Evaluation of normal tissue exposure in patients receiving radiotherapy for pancreatic cancer based on RTOG 0848
Introduction
Pancreatic cancer accounts for over 30,000 deaths annually in the United States (1). Surgical resection offers the best chance of long term survival. Both local and systemic recurrences are common after a pancreaticoduodenectomy. Adjuvant chemoradiotherapy (CRT) is utilized in many cases to improve locoregional control. But, toxicities associated with radiation may be significant given the location of the pancreas. The ongoing RTOG 0848 protocol seeks to further prospectively investigate the role of CRT using either IMRT or 3D conformal photon radiotherapy (3DCRT) in pancreatic cancer.
Conventional radiation therapy (3DCRT therapy) utilizes X-ray beams which enter and exit the body creating both entrance and exit dose. As a result, non-targeted organs and surrounding the pancreatic target are also exposed to radiation. Improvements in radiation delivery techniques have found methods to improve beam conformity around treatment targets. Intensity-modulated radiation therapy (IMRT) is one such method that utilizes multiple beam angles at varying intensities to escalate dose at the target while sparing surrounding normal tissue from high dose regions. One report has suggested IMRT can reduce high grade gastrointestinal (GI) toxicity in the setting of pancreatic cancer (2). Proton radiotherapy is another form of radiation treatment which utilizes charged particle beams. Proton beams deposit most of its energy at a discreet depth within tissue called the “Bragg Peak.” The Bragg Peak is predictable and can be created to match the exact depth and thickness of the tumor target. The entirety of the beam’s energy is deposited so there is no subsequent exit dose. Some previous dosimetric studies have shown a potential role for proton radiotherapy in the post-operative setting (3). The purpose of this study was to quantify the dosimetric changes seen in using protons or photons with consistent planning parameters in patients receiving CRT for pancreatic cancer.
Patients and methods
Patient selection
We retrospectively evaluated ten patient cases from our institution with pancreatic head adenocarcinoma. See Table 1 for a summary of patient characteristics. All patients were treated between 2010 and 2013.
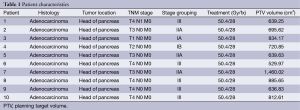
Full table
Simulation and treatment planning
Patients were simulated in the supine position using intravenous and oral contrast-enhanced CT imaging (GE Lightspeed VCT scanner, Little Chalfont, UK) with 2.5 mm slice thickness. All patients were scanned from above the diaphragm to 3 to 4 cm below the iliac crest. All treatment plans were created with Odyssey 4.8 planning system (Optivus Proton Therapy, Inc., San Bernardino, USA). All planning volume expansions were created per RTOG 0848 treatment guidelines. The gross tumor volume (GTV) was defined as the pre-operative tumor bed, for the purposes of this study, the gross tumor lesions. The clinical target volume (CTV) was defined as the GTV plus a 1 cm manual expansion in all directions. Also included in the CTV were the celiac axis, superior mesenteric artery (SMA), the portal vein (PV) plus a 1 cm expansion in all directions, and an asymmetric aortic expansion. The pancreaticojejunostomy (PJ) or pancreaticogastrostomy (PG) was included in the CTV, if readily visible. Setup uncertainty from respiratory motion and diaphragm movement was accounted for with close attention to target expansions. The planning target volume (PTV) was generated by expanding the CTV by 1.5 and 1.2 cm for the 3DCRT and IMRT plans, respectively. All 3DCRT plans are given a 1 cm margin beyond the PTV to the block edge to account for beam penumbra. The lateral penumbra and distal margin of proton plans generated were between 1-1.5 by the treatment planning system based on the beam energy selected.
A dose of 50.4 Gy given in 28 fractions was delivered to the PTV. All plans were optimized to allow 95% isodose coverage of at least 90% of the PTV. The proton plan beam arrangements consisted of 2 to 4 beams at oblique angles. The median proton beam energy was 250 MeV with some minor deviations depending on the depth of the target. The depth of the proximal and distal edge helped determine the beam energy selected. Our institution uses a passive scattering beam system which requires a patient portal-specific collimating aperture to shape the dose to the target field laterally. A range compensator was used to conform the dose to the distal aspect of the target volume. A spread-out Bragg peak to cover the target in the beam direction is achieved with a modulator wheel. All IMRT plans consisted of 6 to 9 coplanar, non-parallel opposed 6 MV photon beams delivered with a multi-leaf collimator using a step-and-shoot technique. Each 3DCRT plans each consisted of a 4-field box (AP, PA, RL, LL) using 15-24 MV photon beams delivered with either a multi-leaf collimator or custom-cut block.
Normal tissue dose-volume constraints per RTOG 0848 were strictly adhered to. For total kidney D50% <18 Gy and mean dose <18 Gy. If there is only one kidney present then the D15% ≤18 Gy. Mean liver dose was ≤25 Gy. The maximum dose to stomach and bowel was kept ≤54 Gy and D15% <45 Gy. The max dose to a point 0.03 cm3 on the spinal cord was kept ≤45 Gy.
Plan evaluation and analysis
In order to compare the plans with the different modalities and beam arrangements, dose-volume histograms (DVH) were calculated and analyzed. The organs at risk (OAR) being evaluated in this study are the kidneys, liver, small bowel, and spinal cord. Analysis was performed for the volume of kidney receiving 15 Gy (V15), 20 Gy (V20), and mean kidney doses were collected for the left, right and bilateral kidneys. The small bowel V15 and V50, the dose delivered to 1/3 of the liver (D1/3), mean liver dose, and the maximum spinal cord dose were also analyzed. Each plan was created to ensure that 90% of the PTV received at least 95% of the prescription dose and at least 99% of the CTV received 95% of the prescribed dose.
Conformity indices were also obtained and analyzed for plans between the three treatment modalities. The homogeneity index (HI) was defined as the difference between the maximum and minimum dose to the target volume (D1% and D99%, respectively) divided by the prescription dose (4,5). Uniformity index (UI) was also used; it was defined as the ratio of D5% to D95% (6,7). Both HI and UI were utilized to assess overall plan uniformity per previously established methodology (8). The conformity index was defined, per RTOG guidelines, as the volume of the 95% isodose curve divided by the PTV volume. To determine statistical significance, ANOVA and two-tailed paired t-tests were performed with P values <0.05 considered to be statistically significant.
Results
A total of ten patient scans were utilized for this study. Three treatment plans were created on each scan: proton, IMRT, 3DCRT. Table 2 presents dose-volume parameters obtained from these plans. Dose distributions for liver, kidney, and small bowel from two of our study patients are presented in Figure 1. The CTV was encompassed by the 95% isodose line in all cases. At least 95% of the PTV was encompassed by the 95% isodose line. Separate plans were generated and optimized for all ten study patients (Figure 2). All treatment plans were created in accordance with RTOG 0848 treatment planning parameters. The GTV and CTV were held constant in each patient for each of the three plans.
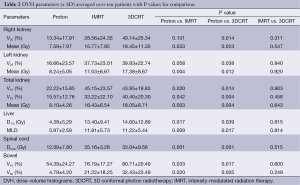
Full table
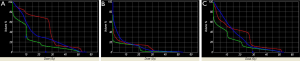
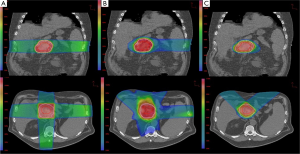
Proton vs. IMRT
First, we compared dose-volume parameters of the IMRT plans with those of proton plans. The proton plans resulted in a lower mean right (7.59 vs. 15.77 Gy, P=0.033), mean left (8.24 vs. 17.03 Gy, P=0.004), and mean total kidney dose (8.10 vs. 16.43 Gy, P=0.003). The mean liver dose was reduced (5.97 vs. 11.81 Gy, P=0.009) as well as the liver D1/3 (4.38 vs. 13.4 Gy, P=0.017). The maximum dose to the spinal cord was also reduced (12.09 vs. 35.16 Gy, P=0.001). IMRT provided better homogeneity (0.43 vs. 0.16), uniformity (1.11 vs. 1.36), and conformity (1.19 vs. 0.74) relative to the proton plans. This was unsurprising given the inverse nature of the IMRT treatment planning.
Protons vs. 3DCRT
The next comparison looked at dose-volume parameters between proton and 3DCRT plans. The proton plans resulted in decreased right, left and total kidney V20 and mean doses. There was a lower kidney V15 seen in the proton plans. The liver D1/3, mean liver dose, maximum spinal cord dose, bowel V15 and V50 were all decreased in the proton plans (Table 2). The 3DCRT plans demonstrated better homogeneity (0.43 vs. 0.16) and better uniformity (1.36 vs. 1.18). The conformity was better in the proton plans (0.74 vs. 1.47).
IMRT vs. 3DCRT
Finally, we compared dose-volume parameters between IMRT plans with 3DCRT plans. There was no significant difference seen in the OAR between these plans. The IMRT plans resulted in better uniformity (1.11 vs. 1.18) and conformity (1.19 vs. 1.47) but there was no difference in homogeneity (0.16 vs. 0.16).
Discussion
The results of our study demonstrate a significant tissue-sparing benefit of proton plans over the IMRT and 3DCRT plans. Target coverage was adequate in each of these treatment planning modalities but the amount of normal tissue irradiated differed among them. Clinically acceptable plans were generated for all ten patients with each of the three treatment modalities. Target coverage was achieved in each plan despite a significant patient-to-patient variation in target volume size and shape. PTV volumes ranged from 530 to 1,460 cc (median 708 cc), suggesting that each of these three modalities could provide clinically acceptable treatment plans at least in terms of target coverage. All target volumes were drawn per RTOG 0848 radiation guidelines with several patients having locally advanced disease (see Table 1). This may explain some of the larger target volumes treated.
Pancreatic tumors arise most frequently in the head of the pancreas, which is typically located to the right of midline. It is not uncommon for one kidney to receive more radiation dose than the other. This is also reflected in our data, which show the right kidney receiving a higher mean dose than the left kidney. The intrinsic properties of proton beams should reduce both total and unilateral kidney dose. Protons have an inherent dosimetric advantage over photons due to their absence of exit dose. This results in a reduction in the volume of kidney receiving low doses of radiation when compared with IMRT and 3DCRT plans (Figure 1C). There is a wide separation of the curves in the low dose region of the DVH, indicating a large difference in the volume of tissue being irradiated by low doses of radiation. The difference begins to dissipate at volumes receiving closer to the prescribed dose. Some studies have reported that 3DCRT plans may cause small increases in kidney volumes exposed to higher doses of radiation (9). On the other hand, it is unsurprising that there was a lack of difference in mean total kidney dose between the IMRT and 3DCRT plans. It would be difficult to avoid the nearest kidney with photon beams given its proximity to the pancreatic target.
Proton beams have a Bragg peak that allow for a lower entrance dose. The Bragg peak, in addition to the characteristic absence of exit dose, results in a lower integral dose delivered to the liver. Our results demonstrate a significantly lower MLD in the proton plans, which is consistent with a lower integral liver dose. Even the liver partial volume doses were significantly lower. Figure 1B shows distinct separation of the curves in the low-dose region of the DVH. In comparison to the kidney DVH, there is a less striking separation of the curves in the liver DVH.
Radiation toxicity to the bowel is another significant matter that has been evaluated in numerous pancreatic irradiation studies. Previous study findings include a significant dose-response relationship for every increment of 5 Gy above ≥15 Gy, as well as a significant volume effect with V15 volumes greater than 150 cm3 (10-12). The dose-volume and fraction-size dependence of bowel toxicity is challenging to interpret given the wide range of toxicities reported in the literature. Modern series reviewed by QUANTEC generally confirm the established dose tolerances commonly used in the clinical setting (13), and institutions commonly impose a V50 <5% to limit late toxicities such as obstruction and perforation.
In our study, the volume of irradiated bowel was lower in the proton plans at both low (V15) and high (V50) dose regions. Proton beam characteristics, along with the flexibility to select a number of beam arrangements, resulted in a lower integral bowel dose. Looking at Figure 1A it is evident that there is a large volume of bowel receiving a low dose in all three plans. The greatest difference in volume irradiated occurred at the lower dose region of the DVH. However, given that the proton plans also have a significantly lower V50 volume than the 3DCRT (4.79% vs. 32.43%, P=0.005) plans, one clinical implication may be that proton therapy offers a means of improving the therapeutic ratio. The bowel is a large organ and is difficult to avoid when treating the post-operative pancreatic target.
The IMRT and 3DCRT plans generate relatively large low-dose regions in comparison to proton plans. In our study, there was no significant difference between the volumes of low-dose regions treated by the IMRT and 3DCRT plans. This was indicated by the lack of a significant difference between the MLD, V15 bowel and V15 kidney parameters. In contrast, the proton plans resulted in significantly smaller low-dose regions in comparison to IMRT. Again, the lack of exit dose seen in proton plans contributes to a much smaller integral dose. The optimal radiotherapy technique for pancreatic cancer, however, is still unique for each patient.
Several studies have been performed comparing these treatment modalities focusing on plan optimization and target coverage (3,14). Nichols et al. compared IMRT and proton radiotherapy plans on eight patients with resected pancreatic head cancers (3). They found that the proton plans met all normal tissue constraints and were isoeffective with the corresponding IMRT plans in terms of PTV coverage. However, the proton plans offered significantly reduced exposure to the bowel, stomach, and right kidney. Bouchard et al. compared IMRT and proton radiotherapy as a means of dose escalation for pancreatic cancer. They concluded that the optimal modality for dose escalation still depended on the pancreatic tumor position in relation to OAR anatomy (14). Hypofractionated proton treatment regimens have also been investigated, both as part of concurrent or neoadjuvant treatment. Preliminary findings from these studies show dosimetrically feasible results with tolerable toxicities and acceptable target coverage (9,15). It appears that proton radiotherapy may warrant further investigation as a means of improving the therapeutic ratio.
The aim of our current study was to compare radiation exposure to normal tissues while using IMRT or 3DCRT to treat pancreatic cancer per RTOG 0848. There was consistent overlap between the PTV and OAR so that no one technique could simultaneously achieve full target coverage while fully respecting OAR constraints. Furthermore, the size and extent of the target volume may preclude the use of certain modalities. Nonetheless, significant conclusions may still be drawn from these generated plans, in which full target coverage was obtained with reasonable uniformity and conformity. Another limitation of the current study was the lack of motion management. However, both motion management and daily IGRT are not required per RTOG 0848. The abdomen is a very mobile part of the body, which creates many challenges with normal breathing motion. Target volume motion during respiration may significantly affect beam selection during the planning process.
In conclusion, there was no difference between the IMRT and 3DCRT plans in dose delivered to the kidneys, liver, or bowel. The proton plans did, however, consistently deliver lower mean total kidney doses, mean liver doses, and liver D1/3 compared to the IMRT plans. The proton plans also gave less mean liver dose, liver D1/3, bowel V15, and bowel V50 in comparison to the 3DCRT plans.
Acknowledgements
Disclosure: The authors declare no conflict of interest.
References
- Jemal A, Siegel R, Ward E, et al. Cancer statistics, 2006. CA Cancer J Clin 2006;56:106-30. [PubMed]
- Yovino S, Poppe M, Jabbour S, et al. Intensity-modulated radiation therapy significantly improves acute gastrointestinal toxicity in pancreatic and ampullary cancers. Int J Radiat Oncol Biol Phys 2011;79:158-62. [PubMed]
- Nichols RC Jr, Huh SN, Prado KL, et al. Protons offer reduced normal-tissue exposure for patients receiving postoperative radiotherapy for resected pancreatic head cancer. Int J Radiat Oncol Biol Phys 2012;83:158-63. [PubMed]
- Iori M, Cattaneo GM, Cagni E, et al. Dose-volume and biological-model based comparison between helical tomotherapy and (inverse-planned) IMAT for prostate tumours. Radiother Oncol 2008;88:34-45. [PubMed]
- Whitelaw GL, Blasiak-Wal I, Cooke K, et al. A dosimetric comparison between two intensity-modulated radiotherapy techniques: tomotherapy vs dynamic linear accelerator. Br J Radiol 2008;81:333-40. [PubMed]
- McIntosh A, Read PW, Khandelwal SR, et al. Evaluation of coplanar partial left breast irradiation using tomotherapy-based topotherapy. Int J Radiat Oncol Biol Phys 2008;71:603-10. [PubMed]
- Sheng K, Molloy JA, Larner JM, et al. A dosimetric comparison of non-coplanar IMRT versus Helical Tomotherapy for nasal cavity and paranasal sinus cancer. Radiother Oncol 2007;82:174-8. [PubMed]
- Joseph KJ, Syme A, Small C, et al. A treatment planning study comparing helical tomotherapy with intensity-modulated radiotherapy for the treatment of anal cancer. Radiother Oncol 2010;94:60-6. [PubMed]
- Kozak KR, Kachnic LA, Adams J, et al. Dosimetric feasibility of hypofractionated proton radiotherapy for neoadjuvant pancreatic cancer treatment. Int J Radiat Oncol Biol Phys 2007;68:1557-66. [PubMed]
- Baglan KL, Frazier RC, Yan D, et al. The dose-volume relationship of acute small bowel toxicity from concurrent 5-FU-based chemotherapy and radiation therapy for rectal cancer. Int J Radiat Oncol Biol Phys 2002;52:176-83. [PubMed]
- Tho LM, Glegg M, Paterson J, et al. Acute small bowel toxicity and preoperative chemoradiotherapy for rectal cancer: investigating dose-volume relationships and role for inverse planning. Int J Radiat Oncol Biol Phys 2006;66:505-13. [PubMed]
- Ito Y, Okusaka T, Kagami Y, et al. Evaluation of acute intestinal toxicity in relation to the volume of irradiated small bowel in patients treated with concurrent weekly gemcitabine and radiotherapy for locally advanced pancreatic cancer. Anticancer Res 2006;26:3755-9. [PubMed]
- Emami B, Lyman J, Brown A, et al. Tolerance of normal tissue to therapeutic irradiation. Int J Radiat Oncol Biol Phys 1991;21:109-22. [PubMed]
- Bouchard M, Amos RA, Briere TM, et al. Dose escalation with proton or photon radiation treatment for pancreatic cancer. Radiother Oncol 2009;92:238-43. [PubMed]
- Hong TS, Ryan DP, Blaszkowsky LS, et al. Phase I study of preoperative short-course chemoradiation with proton beam therapy and capecitabine for resectable pancreatic ductal adenocarcinoma of the head. Int J Radiat Oncol Biol Phys 2011;79:151-7. [PubMed]