Relationship between IL-10 and PD-L1 in esophageal carcinoma tissues and IL-10 down-regulates PD-L1 expression via Met signaling pathway
Introduction
In China, the incidence of esophageal cancer (ESCA) ranks as the fourth most common among cancer-related mortality and is particularly high in provinces of Jiangsu, Henan and Anhui. Worldwide, ESCA is the eighth most common cancer and its incidence rate is ranked sixth among cancer-related disease with an estimated 456,000 new cases per year (1,2). According to the pathological type, ESCA is mainly divided into esophageal adenocarcinoma (EAC) and esophageal squamous cell carcinoma (ESCC). In China, more than 90 percent of cases are ESCC. Traditional treatments for ESCA are mainly surgery, radiotherapy and chemotherapy (3,4). However, many patients with advanced disease cannot tolerate these treatment methods. Therefore, new treatment strategies are urgently needed for ESCA.
Programmed death-ligand 1 (PD-L1, also called B7-H1 or CD274) is expressed on the surface of many kinds of tumor cells and tumor-infiltrating immune cells (ICs). PD-L1 over-expression in cancer cells inhibits the activity of T lymphocytes (5,6), which results in the blocking of anti-tumor immunity and immune escape (7). In 2013, the first PD-L1 inhibitor was used in the treatment of lung cancer and achieved amazing clinical results.
In the next few years of drug clinical trials, PD-L1 inhibitors have also achieved good results in the treatment of ESCA (8-10). However, it also has some setbacks during treatment, which predominantly involve side-effects and a low clinical response rate (11-13). Therefore, to improve the clinical efficacy (14,15), the PD-L1 inhibitor is usually combined with chemotherapy (16,17) and/or radiotherapy (16,18,19). Some recent studies have focused on the efficacy of PD-L1 inhibitors combined with immune factors (20-22).
Interleukin 10 (IL-10) is a multifunctional cellular immunosuppressive factor produced by Th2 cells, and plays an important inhibitory role in inflammatory and immunological responses. By inhibiting the activation of natural killer (NK) and T cells, IL-10 enables tumor cells to escape immune surveillance (23,24). However, IL-10 also functions in immune activation, which promotes tumor-specific immune surveillance and reduces the occurrence of pathogenic inflammatory reactions by activating T and NK cells. Therefore, IL-10 plays a key role in this two-way immune regulatory system (25-27). Some articles indicate that the expression level of PD-L1 is correlated with the expression level of IL-10 in the tumor micro-environment (28-32). However, the relationship between both factors remains unclear.
Mesenchymal-epithelial transition factor (Met) is a tyrosine kinase (TK) receptor for hepatocyte growth factor (HGF) (33,34) (Figure 1A). Met has been reportedly expressed in more than 50 percent of ESCC cases at both the mRNA and protein levels. Activation of the Met gene leads to multiple downstream pathways that promote a tumorigenic phenotype (35).
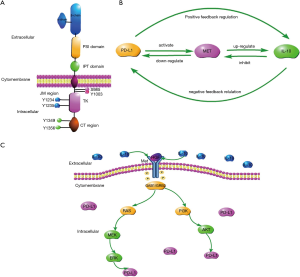
In our study, we found that in esophageal carcinoma tissue, PD-L1 expression levels were positively correlated with IL-10 expression levels. Over-expression of PD-L1 upregulates IL-10 expression in esophageal carcinoma tissue and cells by positive feedback regulation. IL-10 down-regulates the expression levels of PD-L1 in esophageal squamous carcinoma cells-lines by negative feedback regulation. We have also discovered that PD-L1 and IL-10 are linked by the MET signaling pathway (Figure 1B,C).
Thus, our study provides new insights into the functional role of IL-10. The efficacy of combined IL-10 and PD-L1 inhibitor therapy was shown to be better than use of the PD-L1 inhibitor alone.
Methods
Bioinformatics analysis
A bioinformatics analysis was conducted by the TCGA database (https://www.cancer.gov/) and the TCGA-based visualization website GEPIA (http://gepia.cancer-pku.cn/). The expression levels of IL-10, PD-L1 and Met genes were compared in ESCA tissues and normal tissues. The expression correlation of PD-L1 and IL-10 genes of ESCA was analyzed. The analyses also included the relationship between IL-10 and overall survival (OS) or disease-free survival (DFS) or between the expression levels of IL-10 and PD-L1 and pathology stage of ESCA.
Clinical samples
One hundred patients that comprised 60 males and 40 females, with ESCA in the Third Affiliated Hospital of Soochow University from September 2013 to September 2018 were included in the study, which was approved by the Hospital Ethical Review Committee. No patients were treated with any relevant chemotherapy, radiotherapy, targeted therapy, or immunotherapy before surgery. Among 100 ESCA surgical resections, 50 cases were highly differentiated and 50 cases moderately or poorly differentiated. From each patient, three specimens were collected from ESCA tissues, adjacent tissues to the tumor, and normal tissues. The specimens were confirmed by pathological diagnosis as ESCA. A portion of the tissues was embedded in paraffin and another part was freshly resected surgical tissues, and stored in liquid nitrogen for further experiments.
Patient follow-up
Patients were instructed to return for follow-up, including a clinical examination, gastroscopy, thoracic computed tomography, and abdominal ultrasonography, at 3–6 months intervals for one year, and then every 6–12 months thereafter.
Immunochemistry
The paraffin-embedded tumor samples were sectioned to 5 µm slices. The tissue section slides were deparaffinized and rehydrated. For PD-L1, IL-10 and CD8 were stained by corresponding antibodies. Detailed experimental processes can be seen in the supplementary materials. The cell intensity and the rate of positive cells were recorded (Table S1). For data analysis, the final scores of less than eight were defined as low expression and scores of eight or more, were defined as high expression.
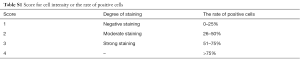
Full table
Quantitative RT-PCR
The tissue pieces were first cut into small sections, and then a homogenizer was used to extract the total RNA using the Trizol method. We compared the differences in IL-10, PD-L1, and Met mRNA expression among cancerous tissues, adjacent tissues, and normal tissues by quantitative RT-PCR using the described primers (please see Table S2). Detailed experimental processes can be seen in the supplementary materials.
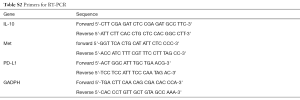
Full table
Cell culture
Human esophageal carcinoma Eca109 and TE-1 cell-lines were obtained from the Cell Research Center, Third Affiliated Hospital of Soochow University for in vitro studies. Detailed culture conditions can be found in the supplementary materials.
SiRNA construction of esophageal squamous cell-lines
We used products of obtained from the Gene Pharma Company to build siMET and siPD-L1. Detailed experimental process can be seen in the supplementary materials. SiRNA sequences are listed in Table S3.

Full table
Over-expression of PD-L1
The ESCA cell line over-expressing PD-L1 (lentivirus-mediated) is from Cell Research Center, the Third Affiliated Hospital of Soochow University. In the article, it is abbreviated as LV-PD-L1.
ELISA
One day before drug treatment, cells in logarithmic growth phase were removed and seeded on a six-well plate at a density of 3×104–105 per well. When the cells reach 70% to 80% confluency, add different concentrations of IL-10 (Creative BioMart, New York, USA) or anti-IL-10 antibody (Creative Biolabs, New York, USA) to the cells. When the cells were cultured for 24 to 48 hours, the cells were counted by a hemocytometer to ensure that the number of cells per well was approximately the same. PD-L1 Quantikine ELISA kit (R&D systems, Minneapolis, MN, USA) was used to test the PD-L1 expression level in cell culture supernate or cell lynate of after 24 and 48 h.
At the same time, after successful transfection of siPD-L1 Eca109, siPD-L1 TE-1, and LV PD-L1 Eca109, LV PD-L1 TE-1 cell culture supernatant was taken to test the IL-10 expression level after 24, 48 hours. Human IL-10 high sensitivity ELISA kit (Multi Sciences, Hangzhou, Zhejiang, China) was used to test the IL-10 expression level.
CCK8
We used the CCK8 assay (Dojindo, Tokyo, Japan) to detect the proliferation of cells after treatment with Crizotinib (Cell Signaling Technology, Danvers, MA, USA) and Crizotinib combined with IL-10. Lastly, we added Crizotinib or Crizotinib combined with IL-10 to the constructed siMet Eca109 and siMet TE-1. The proliferation of cells was detected by CCK8. Detailed experimental processes can be seen in the supplementary materials
Transwell test
Analysis of tumor cell migration and invasion was performed by a transwell chamber assay (Corning, New York, USA). About 2–5×103 cells, Eca109 or siMet-Eca109 cells and TE-1 or siMet-TE-1 cells, were seeded onto the upper chamber in serum-free medium, and then 500 µL of medium containing 1% FBS was added to the lower chamber. Crizotinib or crizotinib combined with IL-10 were added to the lower chamber. After 24 or 48 h incubation, the cells were fixed by incubating in 4% formaldehyde at room temperature for 5 min. After staining with crystal violet, the number of cells that migrated or invaded were observed under the light microscope.
Western blots
PD-L1 expression levels, MET signaling pathway-related molecules (MET and phospho-MET) and their downstream MAPK signaling pathway-related molecules (akt, phospho-akt, Mek, phospho-Mek, Erk, phospho-Erk) were analyzed by Western blotting. Meanwhile, we performed Western immunoblotting on ESCA cells that over-expressed or displayed knocked-down PD-L1 gene expression to detect differences in IL-10 expression levels.
After that, crizotinib or crizotinib combined with IL-10 were added to the cells. Met signaling pathway-related molecules (Met and phospho-Met) were analyzed. In our tissue experiments, the tissue pieces were first cut into small sections, and then a homogenizer was used to extract tissue protein using the protein extraction agent. We compared the differences in IL-10, PD-L1, and Met expression among cancerous tissues, adjacent tissues, and normal tissues. Detailed experimental processes can be seen in the supplementary materials.
Statistical methodology
Quantitative data were presented as mean ± SD. Comparison of the differences between the two groups of data used the χ2 test. Kaplan-Meier curves were generated for OS and DFS. SPSS version 25.0 statistical software was used to assess Cox regression analyses. Alpha values of P<0.05 were considered statistically significant. All data was analyzed by SPSS version 25.0 or Graphpad 5.0 software. Western blotting gray values were analyzed by Image J software.
Results
Bioinformatics analysis results
The gene expression levels of PD-L1, IL-10 and Met in ESCA tissues tend to higher than those in normal tissues. There was no statistically significant difference for either PD-L1 or IL-10 (P≥0.01) (Figure 2A,B), although a statistical difference was noted for Met (P<0.01) (Figure 2C). There was a positive correlation between PD-L1 (CD274) and IL-10 expression (Figure 2D), and the expression level of the IL-10 gene did not affect OS or DFS of patients with ESCA (Figure 2E,F). IL-10 gene expression was associated with the pathological stage of ESCA (P=0.0328; Figure 2G). PD-L1expression was not associated with the pathological stage of ESCA (P=0.423; Figure 2H).
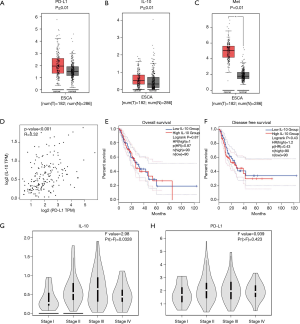
Patient characteristics and immunohistochemistry
The demographic and clinicopathologic characteristics of 100 ESCA patients are summarized in Tables 1 and 2. By the immunohistochemistry (IHC) evaluation, PD-L1 was mainly expressed in the membrane and cytoplasm (Figure 3A,B) and IL-10 in the cytoplasm of cancer cells (Figure 3C,D). CD8 was mainly located on the surface of the T lymphocyte membrane (Figure 3E,F). Among the 100 ESCA cases, 72 cases (72.0%) exhibited high PD-L1 expression levels and 69 cases (69.0%) displayed high IL-10 levels; however, only 15 cases exhibited high expression levels of CD8+ T lymphocyte in tumor tissues. Conversely, 52 cases displayed high expression levels of CD8+ T lymphocyte in adjacent tissues. A comparison of the clinicopathological characteristics according to the PD-L1 expression levels is listed in Table 1. Higher PD-L1 expression levels tended to be related to a patients of a higher age group (P=0.000), poor tumor differentiation (P=0.014), advanced T stage (P=0.000), pathology stage (P=0.001) higher IL-10 expression levels (P=0.038) and higher CD8 expression levels (P=0.008). Higher IL-10 expression levels were associated with poor tumor differentiation (P=0.03), advanced T stage (P=0.001), N pathological stage (P=0.02), the pathologic stage (P=0.000), and higher PD-L1 expression (P=0.000) of ESCA (Table 2).
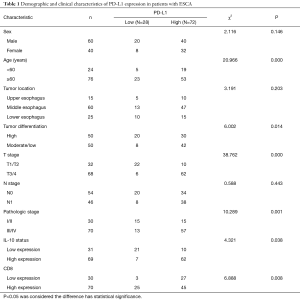
Full table
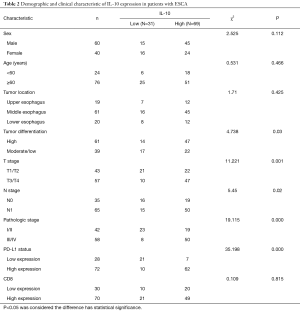
Full table
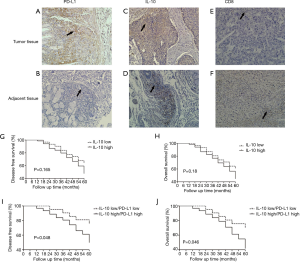
Relationship between the IL-10 expression level and survival periods
The high IL-10 expression level group tended to have shorter survival periods than those with low IL-10 expression levels. The 5-year DFS (P=0.165) and OS rate (P=0.18) in ESCA patients with the IL-10 high expression level was poor as compared those with low expression levels, but without statistical significance (Figure 3G,H). The 5-year DFS (P=0.048) and OS (P=0.046) in ESCA patients with low IL-10/PD-L1 expression levels were longer than those with high IL-10/PD-L1 expression levels (Figure 3I,J) Furthermore, we performed a multi-factor Cox survival analysis on IL-10/PD-L1, T stage, and N stage, and showed that the combination of IL-10 and PD-L1 was an independent prognostic factor, just as the T stage and N stage are equally known (Table 3).
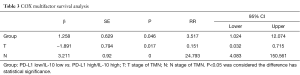
Full table
Positive correlation between IL-10 and PD-L1 expression levels in tissues
Among the 100 ESCA cases, the high expression level of PD-L1 was observed in 72 tumor tissues (72.0%) and in 25 adjacent tissues (25.0%). In tumor tissues, the expression of PD-L1 was significantly higher than that of adjacent tissues (P=0.000; Table 4). Meanwhile, the high expression levels of IL-10 were observed in 69 tumor tissues (69.0%) and in 28 adjacent tissues (28.0%). In tumor tissues, the expression of IL-10 was significantly higher than that of adjacent tissues (P=0.000; Table 5). By Pearson correlation analysis, there was a positive correlation between the expression levels of PD-L1 and IL-10 in tumor and adjacent tissues (Figure 4A,B), and a negative correlation was found between the expression levels of PD-L1and CD8 in tumor and adjacent tissues (Figure 4C,D). Moreover, when analyzing both mRNA by PCR and protein expression by Western blots, we found expression levels of PD-L1 and IL-10 in tumor tissues were higher than those found in adjacent and normal tissues, and the expression levels of Met in the tumor tissues were higher than those found in adjacent tissues (Figure 4E,F,G,H).

Full table

Full table
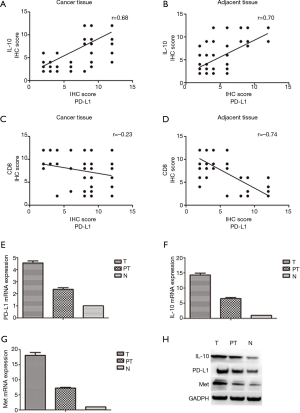
Correlation between IL-10 and PD-L1 expression levels in ESCA cell-lines
ELISA studies showed that in both Eca109 and TE-1 cells, the over-expression of PD-L1 expressing cells increased the IL-10 expression levels. The IL-10 expression levels in siPD-L1 Eca109 and TE-1 cells was lower than that found in the LV-PD-L1 and control group (Figure 5A,B) . The IL-10 expression level was time-dependent. Detection by Western blots showed that the knockdown of PD-L1 (siPD-L1) decreased IL-10 levels, and yet the over-expression of PD-L1 (LV-PD-L1) increased the levels and appearance of IL-10, Met and phospho-Met cells, when compared with control cells (Figure 5C,D) .
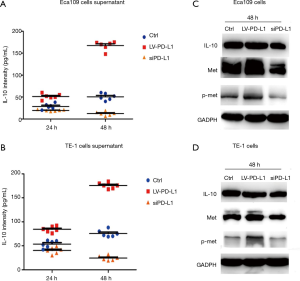
Detecting by ELISA, PD-L1 was expressed in both cell culture supernatant and cell lysates of Eca109 and TE-1 cells. The PD-L1 expression level was significantly higher in cell lysates than that found in the supernatant (Figure 6A,B,C,D). At the protein level, IL-10 inhibited PD-L1 expression in both cell-lines, and anti-IL-10 antibody promoted the expression of PD-L1 in both cell-lines (Figure 6A,B,C,D,E,F). IL-10 acted by inhibiting the Met signaling pathway and the downstream MAPK signaling pathway (Figure 6E,F). Knockdown of Met gene expression in both cell-lines had no significant effect on PD-L1 expression levels after different treatments (Figure 6G). The above phenomena were concentration and time-dependent.
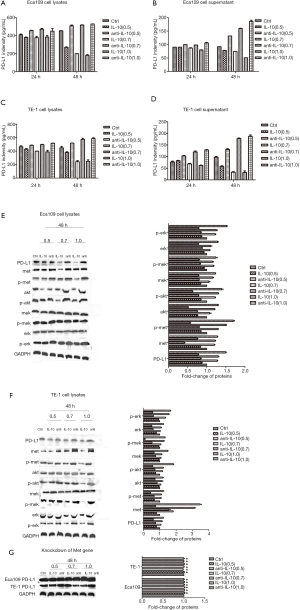
Effect of IL-10 and crizotinib on proliferation, migration and invasion of ESCA cells
When compared with crizotinib treatment alone, the combination of IL-10 and crizotinib more potently inhibited migration and invasion of ESCA cells (Figure 7A,B). After knockdown of the Met gene, there was no significant difference found in terms of the migration and invasion of ESCA cells when comparing any of the treatments with combination therapy of both drugs and crizotinib alone (Figure 7C,D). Moreover, Western blot assay showed that the combination of crizotinib and IL-10 was more potent at inhibiting PD- L1 expression than use of crizotinib alone. This effect worked via the Met signaling pathway (Figure 7E,F). Similarly,the combination of IL-10 and crizotinib more potently inhibited proliferation of ESCA cells (Figure 8A,B). After knockdown of the Met gene, there was no significant difference found in terms of the proliferation of ESCA cells (Figure 8C,D).
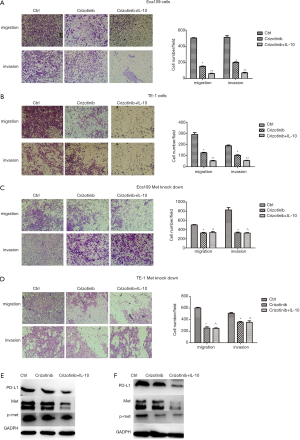
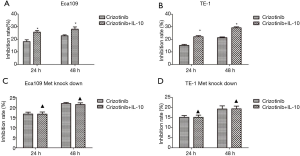
Discussion
Bioinformatics is a new subject and plays an important role in current cancer research for studying the collection, processing, storage, dissemination, analysis and interpretation of biological information. TCGA is a cancer gene database developed by the National Cancer Institute and the National Human Genome Research Institute in the United States. Using the relevant database, we have found a correlation between the expression levels of PD-L1 and IL-10 in patients with esophageal carcinoma (ESCA), which provides novel ideas and a basis for our experimental research.
We also discovered that higher IL-10 expression levels are associated with poor tumor differentiation, advanced T stage, N stage pathological stage, advanced pathologic stage, and higher PD-L1 expression of ESCA. ESCA patients with high IL-10 expression levels usually had a shorter OS and DFS, but with low IL-10 expression levels had longer OS and DFS. However IL-10 is not an independent prognostic factor in patients with ESCA. The results did not match the results of a previous study by Yang’s study. (36).
Our study showed that IL-10 cannot be an independent factor. On the other hand, higher PD-L1 expression levels are associated with higher age, poor tumor differentiation, advanced T stage, pathology stage, higher IL-10 expression levels and higher CD8 expression levels. In addition, PD-L1 combined with IL-10 might be an independent prognostic factor in patients with ESCA. Patients in the low IL-10/low PD-L1 group had an improved prognosis than those in the high IL-10/high PD-L1 group. Results were consistent with prior studies (37-39).
At the transcriptional and translational levels, the higher expression of IL-10 and PD-L1 were observed in cancer tissues, when compared with those in normal tissues, which is consistent with previous studies (40-42). In tumor and adjacent tissues, the PD-L1 expression level was positively correlated with IL-10 expression levels, and was negatively correlated with the CD8+ T cells. Moreover, in cancer tissues, the expression levels of Met at both the transcriptional and translational levels was significantly higher than those in adjacent tissues.
Most of our experimental results are consistent with bioinformatics analysis and have shown that the expression levels of PD-L1, IL-10 and Met in ESCA tissues were higher than those in normal tissues. PD-L1 and IL-10 expression levels were positively correlated. Higher IL-10 expression levels are associated with advanced pathological staging of ESCA. However, some results are inconsistent with bioinformatics analysis. The survival analysis has shown that survival times of patients with higher IL-10 expression levels were shorter than those with lower IL-10 expression levels—although the results were not statistically significant. In our study, we found that higher PD-L1 expression levels are associated with advanced pathological staging of ESCA. The result is different from TCGA. These different reasons might be best described as follows. First, the TCGA database generally collects tumor information from European and American research studies. Differences in expression levels of related factors were due to ethnic differences. Second, the TCGA database generally uses high-throughput sequencing to detect the expression at the gene level, which might not be different at the protein level. Third, due to the difference in sample numbers, data reported in the current study was different from the results of TCGA.
In tissue experiments, we found that the expression levels of PD-L1 and IL-10 were higher in cancer tissues than in adjacent tissues. There was also a positive correlation between the two factors. Similarly, in cellular experiments, we found that over-expression of PD-L1 can over-activate the Met signaling pathway, thereby up-regulating IL-10 expression. Thus, we infer that whether in cancer tissues or cells, over-expressed PD-L1 can activate the Met signaling pathway via positive feedback regulation, thereby up-regulating IL-10 expression. Instead, we also found that IL-10 down-regulates the expression of PD-L1, and anti-IL-10 up-regulates the expression of PD-L1 through the Met signal pathway. Thus we infer that IL-10 can down-regulate PD-L1 expression by inhibiting the Met signaling pathway and the down-stream MAPK signaling pathway via negative feedback regulation (Figure 1B,C).
Some previously published studies suggest that IL-10 is an immunological negative regulator and inhibits the function of T, B and dendritic cells and up-regulates PD-L1 expression (28-30,43). Recent data have shown that IL-10 release might be mediated following PD-L1 blockade, which sustains immunosuppression in ovarian cancer (29). HGF/MET signaling is essential for cancer survival (44) and important in the interaction between IL-10 and PD-L1, which is involved in the immune response, including an increase in IL-10Jeny (45-47) and PD-L1 which co-localizes with MET (48). Thus, in our results, IL-10 down-regulates PD-L1 expression, and does so probably by blocking the Met signaling pathway and the downstream MAPK signaling pathway. When the Met gene was knocked down, we observed no significant differences in the PD-L1 expression levels in different intervention groups. The results demonstrated that the Met signaling pathway plays an important role between IL-10 and PD-L1. The role of IL-10 is complex and multifaceted. IL-10 promotes immune activation, increases tumor-specific immune surveillance and reduces the occurrence of pathogenic inflammatory reactions by activating T and NK cells.
Reportedly, IL-10 inhibits IC function and up-regulates PD-L1 expression (28-30,43). However, our study suggests that IL-10 activates IC function, inhibits the Met signaling pathway, and down-regulates PD-L1 expression in ESCA cells. The results provide new mechanisms concerning the interaction between IL-10 and PD-L1, which might benefit clinical applications in the near future. Therefore, more in vivo and in vitro experiments are arranged to determine the involved modes and mechanisms of action. Our research of course, has still much follow-up work with a deeper exploration of the molecular mechanism of this phenomenon being required.
Supplementary
Immunochemistry
The paraffin-embedded tumor samples were sectioned to 5 µm slices. The tissue section slides were deparaffinized and then rehydrated. For PD-L1 and IL-10 immunochemical staining, antigen retrieval was achieved in boiled EDTA for 30 min. The tissue section slices were incubated with peroxidase blocking reagent (3% H2O2 solution) for 30 min. The slices were incubated with the primary PD-L1 antibody (Novus ittleton, Colorado, USA) and IL-10 antibody (Novus, Littleton, Colorado, USA) in a humidified chamber overnight at 4 °C. After being washed three times in PBS, the slices were then incubated with anti-mouse/rabbit secondary antibody (Maixin, Fuzhou, Fujian, China) in a dedicated chamber for 30 min. We than stained the slices with hematoxylin solution.
The results were evaluated by two independent pathologists at the Third Affiliated Hospital of Suzhou University. The intensity was classified as follows: 0, negative staining; 1, weak staining; 2, moderate staining; 3, strong staining. The rate of positive cells was recorded: 1: 0–25%; 2: 26–50%; 3: 51–75%; and 4: greater than 75%. A final score was achieved by multiplying the staining intensity (0, 1, 2, and 3) and the rate of positive cells observed (1, 2, 3 and 4). For data analysis, scores of less than 8 were defined as low expression and scores of 8 or more, as high expression.
Quantitative RT-PCR
The tissue pieces were first cut into small pieces, and then a homogenizer was used to extract total RNA using the Trizol method. We compared the differences in IL-10, PD-L1, and Met mRNA expression among cancerous, adjacent, and normal tissues. The primers (Sangon, Shanghai, China) were listed in the supplementary material. A Takara (Takara, Tokyo, Japan) RT-PCR Kit were used for this assay.
Cell culture
The esophageal squamous cell-lines Eca109 and TE-1 were from the obtained from the Cell Research Center, Third Affiliated Hospital of Soochow University for in vitro studies. Eca109 cells were cultured in RPMI 1640 medium (Hyclone, Logan City, Utah, USA) and supplemented with 10% fetal calf serum (FCS; Gibco, Thermo-Fisher Scientific, Grand Island, NY, USA), 100 units/mL penicillin, and 100 ug/mL streptomycin. TE-1 cells were cultured in DMEM medium (Hyclone, Logan City, Utah, USA), which was supplemented with 10% FCS, 100 units/mL penicillin, and 100 ug/mL streptomycin. Cells were incubated at 37 °C in a humidified atmosphere containing 5% CO2 in air. Cells were used in the experiments when found to be in the logarithmic growth phase.
SiRNA construction of esophageal squamous cell-lines
One day before transfection, cells in the logarithmic growth phase were removed and seeded on a six-well plate at a density of 104–105 per plate. We used customized siRNA (Gene Pharma Company, Shanghai, China) and configured an X-treme GENE siRNA Transfection Reagent—siRNA plasmid mixture, which was then added to the cells to be transfected. After 36 hours, the transfection effect was detected by PCR. If the transfection efficiency reached above 60 percent, then the cells could be used for the next experiment.
CCK8
One day before drug treatment, cells in the logarithmic growth phase were removed and seeded into a 96-well plate at a density of 2×103 cells per well (100 uL/well). Next, we used CCK8 (Dojindo, Tokyo, Japan) to detect the proliferation of cells after treatment with Crizotinib (Cell Signaling Technology, Danvers, MA, USA) and Crizotinib combined with IL-10. Lastly, we added Crizotinib or Crizotinib combined with IL-10 to the constructed siMet Eca109 and siMet TE-1. The proliferation of cells was detected by CCK8 assay. The inhibition rate is calculated by the following formula: inhibition rate = [(control well-experimental well)/(control well-blank well)] ×100%.
Western blot
Total cellular protein was extracted by a protein extraction agent (KeyGen Biotech, Nanjing, Jiangsu, China) after 48 hours of treatment with different concentrations of IL-10, anti-IL-10 antibody. Protein concentrations were determined by a bicinchoninic acid assay (BCA assay, Pierce, Thermo-Fisher Scientific, Waltham, MA, USA). Aliquots of protein lysates were separated on sodium dodecyl sulfate-polyacrylamide gels (SDS-PAGE) and transferred onto a PVDF membrane, which was blocked with 3% BSA in TBST. The membrane was then hybridized with primary antibodies. Then, with the corresponding secondary antibodies conjugated with horseradish peroxidase, the specific protein bands on the membrane were detected by a chemiluminescence assay (EMD Millipore, Temecula, CA, USA). GADPH was used as a loading control. All primary and secondary antibodies used in the Western blotting assay were purchased from Cell Signaling Technology, Abcam or the Novus Company. PD-L1 expression levels, Met signaling pathway-related molecules (Met and phospho-Met) and their downstream MAPK signaling pathway-related molecules (akt, phospho-akt, Mek, phospho-Mek, Erk, phospho-Erk) were analyzed. In addition, we performed Western blotting on esophageal cancer cells that overexpressed or were knocked down in the expression of the PD-L1 gene to detect differences in IL-10 expression levels. Subsequently, crizotinib or crizotinib combined with IL-10 were added to the cells. Met signaling pathway-related molecules (Met and phospho-Met) were analyzed.
In our tissue experiments, the tissue specimens were first cut into small sections, and then a homogenizer was used to extract tissue protein using the protein extraction reagent. We compared the differences in IL-10, PD-L1, and Met expression among cancerous, adjacent and normal tissues.
Acknowledgments
Funding: None.
Footnote
Conflicts of Interest: All authors have completed the ICMJE uniform disclosure form (available at http://dx.doi.org/10.21037/jgo.2020.01.06). The authors have no conflicts of interest to declare.
Ethical Statement: The authors are accountable for all aspects of the work in ensuring that questions related to the accuracy or integrity of any part of the work are appropriately investigated and resolved. The work was performed in accordance with the local Ethics Committee of the Third Affiliated Hospital of Soochow University (No. 20110140). Written informed consent was obtained from each patient, including signed consent for tissue analysis and consent to be recorded for potential medical research at the time of sample acquisition.
Open Access Statement: This is an Open Access article distributed in accordance with the Creative Commons Attribution-NonCommercial-NoDerivs 4.0 International License (CC BY-NC-ND 4.0), which permits the non-commercial replication and distribution of the article with the strict proviso that no changes or edits are made and the original work is properly cited (including links to both the formal publication through the relevant DOI and the license). See: https://creativecommons.org/licenses/by-nc-nd/4.0/.
References
- Ferlay J, Soerjomataram I, Dikshit R, et al. Cancer incidence and mortality worldwide: sources, methods and major patterns in GLOBOCAN 2012. Int J Cancer 2015;136:E359-86. [Crossref] [PubMed]
- Pennathur A, Gibson MK, Jobe BA, et al. Oesophageal carcinoma. Lancet 2013;381:400-12. [Crossref] [PubMed]
- Kelsen DP, Ginsberg R, Pajak TF, et al. Chemotherapy followed by surgery compared with surgery alone for localized esophageal cancer. N Engl J Med 1998;339:1979-84. [Crossref] [PubMed]
- van Hagen P, Hulshof MC, van Lanschot JJ, et al. Preoperative chemoradiotherapy for esophageal or junctional cancer. N Engl J Med 2012;366:2074-84. [Crossref] [PubMed]
- Pardoll DM. The blockade of immune checkpoints in cancer immunotherapy. Nat Rev Cancer 2012;12:252-64. [Crossref] [PubMed]
- Ribas A. Tumor immunotherapy directed at PD-1. N Engl J Med 2012;366:2517-9. [Crossref] [PubMed]
- Blank C, Mackensen A. Contribution of the PD-L1/PD-1 pathway to T-cell exhaustion: an update on implications for chronic infections and tumor evasion. Cancer Immunol Immunother 2007;56:739-45. [Crossref] [PubMed]
- Ashraf N. Atezolizumab Treatment of Nonsquamous NSCLC. N Engl J Med 2018;379:1187-8. [Crossref] [PubMed]
- Jung KH, LoRusso P, Burris H, et al. Phase I Study of the Indoleamine 2, 3-Dioxygenase 1 (IDO1) Inhibitor Navoximod (GDC-0919) Administered with PD-L1 Inhibitor (Atezolizumab) in Advanced Solid Tumors. Clin Cancer Res 2019;25:3220-8. [Crossref] [PubMed]
- Meindl-Beinker NM, Betge J, Gutting T, et al. A multicenter open-label phase II trial to evaluate nivolumab and ipilimumab for 2nd line therapy in elderly patients with advanced esophageal squamous cell cancer (RAMONA). BMC Cancer 2019;19:231. [Crossref] [PubMed]
- Hamilton G, Rath B. Immunotherapy for small cell lung cancer: mechanisms of resistance. Expert Opin Biol Ther 2019;19:423-32. [Crossref] [PubMed]
- Maughan BL, Bailey E, Gill DM, et al. Incidence of Immune-Related Adverse Events with Program Death Receptor-1- and Program Death Receptor-1 Ligand-Directed Therapies in Genitourinary Cancers. Front Oncol 2017;7:56. [Crossref] [PubMed]
- Raju S, Joseph R, Sehgal S. Review of checkpoint immunotherapy for the management of non-small cell lung cancer. Immunotargets Ther 2018;7:63-75. [Crossref] [PubMed]
- Chowdhury PS, Chamoto K, Honjo T. Combination therapy strategies for improving PD-1 blockade efficacy: a new era in cancer immunotherapy. J Intern Med 2018;283:110-20. [Crossref] [PubMed]
- Esteva FJ, Hubbard-Lucey VM, Tang J, et al. Immunotherapy and targeted therapy combinations in metastatic breast cancer. Lancet Oncol 2019;20:e175-86. [Crossref] [PubMed]
- Bylicki O, Barazzutti H, Paleiron N, et al. First-Line Treatment of Non-Small-Cell Lung Cancer (NSCLC) with Immune Checkpoint Inhibitors. BioDrugs 2019;33:159-71. [Crossref] [PubMed]
- Cyprian FS, Akhtar S, Gatalica Z, et al. Targeted immunotherapy with a checkpoint inhibitor in combination with chemotherapy: A new clinical paradigm in the treatment of triple-negative breast cancer. Bosn J Basic Med Sci 2019;19:227-33. [PubMed]
- Gajiwala S, Torgeson A, Garrido-Laguna I, et al. Combination immunotherapy and radiation therapy strategies for pancreatic cancer-targeting multiple steps in the cancer immunity cycle. J Gastrointest Oncol 2018;9:1014-26. [Crossref] [PubMed]
- Wirsdörfer F, de Leve S, Jendrossek V. Combining Radiotherapy and Immunotherapy in Lung Cancer: Can We Expect Limitations Due to Altered Normal Tissue Toxicity? Int J Mol Sci 2018. [Crossref] [PubMed]
- Hutmacher C, Gonzalo Nunez N, Liuzzi AR, et al. Targeted Delivery of IL2 to the Tumor Stroma Potentiates the Action of Immune Checkpoint Inhibitors by Preferential Activation of NK and CD8 (+) T Cells. Cancer Immunol Res 2019;7:572-83. [Crossref] [PubMed]
- Li J, Xu J, Yan X, et al. Targeting Interleukin-6 (IL-6) Sensitizes Anti-PD-L1 Treatment in a Colorectal Cancer Preclinical Model. Med Sci Monit 2018;24:5501-8. [Crossref] [PubMed]
- Menssen HD, Harnack U, Erben U, et al. Antibody-based delivery of tumor necrosis factor (L19-TNFalpha) and interleukin-2 (L19-IL2) to tumor-associated blood vessels has potent immunological and anticancer activity in the syngeneic J558L BALB/c myeloma model. J Cancer Res Clin Oncol 2018;144:499-507. [Crossref] [PubMed]
- Ip WKE, Hoshi N, Shouval DS, et al. Anti-inflammatory effect of IL-10 mediated by metabolic reprogramming of macrophages. Science 2017;356:513-9. [Crossref] [PubMed]
- Zielinski CE, Mele F, Aschenbrenner D, et al. Pathogen-induced human TH17 cells produce IFN-gamma or IL-10 and are regulated by IL-1beta. Nature 2012;484:514-8. [Crossref] [PubMed]
- Chechlinska M, Kowalewska M, Nowak R. Systemic inflammation as a confounding factor in cancer biomarker discovery and validation. Nat Rev Cancer 2010;10:2-3. [Crossref] [PubMed]
- Danilova AB, Danilov AO, Fakhrutdinova OL, et al. Laboratory evaluation of TGFbeta1, IL-10, VEGF levels in vivo and in vitro in patients with solid tumors. Vopr Onkol 2011;57:759-66. [PubMed]
- Wang DZ, Zhang XH, Wu WX, et al. Exploration of the association of H. pylori and EBV infection with cardiac and distal gastric adenocarcinoma among residents in Cixian County, a high-risk area of esophageal cancer in Hebei province. Zhonghua Zhong Liu Za Zhi 2012;34:446-9. [PubMed]
- Brooks DG, Ha SJ, Elsaesser H, et al. IL-10 and PD-L1 operate through distinct pathways to suppress T-cell activity during persistent viral infection. Proc Natl Acad Sci U S A 2008;105:20428-33. [Crossref] [PubMed]
- Lamichhane P, Karyampudi L, Shreeder B, et al. IL-10 Release upon PD-1 Blockade Sustains Immunosuppression in Ovarian Cancer. Cancer Res 2017;77:6667-78. [Crossref] [PubMed]
- Ruffner MA, Kim SH, Bianco NR, et al. B7-1/2, but not PD-L1/2 molecules, are required on IL-10-treated tolerogenic DC and DC-derived exosomes for in vivo function. Eur J Immunol 2009;39:3084-90. [Crossref] [PubMed]
- Wang J, Rodriquez G, Norcross MA. Control of adaptive immune responses by Staphylococcus aureus through IL-10, PD-L1, and TLR2. Sci Rep 2012;2:606. [Crossref] [PubMed]
- Zhang J, Benedek G, Bodhankar S, et al. IL-10 producing B cells partially restore E2-mediated protection against EAE in PD-L1 deficient mice. J Neuroimmunol 2015;285:129-36. [Crossref] [PubMed]
- Smyth EC, Sclafani F, Cunningham D. Emerging molecular targets in oncology: clinical potential of MET/hepatocyte growth-factor inhibitors. Onco Targets Ther 2014;7:1001-14. [Crossref] [PubMed]
- Zhang Y, Xia M, Jin K, et al. Function of the c-Met receptor tyrosine kinase in carcinogenesis and associated therapeutic opportunities. Mol Cancer 2018;17:45. [Crossref] [PubMed]
- Kashyap MK, Abdel-Rahman O. Expression, regulation and targeting of receptor tyrosine kinases in esophageal squamous cell carcinoma. Mol Cancer 2018;17:54. [Crossref] [PubMed]
- Yang Y, Fa X. Role of IL-10 gene polymorphisms on the susceptibility for esophageal cancer and its association with environmental factors. Int J Clin Exp Pathol 2015;8:9580-5. [PubMed]
- Chen L, Deng H, Lu M, et al. B7-H1 expression associates with tumor invasion and predicts patient's survival in human esophageal cancer. Int J Clin Exp Pathol 2014;7:6015-23. [PubMed]
- Hino R, Kabashima K, Kato Y, et al. Tumor cell expression of programmed cell death-1 ligand 1 is a prognostic factor for malignant melanoma. Cancer 2010;116:1757-66. [Crossref] [PubMed]
- Ito S, Okano S, Morita M, et al. Expression of PD-L1 and HLA Class I in Esophageal Squamous Cell Carcinoma: Prognostic Factors for Patient Outcome. Ann Surg Oncol 2016;23:508-15. [Crossref] [PubMed]
- Imai D, Yoshizumi T, Okano S, et al. IFN-gamma Promotes Epithelial-Mesenchymal Transition and the Expression of PD-L1 in Pancreatic Cancer. J Surg Res 2019;240:115-23. [Crossref] [PubMed]
- Schumacher K, Haensch W, Roefzaad C, et al. Prognostic significance of activated CD8 (+) T cell infiltrations within esophageal carcinomas. Cancer Res 2001;61:3932-6. [PubMed]
- Tsutsumi S, Saeki H, Nakashima Y, et al. Programmed death-ligand 1 expression at tumor invasive front is associated with epithelial-mesenchymal transition and poor prognosis in esophageal squamous cell carcinoma. Cancer Sci 2017;108:1119-27. [Crossref] [PubMed]
- Getts DR, Turley DM, Smith CE, et al. Tolerance induced by apoptotic antigen-coupled leukocytes is induced by PD-L1+ and IL-10-producing splenic macrophages and maintained by T regulatory cells. J Immunol 2011;187:2405-17. [Crossref] [PubMed]
- Papaccio F, Della Corte CM, Viscardi G, et al. HGF/MET and the Immune System: Relevance for Cancer Immunotherapy. Int J Mol Sci 2018. [Crossref] [PubMed]
- Okunishi K, Dohi M, Nakagome K, et al. A novel role of hepatocyte growth factor as an immune regulator through suppressing dendritic cell function. J Immunol 2005;175:4745-53. [Crossref] [PubMed]
- Rutella S, Bonanno G, Procoli A, et al. Hepatocyte growth factor favors monocyte differentiation into regulatory interleukin (IL)-10++IL-12low/neg accessory cells with dendritic-cell features. Blood 2006;108:218-27. [Crossref] [PubMed]
- Shintani Y, Aoki H, Nishihara M, et al. Hepatocyte growth factor promotes an anti-inflammatory cytokine profile in human abdominal aortic aneurysm tissue. Atherosclerosis 2011;216:307-12. [Crossref] [PubMed]
- Balan M, Mier y Teran E, Waaga-Gasser AM, et al. Novel roles of c-Met in the survival of renal cancer cells through the regulation of HO-1 and PD-L1 expression. J Biol Chem 2015;290:8110-20. [Crossref] [PubMed]