Serum N-glycan profiling as a diagnostic biomarker for the identification of hepatitis B virus-associated hepatocellular carcinoma
Introduction
Hepatocellular carcinoma (HCC) is one of the most common malignant tumors, which seriously threatens human health and life (1). HCC often occurs in the context of chronic liver disease, and liver cirrhosis (LC) is the strongest risk factor for HCC (2), including hepatitis B virus (HBV) or hepatitis C virus (HCV) infection, alcohol, diabetes, or obesity-related nonalcoholic steatohepatitis (NASH). However, HBV infection is the most prominent risk factor for the development of HCC, accounting for about 50% of cases (3). The symptoms of HCC in the early stage are not obvious, and HCC is usually diagnosed at the late stage. As a result, many patients miss the best treatment window, which may worsen the disease. Given that HCC poses a serious threat to human life, there is an urgent need for early screening and diagnosis methods.
Currently, alpha-fetoprotein (AFP) is still the most widely used biomarker in the diagnosis and evaluation of HCC. Clinically, AFP is often combined with imaging methods such as ultrasound for the early diagnosis of HCC (4). However, AFP as a screening and diagnostic test for early HCC is controversial. Multiple studies have shown that the sensitivity and specificity of AFP in the diagnosis of HCC are not satisfactory, with a sensitivity of 39–64% and a specificity of 76–91% (5,6). When the AFP cutoff values are 20 and 200 ng/mL, its diagnostic sensitivity are 60% and 22% respectively (7). Studies have found that AFP levels are related to tumor size, while 80% of patients with small liver cancer have low AFP levels. When the diameter of liver cancer >3 and <3 cm, the diagnostic sensitivity of AFP are 52% and 25% respectively (8). Moreover, a considerable number of patients with chronic hepatitis and cirrhosis also have elevated AFP levels, usually <400 ng/mL. If AFP >400 ng/mL, it strongly suggests HCC (8). It is worth noting that 30–40% of HCC patients have a negative serum AFP level until the advanced stage (9,10). Therefore, a serum biomarker with high sensitivity and specificity is urgently needed for the early diagnosis of HCC.
Recently, more and more evidence has confirmed that N-glycan can be used as a reliable biomarker for disease diagnosis and prognosis (11). N-glycan is one of the most common glycosylation modifications of proteins. Proteins are often modified by glycosylation in disease states (12). Changes in protein glycosylation can reflect the patient’s inflammatory state, and can serve not only as a disease sensor, but also as a marker for tumor progression and prognosis (13). It has been reported that glycosylation can occur in the serum proteins of patients with liver disease (14). However, human serum glycoprotein is mainly produced by the liver (13). Therefore, abnormalities in the quantity and structure of N-glycan often reflect liver lesions and can be reflected in the serum. However, few studies have focused solely on the N-glycan profile in patients with HBV-related liver disease. In this study, we used the DNA sequencer-assisted fluorophore-assisted carbohydrate electrophoresis (DSA-FACE) technique to analyze the characteristic changes of serum N-glycan in patients with HBV relative liver disease and healthy subjects. Moreover, we evaluated the role of specific N-glycan markers in the diagnosis of HCC, which is expected to facilitate diagnosis and corresponding treatment. We present the following article in accordance with the STARD reporting checklist (available at https://jgo.amegroups.com/article/view/10.21037/jgo-22-93/rc).
Methods
Inclusion and exclusion criteria
This study was approved by the Ethics Committee of Nanchang University Second Affiliated Hospital (No. 2019036#) and conducted from October 2019 to February 2020. All procedures performed in this study involving human participants were in accordance with the Declaration of Helsinki (as revised in 2013). A total of 81 individuals were selected for this retrospective study, including chronic hepatitis B (CHB) (n=20), LC (n=20), HCC (n=21), and age-matched healthy controls (n=20). All individuals provided written informed consent. Patients with chronic HBV infection who were treated as outpatients of the Department of Gastroenterology and the Department of Infection of the hospital were included in the study. The inclusion criteria were over 18 years old, non-pregnant women, and no previous history of treatment for hepatitis B. Patients with acute HBV infection, or with HCV or human immunodeficiency virus (HIV) co-infection, and those with long-term alcohol consumption were excluded from the study. Chronic HBV infection was defined as HBsAg positive lasting for more than 6 months, hepatitis B e antigen (HBeAg) (+/−), and alanine aminotransferase (ALT) or aspartate aminotransferase (AST) increasing continuously or intermittently. Cirrhosis was confirmed by ultrasound with associated hypoproteinemia and prolonged prothrombin time. HCC was diagnosed by ultrasound or computed tomography (CT) scans of the liver for a mass and AFP ≥400 ng/mL (15). Liver biopsy was not performed in the evaluation of hepatitis as well as in the diagnosis of patients with a hepatic mass.
Sample collection
Blood samples were collected from outpatients/inpatients and from healthy controls. After the blood samples were centrifuged, the upper serum was separated into eppendorf tubes and placed in a −20 °C refrigerator for further examination.
Serum clinical laboratory tests
The main clinical characteristics of patients with liver disease and healthy subjects are shown in Table 1. All patients with HBV infection were diagnosed by serological tests: hepatitis B surface antigen (HBsAg), hepatitis B surface antibody (HBsAb), hepatitis B e antigen (HBeAg), hepatitis B e antibody (HBeAb), hepatitis B core antibody (HBcAb), and hepatitis B virus (HBV) DNA. Aspartate aminotransferase (AST), alanine aminotransferase (ALT), gamma glutamyl transpeptidase (GGT), albumin (ALB), and total bilirubin (TBIL) were used to determine the degree of liver injury.
Table 1
Clinical parameters | Control | CHB | LC | HCC | P value |
---|---|---|---|---|---|
n (M/F) | 20 (8/12) | 20 (11/9) | 20 (10/10) | 21 (12/9) | 0.149 |
Age (years) | 47.0 (39.2–54.2) | 47.0 (39.2–54.2) | 49.5 (44.0–55.0) | 59.0 (52.0–65.0) | 0.054 |
AST (U/L) | 18.9 (17.8–21.6) | 39.4 (30.5–74.9) | 55.4 (39.6–76.9) | 51.7 (37.1–84.6) | 0.000 |
ALT (U/L) | 16.9 (11.3–24.3) | 48.9 (24.3–113.2) | 42.4 (25.9–57.1) | 42.5 (32.0–69.9) | 0.000 |
GGT (U/L) | 19.8 (14.0–28.1) | 29.2 (17.2–61.9) | 43.9 (28.2–96.0) | 75.9 (34.6–130.4) | 0.000 |
ALB (g/L) | 41.7 (40.1–44.0) | 45.2 (40.2–48.2) | 36.2 (31.7–41.2) | 33.5 (30.9–38.2) | 0.000 |
TBIL (μmol/L) | 11.9 (9.2–14.0) | 14.1 (11.2–17.9) | 22.5 (15.2–39.0) | 18.1 (14.7–21.0) | 0.000 |
Data were median (25th–75th percentile) values as indicated. Significance was defined as P<0.05. HBV, hepatitis B virus; M, male; F, female; AST, aspartate aminotransferase; ALT, alanine aminotransferase; GGT, gamma glutamyl transpeptidase; ALB, albumin; TBIL, total bilirubin; CHB, chronic hepatitis B; LC, liver cirrhosis; HCC, hepatocellular carcinoma.
Serum N-glycan and AFP analysis
The N-glycan structure abundances were measured by DSA-FACE technology with a capillary electrophoresis-based ABI3500dx sequencer. As previously reported, we released, labeled, and analyzed N-glycan on proteins in 2 µL serum (16). The labeled N-glycan was analyzed by GeneMapper version 3.7 software (Applied Biosystems, Foster City, CA, USA). The level of AFP in the serum samples was determined by electrochemiluminescence using the Cobas 8000 e602 Immunology Analyzer (Roche, Bassel City, Switzerland). The experimental operation was carried out by professionals in strict accordance with the standard operating procedures, and testing was repeated.
Statistical analysis
In this study, SPSS25.0 (SPSS Inc., Chicago, IL, USA) was used to carry out statistical analysis on the experimental data. GraphPad Prism 7.0 was used to draw the figures in this study. Categorical variables were expressed as percentage and compared by the χ2 test or Fisher’s exact test. Continuous variables were represented by median (quartile range) and compared by the Mann-Whitney U test. The diagnostic value of N-glycan and AFP was evaluated by receiver operating characteristic (ROC) curves. The optimal cut-off value of biomarker was determined by receiver operating curve (the maximum value of Jorden index was the optimal cut-off value). Pearson correlation coefficient was used to analyze the correlations between N-glycan and laboratory indexes. P<0.05 were considered to be statistically significant.
Results
Baseline characteristics
Data were obtained from 81 (41 men, 40 women) participants. The general characteristics of the 3 hepatic disease groups and the controls are summarized in Table 1. In terms of age and sex, there were no significant differences among the groups. Statistically significant differences were observed in ALT, AST, GGT, ALB, and TBIL levels between patients with liver disease and healthy subjects (P<0.05).
Discovery of abnormal N-glycan structures related to patients with liver disease
DSA-FACE technology was used to detect the serum N-glycan profiles of 61 patients with liver disease (CHB: 20; LC: 20; HCC: 21) and 20 healthy subjects. The structure analysis corresponding to each N-glycan peak has been explained in a previous study (17). The relative concentrations of the 9 N-glycan peaks are shown in Table 2. The results showed that the differences in the abundance of peaks 7, 8, and 9 between the liver disease patient groups and healthy subjects were statistically significant (P<0.05) (Table 2). Moreover, our study found that peak 7 (NA2FB) in LC patients was significantly higher than that in the CHB, HCC, and control groups (P<0.05 for all) (Figure 1A). The abundance of peak 8 (NA3) in the CHB and LC groups was decreased than that in the control group (P<0.05 for both). Although there was no difference between the HCC and control groups, the same trend was observed (Figure 1B). Interestingly, peak 9 (NA3Fb) increased significantly in the HCC group (P<0.05 for all), but there were no significant differences among the other groups (Figure 1C). In addition, in order to realize the diagnosis of HCC in the context of LC, we focused on identifying glycan structures that only increased in HCC but not in cirrhosis. We found that the Log (peak 9/peak 7) of HCC was higher than that of LC (P<0.001) (Figure 1D). Therefore, the index Log (peak 9/peak 7) might be used as a specific diagnostic marker for HCC with LC. Moreover, we also found that AFP levels in the HCC group were significantly elevated compared to those in the CHB, LC, and control groups (P<0.001) (Figure 1E). These data suggested that NA3Fb and AFP were closely related to the occurrence and development of HCC, and NA3Fb may be a specific biomarker for the diagnosis of HCC.
Table 2
Log peak | Control | CHB | LC | HCC | P value |
---|---|---|---|---|---|
Peak 1 | 2.64 (2.51–2.81) | 2.52 (2.46–2.66) | 2.74 (2.49–3.00) | 2.81 (2.41–2.90) | 0.101 |
Peak 2 | 1.99 (1.77–2.10) | 1.89 (1.84–1.98) | 2.09 (1.90–2.22) | 2.08 (1.84–2.24) | 0.106 |
Peak 3 | 2.67 (2.43–2.74) | 2.49 (2.42–2.58) | 2.61 (2.43–2.89) | 2.68 (2.36–2.83) | 0.238 |
Peak 4 | 2.54 (2.48–2.69) | 2.50 (2.46–2.53) | 2.52 (2.34–2.76) | 2.56 (2.38–2.85) | 0.481 |
Peak 5 | 3.82 (3.66–3.88) | 3.70 (3.63–3.79) | 3.62 (3.44–3.84) | 3.77 (3.55–4.01) | 0.191 |
Peak 6 | 3.27 (3.00–3.43) | 3.08 (2.98–3.18) | 3.13 (2.97–3.41) | 3.17 (2.89–3.47) | 0.293 |
Peak 7 | 2.54 (2.41–2.79) | 2.54 (2.41–2.60) | 2.72 (2.60–2.96) | 2.63 (2.43–2.88) | 0.002 |
Peak 8 | 3.13 (2.97–3.27) | 2.99 (2.94–3.05) | 2.80 (2.39–3.07) | 3.05 (2.60–3.15) | 0.033 |
Peak 9 | 2.48 (2.26–2.58) | 2.46 (2.26–2.77) | 2.46 (2.31–2.76) | 2.81 (2.66–2.96) | 0.000 |
Data were median (25th–75th percentile) values as indicated. Significance was defined as P<0.05. Peak 1 indicates NGA2F, peak 2 indicates NGA2FB, peaks 3 and 4 indicate NG1A2F, peak 5 indicates NA2, peak 6 indicates NA2F, peak 7 indicates NA2FB, peak 8 indicates NA3, and peak 9 indicates NA3Fb. HBV, hepatitis B virus; CHB, chronic hepatitis B; LC, liver cirrhosis; HCC, hepatocellular carcinoma.
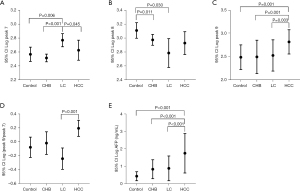
N-glycan biomarkers in different stages of HCC
To evaluate the relationship between the HCC glycomic markers and tumor stage, the HCC subgroup (n=21) with defined tumor sizes and stages was analyzed for glycomic changes. According to the Barcelona clinic liver cancer (BCLC) criteria, patients with HCC were classified into BCLC A (n=7), BCLC B/C (n=9), and BCLC D groups (n=5). The comparative analysis of N-glycan in the three subgroups is shown in Table 3. The abundance of peak 9 (NA3Fb) in the BCLC D stage group was significantly higher than that in the BCLC A and B/C stage groups (P=0.018, P=0.012, respectively) (Figure 2). The abundance of NA3Fb increased with the progression of the disease.
Table 3
Log peak | BCLC A (n=7) | BCLC B/C (n=9) | BCLC D (n=5) | P value |
---|---|---|---|---|
Peak 1 | 2.73 (2.35–2.92) | 2.68 (2.42–2.90) | 2.86 (2.61–3.18) | 0.562 |
Peak 2 | 1.92 (1.75–2.24) | 2.05 (1.84–2.23) | 2.23 (1.84–2.43) | 0.705 |
Peak 3 | 2.77 (2.26–2.84) | 2.68 (2.41–2.73) | 2.82 (2.46–3.04) | 0.612 |
Peak 4 | 2.55 (2.28–2.81) | 2.59 (2.42–2.86) | 2.57 (2.42–3.01) | 0.520 |
Peak 5 | 3.76 (3.31–3.91) | 3.73 (3.55–3.98) | 3.99 (3.78–4.26) | 0.127 |
Peak 6 | 3.07 (2.77–3.55) | 3.15 (2.96–3.15) | 3.46 (3.06–3.59) | 0.499 |
Peak 7 | 2.44 (2.06–2.95) | 2.63 (2.49–2.83) | 2.81 (2.57–3.04) | 0.346 |
Peak 8 | 2.99 (2.37–3.11) | 2.95 (2.60–3.14) | 3.13 (2.90–3.45) | 0.228 |
Peak 9 | 2.75 (2.60–2.86) | 2.73 (2.62–2.90) | 2.97 (2.95–3.24) | 0.027 |
Data were median (25th–75th percentile) values as indicated. Significance was defined as P<0.05. n, case number; HCC, hepatocellular carcinoma; BCLC, Barcelona clinic liver cancer.
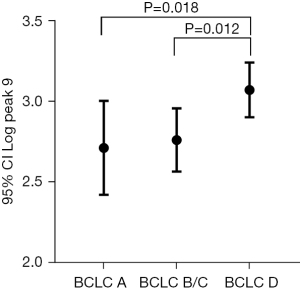
Correlation between N-glycan markers and laboratory indexes
Common laboratory indicators included age, AST, ALT, GGT, ALB, TBIL, and AFP, and the correlation analysis with N-glycan markers (peak 7, 8, 9) is shown in Table 4. Our results showed that peak 7 was positively correlated with AST (R=0.239, P=0.032), but was negatively correlated with ALB (R=−0.363, P=0.001). There was no correlation between peak 8 and other laboratory indexes. Peak 9 was positively correlated with AST, ALT, GGT, and AFP (R=0.297, P=0.007; R=0.245, P=0.027; R=0.336, P=0.002; R=0.338, P=0.002), but was negatively correlated with ALB (R=−0.337, P=0.002). Our results suggested that serum N-glycan levels were affected by multiple factors in vivo.
Table 4
Correlations | Peak 7 | Peak 8 | Peak 9 |
---|---|---|---|
Age (years) | |||
R | −0.172 | −0.156 | 0.069 |
P | 0.126 | 0.164 | 0.543 |
AST (U/L) | |||
R | 0.239 | −0.094 | 0.297 |
P | 0.032* | 0.406 | 0.007* |
ALT (U/L) | |||
R | 0.149 | −0.02 | 0.245 |
P | 0.184 | 0.862 | 0.027* |
GGT (U/L) | |||
R | 0.170 | −0.065 | 0.336 |
P | 0.129 | 0.563 | 0.002* |
ALB (g/L) | |||
R | −0.363 | 0.145 | −0.337 |
P | 0.001* | 0.185 | 0.002* |
TBIL (μmol/L) | |||
R | 0.167 | −0.097 | 0.040 |
P | 0.135 | 0.387 | 0.720 |
AFP (ng/mL) | |||
R | 0.100 | 0.016 | 0.338 |
P | 0.373 | 0.886 | 0.002* |
*, represents significant correlation between laboratory indexes and corresponding N-glycan biomarkers. AST, aspartate aminotransferase; ALT, alanine aminotransferase; GGT, gamma glutamyl transpeptidase; ALB, albumin; TBIL, total bilirubin; AFP, alpha-fetoprotein.
Diagnostic value of N-glycan markers and AFP in HCC
In view of the slight increase in the level of AFP in patients with liver disease, there are limitations of AFP in screening for HCC. Our study found that the sensitivity for HCC diagnosis was lower when AFP was at a low threshold. Table 5 shows the diagnostic sensitivity and specificity data for different AFP cutoff values. The sensitivities of Log peak 9 (cutoff =2.59) and log (peak 9/peak 7) (cutoff =0.03) to HCC were 90.5% and 85.7% respectively, which were higher than those of AFP with different cutoff values (33.3–57.1%). The total specificity of both was similar to that of AFP.
Table 5
Cutoff values | HCC (n) | False positive (n) | Sensitivity (%) | Specificity (%) |
---|---|---|---|---|
Log peak 9 cutoff of 2.59 | 19 | 15 | 90.5 | 75.0 |
Log (peak 9/peak 7) cutoff of 0.03 | 18 | 13 | 85.7 | 78.3 |
AFP cutoff 10 ng/mL | 12 | 13 | 57.1 | 78.3 |
AFP cutoff of 20 ng/mL | 11 | 7 | 52.4 | 88.3 |
AFP cutoff of 100 ng/mL | 11 | 2 | 52.4 | 96.7 |
AFP cutoff of 200 ng/mL | 9 | 2 | 42.9 | 96.7 |
AFP cutoff of 400 ng/mL | 7 | 1 | 33.3 | 98.3 |
n, case number; HCC, hepatocellular carcinoma; AFP, alpha-fetoprotein.
Moreover, in order to further understand the effect of N-glycan markers in the diagnosis of HCC, we analyzed the ROC curves of Log peak 9, Log (peak 9/peak 7) alone, and combined with AFP in the diagnosis of HCC (Figure 3A). The ROC curve showed that the accuracy of Log peak 9 [area under the ROC curve (AUC): 0.81±0.05] was better than that of Log (peak 9/peak 7) (AUC: 0.79±0.05) and AFP (AUC: 0.76±0.07), while the accuracy of AFP combined with above 2 indexes was better than that of the single index. Moreover, Log (peak 9/peak 7) combined with AFP was similar to Log peak 9 combined with AFP (AUC: 0.86±0.05), and both had better accuracy in the diagnosis of HCC.
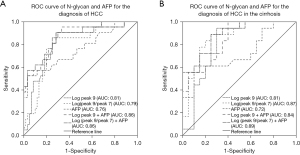
Diagnostic value of N-glycan markers and AFP in HCC with cirrhosis
In order to further realize the diagnosis of HCC in the context of LC, we excluded 3 HCC patients without LC. Low sensitivity to HCC diagnosis was also found in patients with LC when the AFP threshold was low, as shown in Table 6, which displays the data for different AFP cutoff values. The sensitivities of Log peak 9 (cutoff =2.59) and Log (peak 9/peak 7) (cutoff =0.03) in the diagnosis of HCC were 94.4% and 88.9%, respectively, which were higher than those of AFP with different cutoff values (33.3–61.1%). The total specificity of both was similar to that of AFP.
Table 6
Cutoff values | HCC (n) | False positive (n) | Sensitivity (%) | Specificity (%) |
---|---|---|---|---|
Log peak 9 cutoff of 2.59 | 17 | 6 | 94.4 | 70.0 |
Log (peak 9/peak 7) cutoff of 0.03 | 16 | 5 | 88.9 | 75.0 |
AFP cutoff of 10 ng/mL | 11 | 7 | 61.1 | 65.0 |
AFP cutoff of 20 ng/mL | 10 | 3 | 55.6 | 85.0 |
AFP cutoff of 100 ng/mL | 10 | 2 | 55.6 | 90.0 |
AFP cutoff of 200 ng/mL | 8 | 2 | 44.4 | 90.0 |
AFP cutoff of 400 ng/mL | 6 | 1 | 33.3 | 95.0 |
n, case number; HCC, hepatocellular carcinoma; AFP, alpha-fetoprotein.
In order to further understand the effect of N-glycan markers in diagnosing HCC in patients with LC, we used Log peak 9 and Log (peak 9/peak 7) alone or in combination with AFP to detect HCC in patients with cirrhosis. The ROC curve of HCC in patients with LC is shown in Figure 3B, and its diagnostic value was compared in Table 6. The AUCs showed that Log (peak 9/peak 7) (AUC: 0.87±0.06) was better than Log peak 9 (AUC: 0.81±0.07) and AFP (AUC: 0.72±0.09), Log peak 9 and Log (peak 9/peak 7) combined with AFP was better than a single index, while Log (peak 9/peak 7) combined with AFP (AUC: 0.89±0.06) was better than Log peak 9 combined with AFP (AUC: 0.84±0.06).
Discussion
Abnormal glycosylation caused by inflammation or malignant transformation is related to liver disease and plays an important role in the transformation and metastasis of malignant tumors (13). Abnormal glycosylation can interfere with the regulation of cell adhesion, migration, and proliferation, which may be a sign of cancer, reflecting the changes in cancer-specific glycosylation biosynthetic pathways (18). As glycosylation pathways in inflammatory cells or cancer cells are often altered, the discovery of new serum N-glycan biomarkers has attracted increased attention.
As an N-glycan spectrum detection technique, DSA-FACE has the advantages of high sensitivity, high throughput, high speed, simple operation, and high efficiency. It has been widely used in the detection of N-glycan in cancer and other diseases (19,20). In this study, we used this technique to analyze the serum N-glycan of patients with liver diseases and healthy subjects. The study showed that there were differences in the abundance of NA2FB, NA3, and NA3Fb among patients with liver disease and healthy people. The abundance of NA2FB was the highest in the LC group, while NA3Fb was the most abundant in the HCC group. Consistent with a previous study, NA2FB and NA3Fb have been reported to be elevated in patients with LC and HCC, respectively (17). N-acetylglucose aminotransferase III (GnT-III) is responsible for forming the “bisecting” GlcNAc structure (such as NA2FB), while GnT IV and GnT V (GnT-V) catalyze β1-6-GlcNAc branching (such as NA3Fb) (21). GnT-III usually serves as a tumor suppressor. Overexpression of GnT-III inhibits cancer cells in vivo and in vitro (22). However, GnT-V is a known cancer-promoting glycosyltransferase whose expression is significantly up-regulated in liver cancer cells (23,24). Several studies have shown that GnT-III can inhibit cancer metastasis, while GnT-V contributes to metastasis (23,25). Moreover, NA2FB competes with NA3Fb for substrate (NA2F), which leads to a decrease in peak 7 and an increase in peak 9 during tumor development (17).
Abnormal laboratory indicators often reflect protein instability and inflammation in patients with liver disease. We performed Pearson correlation analysis of N-glycan and laboratory indicators and found that NA3FB and NA3Fb were negatively correlated with ALB. ALB is synthesized and secreted by the liver (10–15 g/d), and the decrease in ALB level may reflect impaired liver function. This indicates that high levels of NA2FB and NA3Fb may be related to liver damage. Moreover, this study also found that elevated NA3Fb levels were positively correlated with AST, ALT, and GGT. AST and ALT are not only sensitive indicators of liver damage, but also sensitive indicators of the progression of viral hepatitis (26). GGT, known as cholestatic liver enzyme, is elevated in obesity, alcoholism, and fatty liver, and after taking certain drugs or herbs. GGT also showed good sensitivity when viral hepatitis progressed (27). Therefore, it cannot be excluded that the high level of NA3Fb present in patients with HCC was not associated with hepatocyte damage and cholestasis. Interestingly, our study also suggested that NA3Fb (peak 9) abundance was related to tumor BCLC staging, especially the highest NA3Fb level in BCLC D stage, which may be due to changes in the liver cell glycosylation mechanism during malignant transformation. The level of NA3Fb in HCC patients gradually increased with the progression of the disease. The mechanism may be due to the increase of NA3Fb on the surface of liver cancer cells caused by the high expression of GnT-IVa, which can promote cancer progression (28).
The changes of N-glycan are closely related to the occurrence and development of LC and HCC (29). The combination of new serum N-glycan markers and other existing serological markers are valuable in the diagnosis of liver diseases (17). We used Log peak 9 and Log (peak 9/peak 7) alone or in combination with AFP to diagnose HCC in this study. For HCC screening in patients, especially in patients with LC, Log peak 9 and Log (peak 9/peak 7) were more sensitive than AFP, and their specificity was similar to that of AFP. The ROC curve showed that the accuracy of Log peak 9 and Log (peak 9/peak 7) was better than that of AFP, while the accuracy of AFP combined with the above 2 indexes was better than that of a single index. Moreover, Log (peak 9/peak 7) combined with AFP had the best accuracy in the diagnosis of HCC. Due to the difficulty of ultrasound imaging in diagnosing HCC in patients with LC, serum glycan markers can be used as a valuable supplement to AFP in the diagnosis of HCC (30). The main limitation of this study is the small sample size. In the future, we will expand the sample size of patients to further verify whether Log peak 9 and Log (peak 9/peak 7) can be effectively used in the clinical diagnosis of HCC.
Conclusions
In summary, we analyzed the specific N-glycan profiles in the serum of patients with liver disease using DSA-FACE. Our experimental data suggested that the abundances of NA2FB and NA3Fb in serum were significantly elevated in patients with liver disease compared with healthy subjects, while NA3 abundance was obviously decreased. Moreover, Log peak 9 and Log (peak 9/peak 7) may be used as serum biomarkers for the diagnosis of HCC, especially Log (peak 9/peak 7), which was better in the diagnosis of HCC in patients with LC. Therefore, serum N-glycan profiles may be helpful for the early diagnosis of HCC, and complementation with AFP can improve the detection of HCC.
Acknowledgments
Funding: This work was supported by the National Natural Science Foundation of China (Nos. 81960440 and 82070594).
Footnote
Reporting Checklist: The authors have completed the STARD reporting checklist. Available at https://jgo.amegroups.com/article/view/10.21037/jgo-22-93/rc
Data Sharing Statement: Available at https://jgo.amegroups.com/article/view/10.21037/jgo-22-93/dss
Conflicts of Interest: All authors have completed the ICMJE uniform disclosure form (available at https://jgo.amegroups.com/article/view/10.21037/jgo-22-93/coif). All authors report this work was supported by the National Natural Science Foundation of China (Nos. 81960440 and 82070594). The authors have no other conflicts of interest to declare.
Ethical Statement: The authors are accountable for all aspects of the work in ensuring that questions related to the accuracy or integrity of any part of the work are appropriately investigated and resolved. All procedures performed in this study involving human participants were in accordance with the Declaration of Helsinki (as revised in 2013). The study was approved by the Ethics Committee of Nanchang University Second Affiliated Hospital (No. 2019036#) and informed consent was taken from all the individuals.
Open Access Statement: This is an Open Access article distributed in accordance with the Creative Commons Attribution-NonCommercial-NoDerivs 4.0 International License (CC BY-NC-ND 4.0), which permits the non-commercial replication and distribution of the article with the strict proviso that no changes or edits are made and the original work is properly cited (including links to both the formal publication through the relevant DOI and the license). See: https://creativecommons.org/licenses/by-nc-nd/4.0/.
References
- Bray F, Ferlay J, Soerjomataram I, et al. Global cancer statistics 2018: GLOBOCAN estimates of incidence and mortality worldwide for 36 cancers in 185 countries. CA Cancer J Clin 2018;68:394-424. [Crossref] [PubMed]
- European Association for the Study of the Liver. Electronic address: easloffice@easloffice.eu; European Association for the Study of the Liver. EASL Clinical Practice Guidelines: Management of hepatocellular carcinoma. J Hepatol 2018;69:182-236. Erratum in: J Hepatol 2019;70:817. [Crossref]
- Global Burden of Disease Liver Cancer Collaboration. The Burden of Primary Liver Cancer and Underlying Etiologies From 1990 to 2015 at the Global, Regional, and National Level: Results From the Global Burden of Disease Study 2015. JAMA Oncol 2017;3:1683-91. [Crossref] [PubMed]
- Malaguarnera G, Giordano M, Paladina I, et al. Serum markers of hepatocellular carcinoma. Dig Dis Sci 2010;55:2744-55. [Crossref] [PubMed]
- Okuda K. Early recognition of hepatocellular carcinoma. Hepatology 1986;6:729-38. [Crossref] [PubMed]
- Collier J, Sherman M. Screening for hepatocellular carcinoma. Hepatology 1998;27:273-8. [Crossref] [PubMed]
- Trevisani F, D'Intino PE, Morselli-Labate AM, et al. Serum alpha-fetoprotein for diagnosis of hepatocellular carcinoma in patients with chronic liver disease: influence of HBsAg and anti-HCV status. J Hepatol 2001;34:570-5. [Crossref] [PubMed]
- Saffroy R, Pham P, Reffas M, et al. New perspectives and strategy research biomarkers for hepatocellular carcinoma. Clin Chem Lab Med 2007;45:1169-79. [Crossref] [PubMed]
- Toso C, Asthana S, Bigam DL, et al. Reassessing selection criteria prior to liver transplantation for hepatocellular carcinoma utilizing the Scientific Registry of Transplant Recipients database. Hepatology 2009;49:832-8. [Crossref] [PubMed]
- Forner A, Bruix J. Biomarkers for early diagnosis of hepatocellular carcinoma. Lancet Oncol 2012;13:750-1. [Crossref] [PubMed]
- Wang M, Zhu J, Lubman DM, et al. Aberrant glycosylation and cancer biomarker discovery: a promising and thorny journey. Clin Chem Lab Med 2019;57:407-16. [Crossref] [PubMed]
- Dube DH, Bertozzi CR. Glycans in cancer and inflammation--potential for therapeutics and diagnostics. Nat Rev Drug Discov 2005;4:477-88. [Crossref] [PubMed]
- Peracaula R, Sarrats A, Rudd PM. Liver proteins as sensor of human malignancies and inflammation. Proteomics Clin Appl 2010;4:426-31. [Crossref] [PubMed]
- Turner GA. N-glycosylation of serum proteins in disease and its investigation using lectins. Clin Chim Acta 1992;208:149-71. [Crossref] [PubMed]
- Zhou J, Sun H, Wang Z, et al. Guidelines for the Diagnosis and Treatment of Hepatocellular Carcinoma (2019 Edition). Liver Cancer 2020;9:682-720. [Crossref] [PubMed]
- Laroy W, Contreras R, Callewaert N. Glycome mapping on DNA sequencing equipment. Nat Protoc 2006;1:397-405. [Crossref] [PubMed]
- Liu XE, Desmyter L, Gao CF, et al. N-glycomic changes in hepatocellular carcinoma patients with liver cirrhosis induced by hepatitis B virus. Hepatology 2007;46:1426-35. [Crossref] [PubMed]
- Reis CA, Osorio H, Silva L, et al. Alterations in glycosylation as biomarkers for cancer detection. J Clin Pathol 2010;63:322-9. [Crossref] [PubMed]
- Zou C, Huang C, Yan L, et al. Serum N-glycan profiling as a diagnostic biomarker for the identification and assessment of psoriasis. J Clin Lab Anal 2021;35:e23711. [Crossref] [PubMed]
- Wan L, Guo L, Hu Y, et al. Comparing the diagnostic value of serum oligosaccharide chain (G-test) and alpha-fetoprotein for hepatitis B virus-related liver cancer. Clin Biochem 2021;89:44-50. [Crossref] [PubMed]
- Link-Lenczowski P, Bubka M, Balog CIA, et al. The glycomic effect of N-acetylglucosaminyltransferase III overexpression in metastatic melanoma cells. GnT-III modifies highly branched N-glycans. Glycoconj J 2018;35:217-31. [Crossref] [PubMed]
- Kizuka Y, Taniguchi N. Enzymes for N-Glycan Branching and Their Genetic and Nongenetic Regulation in Cancer. Biomolecules 2016;6:25. [Crossref] [PubMed]
- Yao M, Zhou DP, Jiang SM, et al. Elevated activity of N-acetylglucosaminyltransferase V in human hepatocellular carcinoma. J Cancer Res Clin Oncol 1998;124:27-30. [Crossref] [PubMed]
- Ito Y, Miyoshi E, Sakon M, et al. Elevated expression of UDP-N-acetylglucosamine: alphamannoside beta1,6 N-acetylglucosaminyltransferase is an early event in hepatocarcinogenesis. Int J Cancer 2001;91:631-7. [Crossref] [PubMed]
- Zhao Y, Nakagawa T, Itoh S, et al. N-acetylglucosaminyltransferase III antagonizes the effect of N-acetylglucosaminyltransferase V on alpha3beta1 integrin-mediated cell migration. J Biol Chem 2006;281:32122-30. [Crossref] [PubMed]
- Giannini E, Risso D, Testa R. Transportability and reproducibility of the AST/ALT ratio in chronic hepatitis C patients. Am J Gastroenterol 2001;96:918-9. [Crossref] [PubMed]
- Silva IS, Ferraz ML, Perez RM, et al. Role of gamma-glutamyl transferase activity in patients with chronic hepatitis C virus infection. J Gastroenterol Hepatol 2004;19:314-8. [Crossref] [PubMed]
- Vanhooren V, Liu XE, Franceschi C, et al. N-glycan profiles as tools in diagnosis of hepatocellular carcinoma and prediction of healthy human ageing. Mech Ageing Dev 2009;130:92-7. [Crossref] [PubMed]
- Nie H, Liu X, Zhang Y, et al. Specific N-glycans of Hepatocellular Carcinoma Cell Surface and the Abnormal Increase of Core-α-1, 6-fucosylated Triantennary Glycan via N-acetylglucosaminyltransferases-IVa Regulation. Sci Rep 2015;5:16007. [Crossref] [PubMed]
- Huang C, Fang M, Feng H, et al. N-glycan fingerprint predicts alpha-fetoprotein negative hepatocellular carcinoma: A large-scale multicenter study. Int J Cancer 2021;149:717-27. [Crossref] [PubMed]
(English Language Editor: C. Betlazar-Maseh)