This article has an erratum available at: http://dx.doi.org/10.21037/jgo-22-381 the article has been update on 2022-04-21 at here.
LncRNA POT1-AS1 accelerates the progression of gastric cancer by serving as a competing endogenous RNA of microRNA-497-5p to increase PDK3 expression
Introduction
Gastric cancer (GC) is considered to be one of the common malignancy digestive carcinomas (1). This carcinoma is particularly widespread in China (2), where it is considered to be the second most prevalent and third deadliest carcinoma (3). While multiple detailed studies of GC have been undertaken to date, the processes driving the genesis and progression of the disease remain elusive, restricting patients’ access to effective therapeutic options.
Long non-coding RNAs (lncRNAs) have been discovered to be a novel collection of sequences for researching the processes of different types of carcinomas (4-6). LncRNAs contain over 200 nucleotides and exist in the form of a linear transcript (7). As these linear transcripts cannot translate into proteins, they were once thought to be “junk” and “noise” in the genome (8). These non-coding RNAs are linked to various genetic phenomena, including gene expression at the transcriptional level, according to overwhelming evidence (9,10). Various human disorders, including neurodegenerative disorders, malignancies, heart-related complications, and endocrine diseases are linked to lncRNA dysfunction (11). According to the reported studies, in GC, abnormal lncRNA expression is common, and their dysfunction has been linked to GC progression (12-14). Multiple studies have suggested that abnormal lncRNA levels have tumor-promoting or suppressing activities. Furthermore, these RNAs play significant roles in the manipulation of a variety of carcinoma features (15-17). The functional association and expression pattern of POT1-AS1 in GC has remained elusive. In view of this fact, POT1-AS1 was considered as the subject of our investigation. This research may provide new insights into the pathophysiology of GC and may suggest novel therapeutic options. We present the following article in accordance with the MDAR reporting checklist (available at https://dx.doi.org/10.21037/jgo-21-709).
Methods
Patients and tissue specimens
The current study included 132 patients suffering from GC who had never been treated with anticancer treatment. Following surgical removal, snap-freezing of GC tissues and nearby healthy tissues was carried out in liquid nitrogen, followed by storage at cryogenic temperature (−80 °C). Approval for this study was obtained from Changhai Hospital’s research ethics committee (No. 16ZR1400800). Each experiment was performed in accordance with the Declaration of Helsinki (as revised in 2013). Table 1 represents the patient demographics as well as clinical data. All patients provided informed consent.
Table 1
Clinical features | Total | POT1-AS1 expression | P value | |
---|---|---|---|---|
High (N=65) | Low (N=67) | |||
Age (years) | 0.510 | |||
<60 | 38 | 17 | 21 | |
≥60 | 94 | 48 | 46 | |
Gender | 0.302 | |||
Male | 73 | 33 | 40 | |
Female | 59 | 32 | 27 | |
Tumor location | 0.167 | |||
Rectum | 59 | 33 | 26 | |
Colon | 73 | 32 | 41 | |
Tumor size (cm) | 0.001 | |||
<5 | 52 | 16 | 36 | |
≥5 | 80 | 49 | 31 | |
Differentiation grade | 0.176 | |||
Well | 44 | 18 | 26 | |
Moderate + poor | 88 | 47 | 41 | |
TNM stage | 0.008 | |||
I + II | 82 | 33 | 49 | |
III | 50 | 32 | 18 | |
Depth of invasion | 0.083 | |||
T1 + T2 | 61 | 35 | 26 | |
T3 + T4 | 71 | 30 | 41 | |
Lymph node metastasis | 0.001 | |||
No | 82 | 31 | 51 | |
Yes | 50 | 34 | 16 | |
Distant metastasis | 0.913 | |||
No | 101 | 50 | 51 | |
Yes | 31 | 15 | 16 | |
Adjuvant chemotherapy | 0.054 | |||
No | 62 | 25 | 37 | |
Yes | 70 | 40 | 30 | |
CA19-9, kU/L | 0.132 | |||
<40 | 99 | 45 | 54 | |
≥40 | 33 | 20 | 13 |
Pearson chi-square test was used for comparisons between subgroups. CA19-9, carbohydrate antigen 19-9.
Cell culture
A healthy gastric epithelial cell line (RGM-1) and 4 types of GC cell lines (MKN-45, MGC-803, SGC-7901, and HGC-27) were provided by ATCC, Manassas, USA. Cells were then seeded in DMEM supplemented with 10% fetal bovine serum (FBS; heat-inactivated), 100 U/mL penicillin, and 100 mg/mL streptomycin. These antibiotics, DMEM, and FBS were obtained from Gibco. At 37 °C, cells were incubated in a humidified environment with a continuous supply of 5% CO2.
Cell transfection
An siRNA targeting POT1-AS1 (si-POT1-AS1) and non-targeting control siRNA (si-NC) were procured from Guangzhou RiboBio Co., Ltd. for the loss-of-function study. The design and development of miR-497-5p agomir (agomir-497-5p) were carried out by GenePharma Co., Ltd. (Shanghai), and agomir (agomir-NC) was employed as a negative control. Endogenous miR-497-5p expression was silenced using miR-497-5p antagomir (antagomir-497-5p), and antagomir-NC was utilized as a control for antagomir-497-5p. GenePharma also produced the PDK3 overexpression vector pcDNA3.1-PDK3 (pc-PDK3) and the empty pcDNA3.1 vector. One day before transfection, cell seeding was carried out in 6-well plates, followed by transient transfection of the molecular products using Lipofectamine 2000TM reagent (Invitrogen). Si-NC: 5'-UUCUCCGAACGUGUCACGUGGC-3'; Si-POT1-AS1: 5'-UCCCCCCCTGCTGGUCAGAGAC-3'.
RT-qPCR
The content and quality of total RNA were determined via NanoDrop, Thermo Fisher Scientific, Inc. after isolation using TRIzol® reagent (Invitrogen). To assess the miR-497-5p expression, complementary DNA (cDNA) was obtained using a Mir-XTM miRNA First-Strand Synthesis Kit, followed by qPCR with a Mir-XTM miRNA qRT-PCR TB Green® Kit. For normalizing miR-497-5p expression, U6 snRNA was considered as an internal control. cDNA was obtained from total RNA using the PrimeScriptTM RT Reagent Kit to measure POT1-AS1 and PDK3 mRNA expression, followed by amplification of the cDNA products via SYBR-Green PCR Master Mix. These kits and PCR Master Mix were procured from TaKaRa Bio Inc. Actin expression was used to normalize the levels of POT1-AS1 and PDK3 mRNA. The 2−ΔΔCq technique was used to examine the expression of each gene.
POT1-AS1: F: 5'-AGATCTAGGCTTCGGAAGGAAAAGC-3', R: 5'-CCCAAGATCTAGCGCACCGCCTA-3'; PDK3: F: 5'-AGATCTAGATCTCGTCCACTGAAGGCACAAAAGC-3', R: 5'-CCCAAGCTTAAGCTTGGCGCACAGACCCGCCTA-3'; U1 F: 5'-GGCCACACTGACTTCTCAAAAT-3', R: 5'-GGCTGGCACATACTGCGGG-3'; GAPDH: F: 5'-GGAGCGAGATCCCTCCAAAAT-3', R: 5’-GGCTGTTGTCATACTTCTCATGG-3'; miR-497-3p: F: 5'-AACAGTGCAAACCACACTGTG-3', R: 5'-GTCGTATCCAGTGCAGGGT-3'; U6: F: 5'-GCTTCGGCAGCACATATACTAAAAT-3', R: 5'-CGCTTCACGAATTTGCGTGTCAT-3'.
CCK-8 assay
To assess the proliferative ability of the cultured cell lines, a CCK-8 assay was employed. After overnight incubation, transfected cells were exposed to trypsin. Next, cells (2×103 cells/well) were grown in each well of a 96-well plate, followed by adding CCK-8 solution (10 µL), and then 2 h incubation was carried out at 37 °C. A microplate reader (Bio-Rad) was employed for the measurement of optical density (OD) at 450 nm.
Transwell assays
Cell digestion was carried out using trypsin 48 h post transfection, followed by washing the cells (twice) with PBS. For the evaluation of the invasive properties of cells, transfected cells (5×104) were re-suspended in DMEM (200 µL) and then added into each well of the upper portion of a Transwell insert (8-µm pore; Corning) with Matrigel® precoated membranes (BD Biosciences). Next, 600 µL of 10% FBS cultured media was added to the lower part. For 24 h, the Transwell inserts were incubated in a humidified environment with a continuous supply of 5% CO2 (at 37 °C). The noninvasive cells (on the upper insert portion) were then cleaned (using a cotton swab), while fixation of the invasive cells was carried out in pure methanol, followed by staining with 0.1% crystal violet (Sigma-Aldrich; Merck KGaA) for 10 min at room temperature. The method for assessing cell migration was the same as above, however, Matrigel® was not used for membrane precoating. An Olympus microscope (Olympus Corporation) was used to image the migratory as well as invasive cells, followed by evaluating their migratory and invasive potential.
Bioinformatics evaluations
StarBase-3.0 (http://starbase.sysu.edu.cn/) was employed for predicting the possible POT1-AS1 targets.
RNA Immunoprecipitation (RIP) assay
RIP assay was utilized to examine the binding between POT1-AS1 and miR-497-5p in GC cells via the RIP Kit (Millipore). Next, the cells were exposed to lysis buffer, and then the cell lysates were treated with magnetic beads coated with human antibodies AGO2 or IgG (as a negative control) for 24 h (at 4 °C). Subsequently, the collection of magnetic beads was carried out and then immunoprecipitated RNA was isolated. Finally, RT-qPCR was performed to evaluate the enrichment of POT1-AS1 and miR-497-5p.
LncRNA localization
The isolation and extraction of nuclear and cytoplasmic RNA of the indicated GC cell lines were carried out using the Cytoplasmic and Nuclear RNA Purification Kit (Amyjet) to localize lncRNA. Next, qRT-PCR was conducted to identify the expression of POT1-AS1. The cytoplasmic and nuclear controls were GAPDH and U1, as respectively performed (18).
Luciferase reporter assay (LRA)
Shanghai GenePharma developed and produced POT1-AS1 fragments with wild-type (WT) and mutant (MUT) miR-497-5p interacting sites. The WT-POT1-AS1 and MUT-POT1-AS1 reporter plasmids were generated and then the reporter plasmids for WT-PDK3 and MUT-PDK3 were also made in the same way, followed by growing the cells in 24-well plates to 60–70% confluency. In the presence of agomir-NC or agomir-497-5p, cultured cells were treated with WT or MUT reporter plasmids, followed by collecting the plasmid-treated cells 48 h post transfection, and their activity was then assessed with a DLR Assay System (Promega Corporation). The normalization of data was carried out with Renilla luciferase activity.
Western blotting (WB)
Using RIPA buffer, proteins were extracted from cultured cells, followed by measuring the protein concentration via a bicinchoninic acid assay kit. The above buffer and kit were procured from the Beyotime Institute of Biotechnology. For the separation of an equal amount of protein, the obtained proteins were processed on SDS-PAGE, followed by transferring these proteins to PVDF membranes (Millipore). Next, skim milk powder (5% solution) was used to block the membrane (for 2 h) at ~25 °C. Overnight incubation of the membrane was carried out with mouse anti-human PDK4 (1:1,000; ab182408, Abcam) and Actin (1:1,000; ab128915, Abcam) primary antibodies at 4 °C, followed by incubating the membrane with secondary antibodies conjugated to HRP (1:5,000; cat. No. ab205718, Abcam) for 2 h. Protein signals were identified using an HRP Substrate kit (EMD Millipore).
Statistical analysis
The obtained data were represented as mean ± SD. Each experimental procedure was conducted 3 times in an independent manner. The relationships between POT1-AS1 expression and clinical parameters in GC patients were assessed with the χ2 test, followed by comparing groups via Student’s t-test or one-way ANOVA. Next, Tukey's post hoc test was performed for the comparison of variations among groups. The Kaplan–Meier (K-M) method was used to calculate the overall survival (OS) rate of GC patients, and the log-rank (L-R) test was used to compare the results. Spearman's correlation analysis was performed to examine the link between the expressions of 2 genes in GC tissues. P value <0.05 was considered to be statistically significant.
Results
POT1-AS1 is increased in GC
We used RT-qPCR to examine the expression profile of POT1-AS1 in 132 pairs of GC tissues as well as neighboring normal tissues to identify the role of POT1-AS1 in GC. The obtained data indicated high expression of POT1-AS1 in GC tissues compared with nearby healthy tissues, as depicted in Figure 1A (P<0.05). RT-qPCR was conducted to evaluate the expression of POT1-AS1 in 4 GC cell lines (MGC-803, SGC-7901, MKN-45, and HGC-27) and a healthy gastric epithelial cell line (RGM-1) to further support the aforementioned data. In GC cells, POT1-AS1 expression was elevated compared with RGM-1 cells, as depicted in Figure 1B (P<0.05 vs. RGM-1). We evaluated the clinical implications of POT1-AS1 in GC after confirming the abnormal upregulation of POT1-AS1. The median value of POT1-AS1 expression in GC tissues was used as the cut-off value, and each GC patient in this investigation was separated into 2 groups: low POT1-AS1 expression and high POT1-AS1 expression. Elevated expression of POT1-AS1 was significantly linked with larger tumor size (P=0.001), lymph node metastasis (P=0.001), and better TNM stage (P=0.008) in GC patients, as represented in Table 1. The GEPIA software program was employed to validate the link between the expression of POT1-AS1 and the outcomes of 358 patients with GC from TCGA, and the obtained data revealed that elevated expression of POT1-AS1 was significantly linked with poor OS [n=373, HR =1.6, P(HR) =0.0057, L-R P=0.0054] and disease-free survival (DFS) [n=373, HR =2.1, P(HR) =0.00021, L-R P=0.00015] in CRC, as depicted in Figure 1C,1D. These findings reveal that POT1-AS1 may have a role in GC progression.
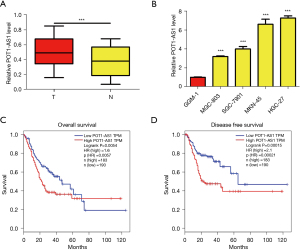
Silencing of POT1-AS1 attenuates the progression of GC cells in vitro
POT1-AS1 expression was elevated in MKN-45 and HGC-27 cells relative to MGC-803 and SGC-7901 cells. To identify the function of POT1-AS1 in GC development, POT1-AS1 was depleted in cells (MKN-45 and HGC-27) via transfection with si-POT1-AS1, as represented in Figure 2A. A control group of cells was transfected with si-NC. The obtained data of the CCK-8 assay revealed that MKN-45 and HGC-27 cells transfected with si-POT1-AS1 had considerably decreased proliferative potential than cells transfected with si-NC, as depicted in Figure 2B. Furthermore, a considerable decrease was found in the migratory and invasive potential of POT1-AS1-silenced MKN-45 and HGC-27 cells relative to si-NC-transfected cells, as represented in Figure 2C,2D. These data suggest that POT1-AS1 is a cancer-enhancing lncRNA in GC.
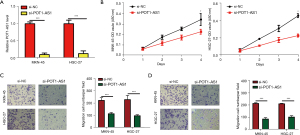
POT1-AS1 directly binds with miR-497-5p in GC cells as a molecular miRNA sponge
Several biological activities have been linked with lncRNAs, including acting as competing endogenous RNAs (ceRNAs) for miRNAs, according to new findings. The subcellular location of POT1-AS1 was initially determined to evaluate whether it could be a ceRNA. The results of the nuclear/cytoplasmic fractionation experiment revealed that POT1-AS1 is preferentially localized in the cytoplasm (Figure 3A). Hence, it was speculated that POT1-AS1 might be a ceRNA. The directly interacting miRNAs of POT1-AS1 were then predicted using the publicly accessible database starBase 3.0, followed by selecting miR-497-5p for further evaluations, as it shares complementary binding sites with POT1-AS1 and suppresses tumors in GC, as depicted in Figure 3B (19). The binding between miR-497-5p and POT1-AS1 in GC cells was evaluated by LRA. In the presence of agomir-497-5p or agomir-NC, the transfection of WT-POT1-AS1 or MUT-POT1-AS1 was carried out into cells (HGC-27 and MKN-45). The miR-497-5p expression was considerably elevated in these cells post transfection with agomir-497-5p, as represented in Figure 3C. The obtained data indicated that the luciferase activity of WT-POT1-AS1 cells was considerably lowered (P<0.05), however, variations were not observed in the activity of MUT-POT1-AS1 with high expression of miR-497-5p (Figure 3D). The RIP assay was performed to investigate the link between miR-497-5p and POT1-AS1, and the data indicated that POT1-AS1 and miR-497-5p were enriched in immunoprecipitates (Ago2-containing) relative to the IgG control, as depicted in Figure 3E. We used RT-qPCR to evaluate the expression of miR-497-5p in 132 pairs of GC tissues and their surrounding healthy tissues to back up our findings. In comparison to surrounding healthy tissues, miR-497-5p was only modestly expressed in GC tissues, as depicted in Figure 3F. Furthermore, Spearman’s correlation analysis indicated an inverse expression association between POT1-AS1 and miR-497-5p in the 132 GC tissues (Figure 3G; Spearman r=0.443, P<0.001). The impact of POT1-AS1 on miR-497-5p expression was further evaluated in GC cells and the data revealed that miR-497-5p expression was considerably increased by POT1-AS1 silencing in HGC-27 and MKN-45 cells, as indicated in Figure 3H. According to these findings, POT1-AS1 may function as a molecular sponge for miR-497-5p in GC cells.
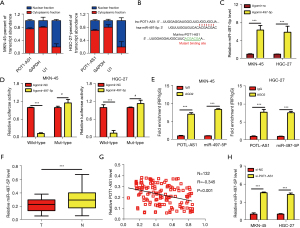
The impact of POT1-AS1 on PDK3 expression in GC cells by miR-497-5p sponging
In GC cells, PDK3 appears to be a direct target gene of miR-497-5p, according to a prior study (20). We investigated whether POT1-AS1 plays a role in the regulation of PDK3 in GC cells after discovering that it sponges miR-497-5p. To accomplish this, HGC-27 and MKN-45 cells were transfected with si-POT1-AS1 or si-NC, and PDK3 expression was examined. POT1-AS1 silencing significantly reduced PDK3 expression in HGC-27 and MKN-45 cells at both the transcriptional (Figure 4A) and translational (Figure 4B) levels. Using RT-qPCR, PDK3 expression was evaluated in 132 pairs of GC tissues as well as neighboring healthy tissues. In comparison to the nearby normal tissues, PDK3 expression was considerably elevated in GC tissues, as shown in Figure 4C. Furthermore, Spearman’s correlation analysis revealed a positive link between PDK3 mRNA expression and POT1-AS1 expression in the 132 GC tissues (Figure 4D; Spearman r=0.329, P=0.001). Si-POT1-AS1 plus antagomir-497-5p or antagomir-NC was transfected into MKN-45 and HGC-27 cells to observe whether POT1-AS1 influences PDK3 expression by sponging miR-497-5p. RT-qPCR was conducted to confirm the effectiveness of the antagomir-497-5p transfection. Antagomir-497-5p transfection led to a substantial reduction in miR-497-5p expression in MKN-45 and HGC-27 cells (Figure 4E). In MKN-45 and HGC-27 cells, antagomir-497-5p re-introduction reversed the downregulation of PDK3 at the transcriptional (Figure 4F) and translational level (Figure 4G, P=0.05) caused by POT1-AS1. These findings revealed that POT1-AS1 sponging miR-497-5p is used to favorably control PDK3 expression in GC cells.
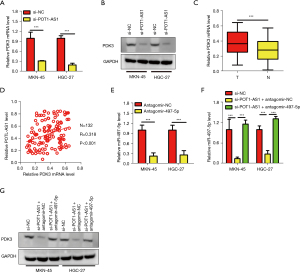
The effects of POT1-AS1 silencing in GC are partially rescued by attenuating miR-497-5p
Rescue experiments were conducted to identify the effect of the miR-497-5p/PDK3 axis on POT1-AS1 in GC cells. The proliferative, apoptotic, migratory, and invasive potential of MKN-45 and HGC-27 cells was evaluated post si-POT1-AS1 co-transfection with antagomir-497-5p or antagomir-NC. The proliferative, migratory, and invasive potential of MKN-45 and HGC-27 cells was decreased by POT1-AS1 silencing, as indicated in Figure 5A-5C, respectively. In these cells, however, suppression of miR-497-5p partially neutralized the impact of POT1-AS1 silencing.
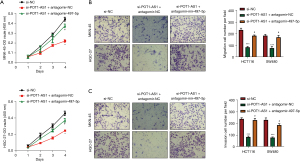
By restoring PDK3 expression, the impact of POT1-AS1 silencing on the progression of GC cells was reversed
Si-POT1-AS1 and pc-PDK3 or the empty pcDNA3.1 vectors were co-transfected into MKN-45 and HGC-27 cells. The transfection capacity of pc-PDK3 was determined using WB. Following pc-PDK3 transfection, PDK3 protein expression was considerably enhanced in MKN-45 and HGC-27 cells, as indicated in Figure 6A. Co-transfection of pc-PDK3 in MKN-45 and HGC-27 cells eliminated the decrease in the proliferative rate (Figure 6B) caused by POT1-AS1 silencing, as confirmed by the CCK-8 assay. Furthermore, suppressing POT1-AS1 elevated the rate of apoptosis in MKN-45 and HGC-27 cells, whereas elevated PDK3 expression reversed this impact (Figure 6B). The migration and invasion of MKN-45 and HGC-27 cells (Figure 6C,6D) were similar to the aforementioned results. Overall, these findings suggest that POT1-AS1 has a pro-oncogenic impact on GC development by acting as a ceRNA for miR-497-5p, thereby enhancing PDK3 expression.
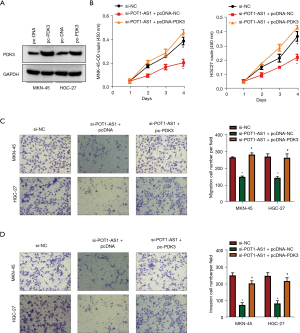
Discussion
Because of their critical roles in the progression of cancer and their significant clinical uses, lncRNAs have now generated great interest (20,21). Abnormal expression of lncRNAs in GC has been reported in several studies and plays a role in GC metastasis and progression (22). As a result, investigating the functions of lncRNAs in the carcinogenesis of GC may lead to the discovery of novel treatment targets for patients with GC. The goal of the present study was to reveal the expression of POT1-AS1 in GC and to determine its therapeutic relevance in patients suffering from GC. We extensively studied the involvement of POT1-AS1 in GC cell progression. We also identified the exact processes behind POT1-AS1’s pro-oncogenic involvement in GC development. POT1-AS1 was substantially expressed in GC cells according to our findings. In patients suffering from GC, elevated POT1-AS1 expression was associated with increased tumor volume, the presence of metastases in lymph nodes, and a better TNM stage, with P values of 0.001, 0.001, and 0.008, respectively. Furthermore, GC patients with elevated expression of POT1-AS1 exhibited lower OS and DFS compared with patients with decreased expression of POT1-AS1. The functional assays conducted in this work demonstrated that silencing POT1-AS1 inhibits cellular proliferation, colony formation, migration, and invasion.
Through multiple mechanisms, lncRNAs can contribute significantly to tumorigenesis and the progression of cancer. However, the most studied and dominant mechanism is the ceRNA regulatory mechanism, in which lncRNAs compete with miRNAs and consequently elevate the expression of specific miRNA target genes (23). We aimed to explore the pathways underlying POT1-AS ehanced GC cells migraion and invasion. Firstly, miR-497-5p has been definited to contain a complementary binding site (CBS) for POT1-AS1. RIP and the LRA were carried out for the validation of the interaction between miR-497-5p and POT1-AS1. Secondly, the expression of miR-497-5p was found to be comparatively lower in GC tissues and was inversely linked with the expression of LINC00577. Thirdly, decreased expression of POT1-AS1 enhanced the miR-497-5p level, which led to reduce a PDK3 expression level. Finally, restoring PDK3 or suppressing miR-497-5p could reverse the effects of POT1-AS1 suppression on GC oncogenic phenotypes. Our obtained results indicated a ceRNA model in GC cells that included POT1-AS1, miR-497-5p, and PDK3. In terms of the mechanism, the PDK family is comprised of 4 members, namely PDK1, PDK2, PDK3, and PDK4. The fact that these kinases are expressed differently in various tissues suggests that their physiological and pathological functions are tissue specific (23,24). For example, PDK4 is implicated in metabolic alterations due to fat-enriched diet and diabetes (24,25), but most studies have revealed that PDK1 and PDK3 play roles in cell survival and cellular metabolic switching when cells are exposed to hypoxia (26-28). In contrast, PDK3 is involved in carcinoma development. Elevated PDK3 expression is linked to poor prognosis in acute myelogenous leukemia (29). The apoptotic process of glioblastoma multiforme (30) was facilitated when PDK3 expression was downregulated. Furthermore, dichloroacetate inhibits PDK3 activity, which increases the anticancer effects of elesclomol (31) in melanoma. Taken together, these findings reveal that PDK3 can serve as a candidate target against the progression of carcinomas. Our obtained results revealed a unique upstream mechanism that controls the miR-497-5p/PDK3 interaction in GC cells through in vitro experiments. POT1-AS1 has an miR-497-5p interacting site which acts as a molecular ceRNA, elevating PDK3 expression via the increased level of miR-497-5p expression in GC cells. The discovery of the POT1-AS1/miR-497-5p/PDK3 regulation cascade may aid in furthering the understanding of GC pathophysiology and may provide potential treatment options for patients suffering from GC.
The following are some of the limitations of this study: (I) we did not evaluate onco-POT1-AS1 targets in GC cells, including miR-122-5p, miR-140-3p, or miR-552-3p. To fully understand the role of POT1-AS1 in GC, more research is needed in the future; (II) in this work, we used an arbitrary cut-off value for the POT1-AS1 expression level, and future research should focus on determining the best clinically relevant cut-off value; (III) furthermore, because our study sample size was small, more research is needed to demonstrate that POT1-AS1 is a useful biomarker for GC patients.
The present study discovered a unique method through which POT1-AS1 contributes to GC carcinogenesis. Silencing of POT1-AS1 inhibited the malignancy of GC cells. POT1-AS1 strongly regulated PDK3 expression in GC cells by sponging miR-497-5p, and the cancer-promoting effects of POT1-AS1 during GC progression were attributed to the validated ceRNA model. The POT1-AS1/miR-497-5p/PDK3 cascade could be a candidate target for GC treatment.
Acknowledgments
Funding: This study was supported by the National Key Basic Research Program of China (2014CB542102), National Human Genetic Resources Sharing Service Platform (2005DKA21300), Science Fund for Creative Research Groups, NSFC, China (81521091), State Key Infection Disease Project of China (2017ZX10203208), and Yangpu District science and Technology Commission on the project (YP18M05).
Footnote
Reporting Checklist: The authors have completed the MDAR reporting checklist. Available at https://dx.doi.org/10.21037/jgo-21-709
Data Sharing Statement: Available at https://dx.doi.org/10.21037/jgo-21-709
Conflicts of Interest: All authors have completed the ICMJE uniform disclosure form (Available at https://dx.doi.org/10.21037/jgo-21-709). The authors have no conflicts of interest to declare.
Ethical Statement: The authors are accountable for all aspects of the work in ensuring that questions related to the accuracy or integrity of any part of the work are appropriately investigated and resolved. Approval for this study was obtained from Changhai Hospital’s research ethics committee (No. 16ZR1400800). Each experiment was performed in accordance with the Declaration of Helsinki (as revised in 2013). All patients provided informed consent.
Open Access Statement: This is an Open Access article distributed in accordance with the Creative Commons Attribution-NonCommercial-NoDerivs 4.0 International License (CC BY-NC-ND 4.0), which permits the non-commercial replication and distribution of the article with the strict proviso that no changes or edits are made and the original work is properly cited (including links to both the formal publication through the relevant DOI and the license). See: https://creativecommons.org/licenses/by-nc-nd/4.0/.
References
- McGuire S. World Cancer Report 2014. Geneva, Switzerland: World Health Organization, International Agency for Research on Cancer, WHO Press, 2015. Adv Nutr 2016;7:418-9. [Crossref] [PubMed]
- Van Cutsem E, Sagaert X, Topal B, et al. Gastric cancer. Lancet 2016;388:2654-64. [Crossref] [PubMed]
- Yan F, Ying L, Li X, et al. Overexpression of the transcription factor ATF3 with a regulatory molecular signature associates with the pathogenic development of colorectal cancer. Oncotarget 2017;8:47020-36. [Crossref] [PubMed]
- Chi Y, Wang D, Wang J, et al. Long Non-Coding RNA in the Pathogenesis of Cancers. Cells 2019;8:1015. [Crossref] [PubMed]
- Lv Y, Huang S. Role of non-coding RNA in pancreatic cancer. Oncol Lett 2019;18:3963-73. [Crossref] [PubMed]
- Penna GC, Vaisman F, Vaisman M, et al. Molecular Markers Involved in Tumorigenesis of Thyroid Carcinoma: Focus on Aggressive Histotypes. Cytogenet Genome Res 2016;150:194-207. [Crossref] [PubMed]
- Jiang MC, Ni JJ, Cui WY, et al. Emerging roles of lncRNA in cancer and therapeutic opportunities. Am J Cancer Res 2019;9:1354-66. [PubMed]
- Batista PJ, Chang HY. Long noncoding RNAs: cellular address codes in development and disease. Cell 2013;152:1298-307. [Crossref] [PubMed]
- Fernandes JCR, Acuña SM, Aoki JI, et al. Long Non-Coding RNAs in the Regulation of Gene Expression: Physiology and Disease. Noncoding RNA 2019;5:17. [Crossref] [PubMed]
- Ponting CP, Oliver PL, Reik W. Evolution and functions of long noncoding RNAs. Cell 2009;136:629-41. [Crossref] [PubMed]
- Hauptman N, Glavač D. Long non-coding RNA in cancer. Int J Mol Sci 2013;14:4655-69. [Crossref] [PubMed]
- Lu XC, Zhou HY, Wu J, et al. LncRNA LINP1 promotes proliferation and inhibits apoptosis of gastric cancer cells by repressing RBM5. Eur Rev Med Pharmacol Sci 2020;24:137-44. [PubMed]
- Tao Y, Wan X, Fan Q, et al. Long non-coding RNA OIP5-AS1 promotes the growth of gastric cancer through the miR-367-3p/HMGA2 axis. Dig Liver Dis 2020;52:773-9. [Crossref] [PubMed]
- Wu XY, Zhou HY, Yao XM, et al. Long non-coding RNA AB073614 promotes metastasis of gastric cancer cells by upregulating IGF-2. Eur Rev Med Pharmacol Sci 2020;24:145-150. [PubMed]
- Chen ZB, Cao WL, Su K, et al. MIR22HG inhibits cell growth, migration and invasion through regulating the miR-24-3p/p27kip1 axis in thyroid papillary carcinomas. Eur Rev Med Pharmacol Sci 2019;23:5851-62. [PubMed]
- Wu DM, Wang S, Wen X, et al. LncRNA SNHG15 acts as a ceRNA to regulate YAP1-Hippo signaling pathway by sponging miR-200a-3p in papillary thyroid carcinoma. Cell Death Dis 2018;9:947. [Crossref] [PubMed]
- Xia F, Chen Y, Jiang B, et al. Long Noncoding RNA HOXA-AS2 Promotes Papillary Thyroid Cancer Progression by Regulating miR-520c-3p/S100A4 Pathway. Cell Physiol Biochem 2018;50:1659-72. [Crossref] [PubMed]
- Ma YN, Hong YG, Yu GY, et al. LncRNA LBX2-AS1 promotes colorectal cancer progression and 5-fluorouracil resistance. Cancer Cell Int 2021;21:501. [Crossref] [PubMed]
- Feng L, Cheng K, Zang R, et al. miR-497-5p inhibits gastric cancer cell proliferation and growth through targeting PDK3. Biosci Rep 2019;39:BSR20190654. [Crossref] [PubMed]
- Chu F, Xue L, Miao H. Long noncoding RNA TP73-AS1 in human cancers. Clin Chim Acta 2020;500:104-8. [Crossref] [PubMed]
- Gao C, Wu X, Zhai J, et al. Long non-coding RNA SNHG17 promotes gastric cancer progression by inhibiting P15 and P16. Transl Cancer Res 2019;8:520-31. [Crossref]
- Lin MT, Song HJ, Ding XY. Long non-coding RNAs involved in metastasis of gastric cancer. World J Gastroenterol 2018;24:3724-37. [Crossref] [PubMed]
- Yin C, Mou Q, Pan X, et al. MiR-577 suppresses epithelial-mesenchymal transition and metastasis of breast cancer by targeting Rab25. Thorac Cancer 2018;9:472-9. [Crossref] [PubMed]
- Holness MJ, Kraus A, Harris RA, et al. Targeted upregulation of pyruvate dehydrogenase kinase (PDK)-4 in slow-twitch skeletal muscle underlies the stable modification of the regulatory characteristics of PDK induced by high-fat feeding. Diabetes 2000;49:775-81. [Crossref] [PubMed]
- Wu P, Inskeep K, Bowker-Kinley MM, et al. Mechanism responsible for inactivation of skeletal muscle pyruvate dehydrogenase complex in starvation and diabetes. Diabetes 1999;48:1593-9. [Crossref] [PubMed]
- Kim JW, Tchernyshyov I, Semenza GL, et al. HIF-1-mediated expression of pyruvate dehydrogenase kinase: a metabolic switch required for cellular adaptation to hypoxia. Cell Metab 2006;3:177-85. [Crossref] [PubMed]
- Lu CW, Lin SC, Chen KF, et al. Induction of pyruvate dehydrogenase kinase-3 by hypoxia-inducible factor-1 promotes metabolic switch and drug resistance. J Biol Chem 2008;283:28106-14. [Crossref] [PubMed]
- Papandreou I, Cairns RA, Fontana L, et al. HIF-1 mediates adaptation to hypoxia by actively downregulating mitochondrial oxygen consumption. Cell Metab 2006;3:187-97. [Crossref] [PubMed]
- Cui L, Cheng Z, Liu Y, et al. Overexpression of PDK2 and PDK3 reflects poor prognosis in acute myeloid leukemia. Cancer Gene Ther 2020;27:15-21. [Crossref] [PubMed]
- Han JE, Lim PW, Na CM, et al. Inhibition of HIF1α and PDK Induces Cell Death of Glioblastoma Multiforme. Exp Neurobiol 2017;26:295-306. [Crossref] [PubMed]
- Kluza J, Corazao-Rozas P, Touil Y, et al. Inactivation of the HIF-1α/PDK3 signaling axis drives melanoma toward mitochondrial oxidative metabolism and potentiates the therapeutic activity of pro-oxidants. Cancer Res 2012;72:5035-47. [Crossref] [PubMed]