic exposure (36). Recently, drug-eluting beads have been developed in which the chemotherapeutic agents such as doxorubicin or irinotecan are ionically bound to particles of various sizes. Following embolization there is a gradual prolonged release of the chemotherapeutic agent within the tumor with greatly reduced systemic release (37).
Hepatic artery chemoembolization is appropriate for patients with liver dominant metastatic disease, ECOG performance status of 0-2, and preserved liver function with serum bilirubin of less than 2 mg/dL. Ideally the portal circulation is preserved and tumor volume is less than 50%. Standard chemoembolization protocols include a variety of agents but often include mitomycin, doxorubicin and cisplatin emulsified in ethiodized oil (38). Treatment is performed in a lobar distribution starting with the more affected lobe and followed by treatment of the contralateral lobe in 15 to 30 days. Bilobar treatment is considered one cycle. Response is assessed with CT or MR imaging at 30-90 days and treatment cycles are repeated as necessary for disease progression. Most often, chemoembolization is considered after failure of first and second line chemotherapy. In a report by Albert et al., using the previously mentioned standard method of chemoembolization with mitomycin C, doxorubicin, and cisplatin mixed with ethiodized oil, the authors reported partial response, stable disease, and disease progression in 2%, 41%, and 57% of patients, respectively. Median time to liver progression was 5 months and overall disease progression was 3 months. Median survival following the first chemoembolization was 9 months (38). These results are similar to other series reporting median survivals of 8-14 months from the time of first chemoembolization (39-41). Complications of chemoembolization are common but most often minor, including the anticipated post embolization syndrome of fever, nausea, vomiting and abdominal pain, which are usually easily controlled and of limited duration. More serious complications including liver failure, renal failure, liver abscess, cholecystitis, myocardial infarction and pulmonary embolus have also been reported
Advances in drug delivery systems have led to further control of the release of chemotherapeutic agents within hepatic metastatic lesions, increasing contact time, while decreasing systemic exposure. These include hydrogels, microspheres, and polymer implants. The most extensively studied of these are non-biodegradable polyvinyl alcohol (PVA) microspheres (beads) (DC Bead, Biocompatables, West Conshohocken, PA, USA). The beads are modified with the addition of a sulfonic acid containing a moiety resulting in a charge that permits the interaction and binding with oppositely charged drugs, such as doxorubicin and irinotecan (37). Drug eluting beads loaded with irinotecan (DEBIRI) provide an alternative for chemoembolization of colorectal cancer metastatic to the liver. Beads of varying diameter (70-900 microns) are loaded with irinotecan, and based on disease distribution they are delivered in a lobar, segmental or superselective arterial distribution. For lobar administration, a single lobe is initially treated, while the second lobe is treated approximately 14-28 days later, with bilobar treatment considered a single treatment cycle. Cycles can be repeated if disease progression is noted.
In most reports, prior to DEBIRI chemoembolization, patients had undergone previous chemotherapy and failed at least one line of chemotherapy, with some having failed two or three regimens of chemotherapy. In general, patients tolerate DEBIRI well with the most common adverse event being the post-embolization syndrome. Abdominal pain, occasionally severe, is reported in 40-63% of patients. Hypertension is also frequently reported but is most often transient and related to pain. Aliberti reported results in 82 patients who failed initial lines of chemotherapy with response to DEBIRI of 78% at three months, progression free survival of 8 months and median survival of 25 months (42). These results are similar to those reported by Martin with response at 6 and 12 months of 66% and 75%, and progression free and overall survivals of 11 and 19 months, respectively (43). Other reports and meta-analyses report response to treatment in 18-78% of patients with median survival rates of 15-25 months (44). Patients failing only first line chemotherapy exhibit better overall response and survival compared to patients having failed multiple lines of chemotherapy. In a randomized trial comparing DEBIRI to 5-fluorouracil (5-FU)/leucovorin/irinotecan in patients failing first lines of chemotherapy, time to progression and overall survival favored DEBIRI at 7 and 22 versus 4 and 16 months, respectively (44). Toxicity including neutropenia, diarrhea, and mucositis were less frequent in the DEBIRI group (44). Both standard chemoembolization and DEBIRI have been reported to downstage up to 20% of patients to surgical resectability (45) (Table 4).
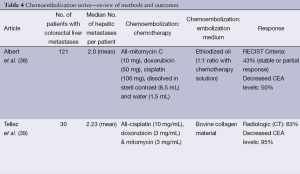
Full table
Radioembolization
Radioembolization incorporates Yttrium-90, a radioactive beta emitter (maximum energy 2.27 MeV and mean range of 2.5 mm in liver tissue) embedded in resin (SIRSpheres, SIRTEX) or glass microspheres (Theraspheres, MDS Nordion) for delivery of high dose radiation to the tumor with reduced radiation exposure to the remaining normal liver parenchyma. The radioembolization procedure consists of a femoral artery catheterization approach to the hepatic artery through which Yttrium-90 microspheres are delivered. Once in the tumor’s vascular network, these particles occlude the smallest capillaries leaving the majority of the microspheres within the tumor whereby they emit radiation therapy, which is known to be one of the most effective cancer therapies for solid tumors. Traditionally, the concern about radiation delivery to the liver has been the risk of radiation induced liver disease (RILD). Due to the hepatic artery-dominant blood supply for about 80% of liver tumors, the Yttrium-90 microspheres preferentially flow to the tumors. Pathological studies have confirmed the distribution of the microspheres (47). In patients treated with radioembolization, RILD consists of a constellation of icteric ascites, hepatomegaly, and mild transaminitis in relationship to the bilirubin, which is markedly elevated. Patients who have received chemotherapy prior to RE are at higher risk for RILD (48). This technique helps to overcome the radiosensitivity of the liver parenchyma.
As the half-life of Yttrium-90 is 64.8 hours, the particles are radioactive for a period of about 14 days but most of the radioactivity is delivered over five days. Although SIRSpheres and TheraSpheres are used interchangeably, SIRSpheres are FDA-approved for colorectal cancer metastases and Therasphers for hepatocellular carcinoma.
The radioembolization process is conducted in at least two parts. The first session, the mapping portion consists of a Technicium-99 macro-aggregated albumin SPECT scan during which particles mimicking the Yttrium-90 microspheres determine the percentage of lung shunting which may occur with the radioembolization procedure. If greater than 20% lung shunting occurs, then the patient is not eligible for radioembolization. Likewise, the Yttrium-90 dose may be modified based on the percentages of lung shunting. Also during this procedure, occlusion of the gastroduodenal artery or other collateral vessels may be performed to prevent retrograde flow of the microspheres, which can result in gastric and duodenal ulcers (15%). The radiation dose is calculated by the body surface area method or an empiric dose may be administered but is thought to carry higher rates of toxicity (49). The next procedure consists of the administration of the Yttrium-90 microspheres, which may be performed in a whole liver approach or sequential lobar treatments. The advantage of sequential treatments is to observe the effect of first radioembolization and to assure that sufficient contralateral liver reserve exists. Patients may be then monitored on an every 2-3 month basis after completion of therapy (Figure 4).
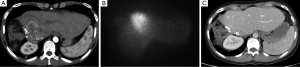
Appropriate patient selection for this procedure helps to ensure that the maximum benefit of this therapy will be provided. For colorectal cancer patients with liver metastases, it is preferable that the patient has liver-limited confirmed metastatic disease to achieve maximal benefit with RE. Small volume extrahepatic disease is permitted. Relative contraindications include a history of ascites or portal vein thrombosis, both conditions are more likely with hepatocellular carcinoma. Other requirements are a Zubrod performance status of 0-2; bilirubin <2 and ideally <1; creatinine <1.5× upper limit of normal, WBC >1.5×109/L; Plt >100×109/L; albumin >30 g/L.
The risks of this procedure include constitutional symptoms and abdominal pain, gastric/duodenal ulcer for which prophylactic proton pump inhibitors are initiated, and radiation induced liver injury. Results of radioemblization are shown in Table 5.
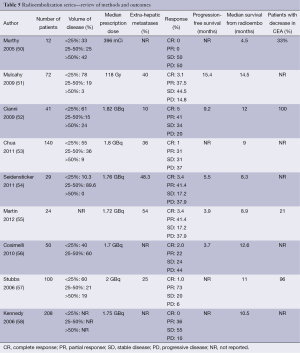
Full table
Several trials combining chemotherapy and radioembolization have demonstrated encouraging outcomes. van Hazel et al. (59) evaluated 5-FU and leucovorin with or without radioembolization in a phase II randomized trial in 21 patients. Radioembolization was administered on the 3rd or 4th day of the second cycle of chemotherapy. The response rate, median survival for 11 patients receiving combination therapy was significantly greater than those who received chemotherapy alone. Sharma et al. (60) evaluated combined radioembolization with modified FOLFOX4 in a phase I study of 20 patients. Grade 3 abdominal pain occurred in 25% of patients (with a 10% rate of radioembolization-related gastric ulcers), grade 3-4 neutropenia in 60% of patients, and one episode of transient grade 3 hepatotoxicity. Partial responses occurred in 90% of cases. Median progression-free survival was 9.3 months, and median time to progression in the liver was 12.3 months. Hendlisz et al. (61) conducted a phase III trial comparing continuous infusion 5-FU alone or with radioembolization for liver-limited metastatic CRC in 46 patients. Median time to tumor progression was 2.1 months for the 5-FU alone group versus 5.4 months in the combination group. Over half of patients (25/44) went on to receive further treatment after progression. Median overall survival was 7.3 months in the 5-FU arm and 10.3 months in the combination arm (P=0.80). Van Hazel also conducted a study of irinotecan concurrently with radioembolization and evaluated three dose levels of irinotecan, and did not reach a maximum tolerated dose, so a dose of 100 mg/m2 on days 1 and 8 of a 3-week cycle was recommended (62). Based on the available data, radioembolization is most often administered independently of chemotherapy to patients with liver-limited metastases to aid in prolonging survival and time to progression.
Stereotactic body radiotherapy (SBRT)
SBRT also known as stereotactic ablative body radiotherapy (SABR) denotes a precise delivery of high doses of radiation to an extracranial target in a small number (usually up to 6) of fractions (63). Standard fractionation of 1.8-2.0 Gray per day is effective because of the differential response of tumor and normal tissue to radiation therapy, with the repeated fractions allowing repair of normal tissues. In contrast, SBRT is thought to be more effective relative to standard fractionation radiation with apparent improvements in tumor cell kill. SBRT is complicated by the need to work with irregularly shaped targets and tumors that are influenced by organ and diaphragm motion. SBRT requires accurate immobilization, a method to manage the respiratory motion of the target, and image guidance to ensure proper alignment and delivery of the radiation dose. Historically SBRT has been used to treat smaller lesions (<6 cm in diameter) in the liver, and it may also be used for larger sized liver metastases as well (63).
SBRT requires careful radiation design with the first step of simulation to create a reproducible position of the patient, using a large rigid pillow conforming to the patient’s external contour with a reference coordinate system beneath and around the patient, which allows for a 3-dimensional localization of the patient. After creating of the immobilization device, a high resolution computed tomography (CT scan) with IV and oral contrast generates an image of the patient, target, and immobilization device. Acquisition of a 4-dimensional CT scan provides information about target and respiratory motion. CT images may be obtained with contrast and can be registered to diagnostic images, such as PET scans or MRIs. During simulation, the method of liver immobilization is determined with options based on institutional availability including controlled breath holds, shallow breathing, abdominal compression devices, beam gating timed to the respiratory cycle, or tumor tracking via implanted fiducial markers (64).
Manual delineation of the target and normal organs by the physician is followed by determination of a beam arrangement to meet specific dose constraints. This process may take several days to generate a highly conformal treatment plan via multiple iterations and discussion between the physician and physics team. Radiation beams may be delivered through a multi-field 3-dimensional conformal plan with a combination of coplanar and non-coplanar beams or intensity modulated radiation therapy (IMRT). To achieve an adequate SBRT plan, numerous beam angles must be employed (6 or more) such that each beam is sufficiently weak to spare radiation dose to normal organs, and at the convergence of the beams, the maximal radiation dose is delivered. Similarly, IMRT employs multiple angles, but within each angle, the shields within the linear accelerator (multileaf collimators) dynamically move to spare organs at risk. IMRT is less ideal for SBRT due to the interplay effect.
During each treatment, assessment of patient position is conducted via image guided radiation therapy, with fluoroscopy, megavoltage or kilovoltage X-rays or cone beam CT scans (CBCT) to assure accuracy of liver positioning. Alternatively, tracking may occur after the placement of fiducials. Although this is a relatively new modality for liver directed therapy, the non-invasive nature of therapy makes it particularly appealing.
Candidates for liver SBRT should have a sufficient performance status (ECOG 0-1) and liver function and no extrahepatic disease. The uninvolved liver volume should be 700 mL or greater (64). Based on the volume of disease, patient comorbidities, baseline liver function, and performance status, the multidisciplinary team can begin to make treatment recommendations for liver-directed therapy. For diffuse disease, the embolization procedures may be appropriate therapy. Although outcomes appear similar in chemo- and radio-embolization, it is important to recognize that the volume of disease in the available literature may be inconsistent. Likewise, most SBRT series allow for larger lesions than RFA despite having apparently similar outcomes (Table 6).
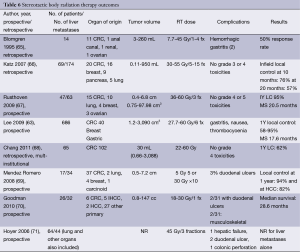
Full table
Summary
Decision making
We provide various cases to demonstrate the decision-making and representative images for each scenario.
Case discussion 1 (Figure 5)
A 67-year-old woman with a history of adenocarcinoma of the sigmoid colon status post sigmoidectomy presents with new onset fatigue and a rise in carcinoembryonic antigen (CEA). A CT of the chest, abdomen and pelvis, reveals new liver lesions in segments I and V, and a CT guided biopsy of the segment V reveals adenocarcinoma. As the patient had tolerated any of her chemotherapy with difficulty, medical oncology recommended liver-directed therapy. Interventional radiology reviewed her films but did not this that was an optimal situation for RFA due to possible heat sink of the central lesion and possible gastric injury for the segment V lesion. Given her low volume of disease, chemo- or radio-embolization was not warranted. Therefore, SBRT was proposed and delivered to the patient.
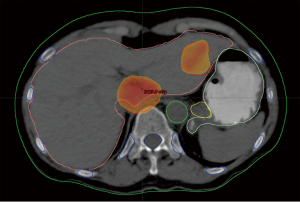
Case discussion 2 (Figure 6)
A 45-year-old woman with a diagnosis of diffuse liver metastases due to colon cancer requires a chemotherapy holiday due to neuropathy from therapy. She has been treated with FOLFOX and FOLFIRI. Given the diffuse nature of her liver metastases and clinical situation, Y90 radio-embolizaiton was recommended. Chemoembolization was considered to be an option but available results have shown better outcomes for smaller volume disease.
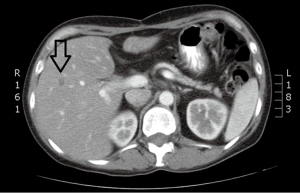
Case discussion 3 (Figure 7)
A 54-year-old man with a remote history of rectal cancer is found to have a new solitary liver metastasis measuring 2 cm. The patient has agreed to receive chemotherapy. RFA was recommended by the multidisciplinary liver tumor board given its long track record and good success in tumors <3 cm.
Conclusions
While there have been many exciting developments in liver directed therapy, most of our understanding of treatment outcomes is derived from observational studies. This limits our ability to critically compare techniques and to incorporate them into the treatment armamentarium of metastatic colorectal cancer. Randomized control trials of liver-directed therapy are necessary to elucidate the optimal management for patients with hepatic metastases. In the interim, multidisciplinary discussion at tumor boards is necessary to carefully decide on the optimal therapy for each patient.
Acknowledgements
Disclosure: The authors declare no conflict of interest.
References
- Wang DS, Louie JD, Sze DY. Intra-Arterial Therapies for Metastatic Colorectal Cancer. Semin Intervent Radiol 2013;30:12-20. [PubMed]
- Köhne CH, Lenz HJ. Chemotherapy with targeted agents for the treatment of metastatic colorectal cancer. Oncologist 2009;14:478-88. [PubMed]
- Tabernero J, Van Cutsem E, Lakomý R, et al. Aflibercept versus placebo in combination with fluorouracil, leucovorin and irinotecan in the treatment of previously treated metastatic colorectal cancer: prespecified subgroup analyses from the VELOUR trial. Eur J Cancer 2014;50:320-31. [PubMed]
- Peeters M, Price TJ, Cervantes A, et al. Final results from a randomized phase 3 study of FOLFIRI {+/-} panitumumab for second-line treatment of metastatic colorectal cancer. Ann Oncol 2014;25:107-16. [PubMed]
- Narayanan G. Irreversible electroporation for treatment of liver cancer. Gastroenterol Hepatol (N Y) 2011;7:313-6. [PubMed]
- Weng M, Zhang Y, Zhou D, et al. Radiofrequency ablation versus resection for colorectal cancer liver metastases: a meta-analysis. PLoS One 2012;7:e45493. [PubMed]
- Pathak S, Jones R, Tang JM, et al. Ablative therapies for colorectal liver metastases: a systematic review. Colorectal Dis 2011;13:e252-65. [PubMed]
- Mulier S, Ruers T, Jamart J, et al. Radiofrequency ablation versus resection for resectable colorectal liver metastases: time for a randomized trial? An update. Dig Surg 2008;25:445-60. [PubMed]
- Gillams AR, Lees WR. Five-year survival in 309 patients with colorectal liver metastases treated with radiofrequency ablation. Eur Radiol 2009;19:1206-13. [PubMed]
- Livraghi T, Solbiati L, Meloni F, et al. Percutaneous radiofrequency ablation of liver metastases in potential candidates for resection: the “test-of-time approach”. Cancer 2003;97:3027-35. [PubMed]
- Abitabile P, Hartl U, Lange J, et al. Radiofrequency ablation permits an effective treatment for colorectal liver metastasis. Eur J Surg Oncol 2007;33:67-71. [PubMed]
- Gillams AR, Lees WR. Radio-frequency ablation of colorectal liver metastases in 167 patients. Eur Radiol 2004;14:2261-7. [PubMed]
- Hildebrand P, Kleemann M, Roblick UJ, et al. Radiofrequency-ablation of unresectable primary and secondary liver tumors: results in 88 patients. Langenbecks Arch Surg 2006;391:118-23. [PubMed]
- Iannitti DA, Dupuy DE, Mayo-Smith WW, et al. Hepatic radiofrequency ablation. Arch Surg 2002;137:422-6; discussion 427. [PubMed]
- Machi J, Oishi AJ, Sumida K, et al. Long-term outcome of radiofrequency ablation for unresectable liver metastases from colorectal cancer: evaluation of prognostic factors and effectiveness in first- and second-line management. Cancer J 2006;12:318-26. [PubMed]
- Solbiati L, Livraghi T, Goldberg SN, et al. Percutaneous radio-frequency ablation of hepatic metastases from colorectal cancer: long-term results in 117 patients. Radiology 2001;221:159-66. [PubMed]
- Solbiati L, Ahmed M, Cova L, et al. Small liver colorectal metastases treated with percutaneous radiofrequency ablation: local response rate and long-term survival with up to 10-year follow-up. Radiology 2012;265:958-68. [PubMed]
- Abdalla EK, Vauthey JN, Ellis LM, et al. Recurrence and outcomes following hepatic resection, radiofrequency ablation, and combined resection/ablation for colorectal liver metastases. Ann Surg 2004;239:818-25; discussion 825-7. [PubMed]
- Wong SL, Edwards MJ, Chao C, et al. Radiofrequency ablation for unresectable hepatic tumors. Am J Surg 2001;182:552-7. [PubMed]
- Schindera ST, Nelson RC, DeLong DM, et al. Intrahepatic tumor recurrence after partial hepatectomy: value of percutaneous radiofrequency ablation. J Vasc Interv Radiol 2006;17:1631-7. [PubMed]
- Simon CJ, Dupuy DE, Mayo-Smith WW. Microwave ablation: principles and applications. Radiographics 2005;25 Suppl 1:S69-83. [PubMed]
- Iannitti DA, Martin RC, Simon CJ, et al. Hepatic tumor ablation with clustered microwave antennae: the US Phase II trial. HPB (Oxford) 2007;9:120-4. [PubMed]
- Martin RC, Scoggins CR, McMasters KM. Safety and efficacy of microwave ablation of hepatic tumors: a prospective review of a 5-year experience. Ann Surg Oncol 2010;17:171-8. [PubMed]
- Seki T, Wakabayashi M, Nakagawa T, et al. Percutaneous microwave coagulation therapy for solitary metastatic liver tumors from colorectal cancer: a pilot clinical study. Am J Gastroenterol 1999;94:322-7. [PubMed]
- Shibata T, Niinobu T, Ogata N, et al. Microwave coagulation therapy for multiple hepatic metastases from colorectal carcinoma. Cancer 2000;89:276-84. [PubMed]
- Bhardwaj N, Strickland AD, Ahmad F, et al. Microwave ablation for unresectable hepatic tumours: clinical results using a novel microwave probe and generator. Eur J Surg Oncol 2010;36:264-8. [PubMed]
- Liang P, Dong B, Yu X, et al. Prognostic factors for percutaneous microwave coagulation therapy of hepatic metastases. AJR Am J Roentgenol 2003;181:1319-25. [PubMed]
- Bageacu S, Kaczmarek D, Lacroix M, et al. Cryosurgery for resectable and unresectable hepatic metastases from colorectal cancer. Eur J Surg Oncol 2007;33:590-6. [PubMed]
- Seifert JK, Morris DL. Prognostic factors after cryotherapy for hepatic metastases from colorectal cancer. Ann Surg 1998;228:201-8. [PubMed]
- Joosten J, Jager G, Oyen W, et al. Cryosurgery and radiofrequency ablation for unresectable colorectal liver metastases. Eur J Surg Oncol 2005;31:1152-9. [PubMed]
- Yan DB, Clingan P, Morris DL. Hepatic cryotherapy and regional chemotherapy with or without resection for liver metastases from colorectal carcinoma: how many are too many? Cancer 2003;98:320-30. [PubMed]
- Paganini AM, Rotundo A, Barchetti L, et al. Cryosurgical ablation of hepatic colorectal metastases. Surg Oncol 2007;16 Suppl 1:S137-40. [PubMed]
- Bartlett DL, Berlin J, Lauwers GY, et al. Chemotherapy and regional therapy of hepatic colorectal metastases: expert consensus statement. Ann Surg Oncol 2006;13:1284-92. [PubMed]
- Barber FD, Mavligit G, Kurzrock R. Hepatic arterial infusion chemotherapy for metastatic colorectal cancer: a concise overview. Cancer Treat Rev 2004;30:425-36. [PubMed]
- Kemeny NE, Gonen M. Hepatic arterial infusion after liver resection. N Engl J Med 2005;352:734-5. [PubMed]
- Osuga K, Maeda N, Higashihara H, et al. Current status of embolic agents for liver tumor embolization. Int J Clin Oncol 2012;17:306-15. [PubMed]
- Liapi E, Lee KH, Georgiades CC, et al. Drug-eluting particles for interventional pharmacology. Tech Vasc Interv Radiol 2007;10:261-9. [PubMed]
- Albert M, Kiefer MV, Sun W, et al. Chemoembolization of colorectal liver metastases with cisplatin, doxorubicin, mitomycin C, ethiodol, and polyvinyl alcohol. Cancer 2011;117:343-52. [PubMed]
- Tellez C, Benson AB 3rd, Lyster MT, et al. Phase II trial of chemoembolization for the treatment of metastatic colorectal carcinoma to the liver and review of the literature. Cancer 1998;82:1250-9. [PubMed]
- Sanz-Altamira PM, Spence LD, Huberman MS, et al. Selective chemoembolization in the management of hepatic metastases in refractory colorectal carcinoma: a phase II trial. Dis Colon Rectum 1997;40:770-5. [PubMed]
- Vogl TJ, Gruber T, Balzer JO, et al. Repeated transarterial chemoembolization in the treatment of liver metastases of colorectal cancer: prospective study. Radiology 2009;250:281-9. [PubMed]
- Aliberti C, Fiorentini G, Muzzio PC, et al. Trans-arterial chemoembolization of metastatic colorectal carcinoma to the liver adopting DC Bead®, drug-eluting bead loaded with irinotecan: results of a phase II clinical study. Anticancer Res 2011;31:4581-7. [PubMed]
- Martin RC 2nd, Scoggins CR, Tomalty D, et al. Irinotecan drug-eluting beads in the treatment of chemo-naive unresectable colorectal liver metastasis with concomitant systemic fluorouracil and oxaliplatin: results of pharmacokinetics and phase I trial. J Gastrointest Surg 2012;16:1531-8. [PubMed]
- Fiorentini G, Aliberti C, Tilli M, et al. Intra-arterial Infusion of Irinotecan-loaded Drug-eluting Beads (DEBIRI®) versus Intravenous Therapy (FOLFIRI) for Hepatic Metastases from Colorectal Cancer: Final Results of a Phase III Study. Anticancer Res 2012;32:1387-95. [PubMed]
- Richardson AJ, Laurence JM, Lam VW. Transarterial chemoembolization with irinotecan beads in the treatment of colorectal liver metastases: systematic review. J Vasc Interv Radiol 2013;24:1209-17. [PubMed]
- Eichler K, Zangos S, Mack MG, et al. First human study in treatment of unresectable liver metastases from colorectal cancer with irinotecan-loaded beads (DEBIRI). Int J Oncol 2012;41:1213-20. [PubMed]
- Kennedy AS, Nutting C, Coldwell D, et al. Pathologic response and microdosimetry of (90)Y microspheres in man: review of four explanted whole livers. Int J Radiat Oncol Biol Phys 2004;60:1552-63. [PubMed]
- Sangro B, Gil-Alzugaray B, Rodriguez J, et al. Liver disease induced by radioembolization of liver tumors: description and possible risk factors. Cancer 2008;112:1538-46. [PubMed]
- Kennedy AS, McNeillie P, Dezarn WA, et al. Treatment parameters and outcome in 680 treatments of internal radiation with resin 90Y-microspheres for unresectable hepatic tumors. Int J Radiat Oncol Biol Phys 2009;74:1494-500. [PubMed]
- Murthy R, Xiong H, Nunez R, et al. Yttrium 90 resin microspheres for the treatment of unresectable colorectal hepatic metastases after failure of multiple chemotherapy regimens: preliminary results. J Vasc Interv Radiol 2005;16:937-45. [PubMed]
- Mulcahy MF, Lewandowski RJ, Ibrahim SM, et al. Radioembolization of colorectal hepatic metastases using yttrium-90 microspheres. Cancer 2009;115:1849-58. [PubMed]
- Cianni R, Urigo C, Notarianni E, et al. Selective internal radiation therapy with SIR-spheres for the treatment of unresectable colorectal hepatic metastases. Cardiovasc Intervent Radiol 2009;32:1179-86. [PubMed]
- Chua TC, Bester L, Saxena A, et al. Radioembolization and systemic chemotherapy improves response and survival for unresectable colorectal liver metastases. J Cancer Res Clin Oncol 2011;137:865-73. [PubMed]
- Seidensticker R, Denecke T, Kraus P, et al. Matched-pair comparison of radioembolization plus best supportive care versus best supportive care alone for chemotherapy refractory liver-dominant colorectal metastases. Cardiovasc Intervent Radiol 2012;35:1066-73. [PubMed]
- Martin LK, Cucci A, Wei L, et al. Yttrium-90 radioembolization as salvage therapy for colorectal cancer with liver metastases. Clin Colorectal Cancer 2012;11:195-9. [PubMed]
- Cosimelli M, Golfieri R, Cagol PP, et al. Multi-centre phase II clinical trial of yttrium-90 resin microspheres alone in unresectable, chemotherapy refractory colorectal liver metastases. Br J Cancer 2010;103:324-31. [PubMed]
- Stubbs RS, O’Brien I, Correia MM. Selective internal radiation therapy with 90Y microspheres for colorectal liver metastases: single-centre experience with 100 patients. ANZ J Surg 2006;76:696-703. [PubMed]
- Kennedy AS, Coldwell D, Nutting C, et al. Resin 90Y-microsphere brachytherapy for unresectable colorectal liver metastases: modern USA experience. Int J Radiat Oncol Biol Phys 2006;65:412-25. [PubMed]
- van Hazel G, Blackwell A, Anderson J, et al. Randomised phase 2 trial of SIR-Spheres plus fluorouracil/leucovorin chemotherapy versus fluorouracil/leucovorin chemotherapy alone in advanced colorectal cancer. J Surg Oncol 2004;88:78-85. [PubMed]
- Sharma RA, Van Hazel GA, Morgan B, et al. Radioembolization of liver metastases from colorectal cancer using yttrium-90 microspheres with concomitant systemic oxaliplatin, fluorouracil, and leucovorin chemotherapy. J Clin Oncol 2007;25:1099-106. [PubMed]
- Hendlisz A, Van den Eynde M, Peeters M, et al. Phase III trial comparing protracted intravenous fluorouracil infusion alone or with yttrium-90 resin microspheres radioembolization for liver-limited metastatic colorectal cancer refractory to standard chemotherapy. J Clin Oncol 2010;28:3687-94. [PubMed]
- van Hazel GA, Pavlakis N, Goldstein D, et al. Treatment of fluorouracil-refractory patients with liver metastases from colorectal cancer by using yttrium-90 resin microspheres plus concomitant systemic irinotecan chemotherapy. J Clin Oncol 2009;27:4089-95. [PubMed]
- Lee MT, Kim JJ, Dinniwell R, et al. Phase I study of individualized stereotactic body radiotherapy of liver metastases. J Clin Oncol 2009;27:1585-91. [PubMed]
- Høyer M, Swaminath A, Bydder S, et al. Radiotherapy for liver metastases: a review of evidence. Int J Radiat Oncol Biol Phys 2012;82:1047-57. [PubMed]
- Blomgren H, Lax I, Näslund I, et al. Stereotactic high dose fraction radiation therapy of extracranial tumors using an accelerator. Clinical experience of the first thirty-one patients. Acta Oncol 1995;34:861-70. [PubMed]
- Katz AW, Carey-Sampson M, Muhs AG, et al. Hypofractionated stereotactic body radiation therapy (SBRT) for limited hepatic metastases. Int J Radiat Oncol Biol Phys 2007;67:793-8. [PubMed]
- Rusthoven KE, Kavanagh BD, Cardenes H, et al. Multi-institutional phase I/II trial of stereotactic body radiation therapy for liver metastases. J Clin Oncol 2009;27:1572-8. [PubMed]
- Chang DT, Swaminath A, Kozak M, et al. Stereotactic body radiotherapy for colorectal liver metastases: a pooled analysis. Cancer 2011;117:4060-9. [PubMed]
- Méndez Romero A, Wunderink W, van Os RM, et al. Quality of life after stereotactic body radiation therapy for primary and metastatic liver tumors. Int J Radiat Oncol Biol Phys 2008;70:1447-52. [PubMed]
- Goodman KA, Wiegner EA, Maturen KE, et al. Dose-escalation study of single-fraction stereotactic body radiotherapy for liver malignancies. Int J Radiat Oncol Biol Phys 2010;78:486-93. [PubMed]
- Hoyer M, Roed H, Traberg Hansen A, et al. Phase II study on stereotactic body radiotherapy of colorectal metastases. Acta Oncol 2006;45:823-30. [PubMed]