Effects of 2-dodecyl-6-methoxycyclohexa-2,5-diene-1,4-dione on autophagy and the PI3K/AKT/mTOR signaling pathway in human cholangiocarcinoma QBC939 cells
Introduction
Cholangiocarcinoma (CCA) is a malignant tumor derived from bile duct epithelial cells, and is the second most common hepatobiliary-related malignancy besides hepatocellular carcinoma, accounting for approximately 15% of all primary liver tumors. It can be divided into distal CCA, hilar CCA, and intrahepatic CCA according to the site of tumor occurrence from far to near, while 50–60% of cases are hilar CCAs (1-3). Epidemiological surveys have shown an increasing trend in the incidence and mortality of CCA, especially in Asian regions such as Thailand, China, and Korea (4). Radical resection is currently the most effective treatment for CCA, which has no specific symptoms in the early stage, has a low diagnostic rate, and is mostly clinically advanced when detected. CCA is prone to malignant biliary obstruction with liver metastases and liver failure, thus many patients miss the best time for radical surgical treatment (5). Most patients are treated with biliary drainage, radiotherapy, and chemotherapy, and the therapeutic effect is not satisfactory. Although immunotherapy and targeted therapy in recent years have shown some efficacy for certain patients, the limitations are obvious. As a result, the mortality rate of bile duct cancer remains high, and the 5-year survival rate after diagnosis is less than 10% (6). Therefore, the search for potential antitumor agents is one of the research priorities of CCA studies.
Autophagy is a catabolic process that captures and degrades damaged proteins and organelles in the lysosome (7). Autophagy occurs frequently during tumorigenesis and tumor chemotherapy. In general, autophagy plays a protective role for cancer cells during chemotherapy, which predisposes to tumor resistance and thus transformation into refractory cancer (8). At different stages of tumor development, autophagy may have opposite effects. CCA is a multistep event caused by genomic aberrations and dysregulation of signaling pathways (9,10). A study has demonstrated a significant increase in the amount of autophagy-associated proteins in CCA tissues (11). Autophagy can promote tumor cell survival during tumor progression by inhibiting the induction of p53 and maintaining mitochondrial metabolic function (12,13). Signaling pathways such as PI3K/AKT that regulate autophagy can significantly regulate epithelial mesenchymal transition and subsequently affect the progression and metastasis of CCA (14), and these studies confirm the close relationship between autophagy and CCA. Current studies show that various natural compounds such as curcumin and ginsenosides may have an important role in cancer prevention by participating in the regulation of oxidative stress responses, blocking proliferation, and regulating autophagy levels (15,16). Chloroquine and its derivative hydroxychloroquine (HCQ) are autophagy inhibitors, and more than 3 relevant clinical trials suggest that autophagy inhibitors are associated with antitumor activity (17,18). The PI3K/AKT/mTOR signaling pathway is widely involved in several biological processes such as cell proliferation, apoptosis, autophagy, and metabolism. A study has found that a variety of natural active ingredients exert their antitumor effects by targeting the PI3K/AKT/mTOR signaling pathway, and targeting autophagy induced by this pathway can be an important tool for tumor therapy and enhancing chemotherapy sensitivity (19). Studies have reported that impaired autophagy regulation is closely associated with carcinogenesis, metastasis and poor prognosis of bile duct cells and can be an effective anti-cancer target. Three autophagy-associated long-stranded non-coding RNAs have been identified as prognostic features of bile duct cancer (20,21).
2-dodecyl-6-methoxycyclohexa-2,5-diene-1,4-dione (DMDD) is a quinone extracted from the root of Populus tremula, with hypoglycemic, lipid-regulating, and inflammation-reducing effects. Recent studies have found that DMDD can inhibit the proliferation of breast, lung, and bone cancer cells, as well as inhibit the growth of breast cancer in mice (22-24). In this study, we investigated the effect of DMDD on human QBC939 CCA cells, and tentatively demonstrated that DMDD is a novel inducer of autophagy and induces autophagy by regulating the PI3K/AKT/mTOR signaling pathway in the QBC939 cell line. We present the following article in accordance with the ARRIVE reporting checklist (available at https://jgo.amegroups.com/article/view/10.21037/jgo-22-298/rc).
Methods
Drugs and cell culture
Yangtze root DMDD was prepared by our group. The human CCA cell line QBC9939 was purchased from Shanghai Cell Bank. QBC939 cells were revived and inoculated in Dulbecco’s modified Eagle’s medium (DMEM) (Biological Industries) containing 10% fetal bovine serum (FBS) (BI) and 1% cytochalasin (Solarbio) and placed in a cell culture incubator. Cells used for experiments were in logarithmic growth phase.
CCK8 assay for QBC939 cell viability
QBC939 cells at logarithmic growth stage were obtained, adjusted to a density of 5×104 cells/mL, and added to 96-well plates at 100 µL/well. Cells were incubated overnight in a CO2 incubator at 37 ℃. The control group and experimental group with different concentrations of DMDD (5, 10, 20, 40, and 80 µM/L) were set up and incubated for 24, 48, and 72 h. Then, 10 µL CCK8 solution was added and incubation was continued for 2 h. The absorbance value of each well was read at 450 nm on an enzyme standard instrument. Cell viability (%) = [optical density (OD) of experimental group − OD of control group]/(OD of control group − OD of blank group) × 100%, and the half-inhibitory concentration (IC50) of DMDD on QBC939 cells was calculated.
Scratch assay
The scratch test was performed to assess the migration ability of cells. QBC939 cells at logarithmic growth stage were obtained, and 1 ml of medium containing 2×105 cells was placed into each well of a 6-well plate and incubated in an incubator at 37 ℃ and 5% CO2 for 24 h. After the cells were spread, a 100 µL pipette tip was used to form a scratch on the cells in each well. QBC939 cells were divided into control, DMDD low concentration group (DMDD L), DMDD medium concentration group (DMDD M), and DMDD high concentration group (DMDD H), and then the cells in different groups were treated with the corresponding concentrations of DMDD in 2% FBS medium for 24 h. Finally, the cells were observed under an inverted light microscope and photographed and recorded, and the cell migration area was calculated by Image J software.
Transwell analysis
Transwell chambers (8 µm pore size, BD) were coated with Matrigel (Corning) and then placed in an incubator at 37 ℃ for 1 h to make them gel-like. QBC939 cells were treated with different concentrations of DMDD for 24 h. Cells were collected and resuspended in serum-free medium, then 300 µL of serum-free medium containing 3×104 cells was added to the transwell and 700 µL of medium containing 10% FBS was added to the lower chamber. After 24 h of incubation, the upper chamber was washed with PBS to remove the upper chamber cells. Cells were fixed on the lower side of the chamber with 4% paraformaldehyde, and crystalline violet (0.1%) was applied to stain the cells for 45 min at room temperature. After washing and drying, photographic recording was performed under an inverted microscope.
Flow cytometry cell cycle assay
Logarithmic growth phase QBC939 cells were inoculated in 6-well (3×105 cells) plates for 24 h, and DMDD medium of different concentrations was added for 24 h. The cell cycle was assessed by flow cytometry according to the Cell Cycle and Apoptosis Analysis Kit (Beyotime, C1052, China).
Cellular autophagy vesicle assay
Logarithmic growth phase QBC939 cells were inoculated in 6-well (3×105 cells) plates for 24 h. DMDD medium of different concentrations was added and incubated for 24 h. The Autophagy Staining Assay Kit with monodansylcadaverine (MDC) (Beyotime, C3018S) was used to detect cellular autophagy quickly and conveniently using MDC as a fluorescent probe. Staining was observed by fluorescence microscopy with photographs and Image J was used to calculate the average fluorescence intensity.
Western blot analysis
Different groups of cells were treated with corresponding concentrations of DMDD for 48 h. Then, QBC939 cells from different groups were digested and collected separately into centrifuge tubes. RIPA cell fast lysate (Beyotime) was mixed with protease inhibitor mixture (Beyotime) in a 100:1 ratio, and the mixture was used to extract total protein. The BCA kit (Beyotime) was used to detect protein concentration. After electrophoresis by SDS-PAGE, proteins were transferred to PVDF membranes (Millipore, ISEQ00010). Membranes were incubated with blocking solution at room temperature for 2 h. After washing with TrisBuffered Saline and Tween 20, membranes were incubated with primary antibodies overnight at 4 ℃. TBST washing was followed by the addition of secondary antibodies and incubation at room temperature for 2 h. The dark room was developed by Enhanced chemiluminescence, and grayscale values were analyzed by Image J software. β-actin was used as an internal reference. The antibodies PI3K, AKT, β-actin, HRP-conjugated Affinipure Goat Anti-Mouse IgG (H+L), and HRP-conjugated Affinipure Goat Anti-Rabbit IgG (H+L) were purchased from Proteintech Group, while mTOR, P62, Beclin 1, and MAP1LC3A antibodies were purchased from Sangon Biotech.
Animals
BALB/c mice (male, 5–6 weeks, 20–22 g) were purchased from the Animal Center of Guangxi Medical University, and all animal experiments were conducted with the approval of the Institutional Animal Care and Use Committee of the First Affiliated Hospital of Guangxi Medical University [No. 2022 (KY-E-031)]. Animal ethics review followed the national standard GB/T35892-2018 published by the Ministry of Science and Technology of the People’s Republic of China on the Guidance on the Handling of Laboratory Animals and the Guide to Ethical Review of Laboratory Animals-Animal Welfare. A protocol was prepared before the study without registration.
Establishment of the xenograft mouse model and animal management
QBC939 cells were cultured in large numbers to reach logarithmic growth phase, followed by digestion and collection. In BALB/c mice, 0.1 mL of cell suspension containing 1×109 QBC939 cells was transplanted subcutaneously in the right axilla to establish a subcutaneous transplantation tumor mouse model of bile duct cancer. The growth of tumors was measured every other day. When the tumor grew to 100 mm3, the mice were randomly divided into 4 groups: model group and DMDD-L group (DMDD, 10 µg/g), DMDD-M group (DMDD, 15 µg/g), and DMDD-H group (DMDD, 20 µg/g). Each group contained 3 mice, and tumor injection treatment was performed on alternate days 3 consecutive times. Mice were observed for 1 week, and then the mice were anesthetized and sacrificed.
Statistical analysis
SPSS 23 software was used to analyze the results. All data were expressed as mean ± standard deviation. Univariate analysis or the t-test was performed to compare the differences between experimental and control groups. P<0.05 was considered statistically significant.
Results
Effect of DMDD on the proliferation, migration, and invasion of CCA cells
The cell proliferation assay showed that DMDD had a significant inhibitory effect on QBC939 cells and showed concentration and time dependence (Figure 1). We set up subsequent experimental groupings, namely the control (CK) group, DMDD L group (10 µM), DMDD M group (15 µM), and DMDD H (20 µM) group. The effects of DMDD on migration, invasion, and the cell cycle were then examined. The results showed that DMDD could significantly inhibit the migration and invasion ability of tumor cells (Figure 2).
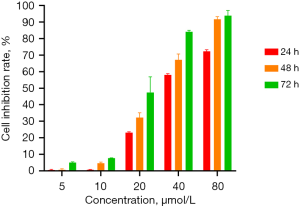
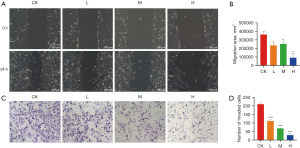
Effect of DMDD on the cell cycle and autophagy of CCA cells
Next, we treated the CCA cell line QBC939 according to the above grouped concentrations of DMDD, and examined the changes in the cell cycle after treatment with different concentrations. With the increase of drug concentration, the number of QBC939 cells in G1 phase gradually increased and the number of cells in S and G2 phases gradually decreased (Figure 3), suggesting that DMDD significantly induced G1 phase cell cycle arrest in QBC939 cells. In addition, we examined the effect of DMDD on autophagy in QBC939 cells using MDC as a fluorescent probe, and as the drug concentration increased, the autophagic vesicles of the cells increased compared with the control group (Figure 4).
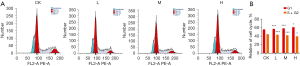
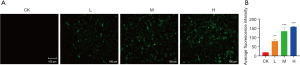
Effect of DMDD on the PI3K/AKT/mTOR signaling pathway in QBC939 cells
Western blot analysis showed that DMDD decreased the protein expression of PI3K, AKT, and mTOR and increased the expression of LC3-II/Ⅰ, Beclin-1, and P62 compared with the control group (Figure 5).
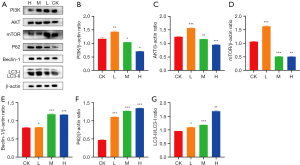
DMDD inhibits tumor growth in CCA mice
The success rate of the mouse CCA xenograft model was 100%. The tumor nodules reached 100 mm3 around day 10. The tumor volume increased slowly in the DMDD group, while the tumor volume increased significantly in the model group (Figure 6).
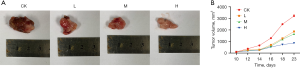
Discussion
Apoptosis is a programmed cell death process involving multiple factors. One of the characteristics of tumor cells is the lack of this programmed death process, and in this way, the induction of apoptosis becomes a means to combat tumors. It was shown that DMDD could inhibit cell proliferation and induce cell cycle arrest and apoptosis in 4T1 breast cancer cells (24). In addition, it was found that DMDD could inhibit apoptosis in HT22 mouse hippocampal neurons and improve cognitive impairment in diabetes mice (25). The effect of DMDD on CCA cells needs to be confirmed. The results of this experiment showed that DMDD treatment in QBC939 cells resulted in decreased cell viability and cell cycle arrest, suggesting that the induction of cell cycle arrest is one of the important mechanisms of DMDD inhibition of CCA, which is consistent with the current literature reports.
Cancer is the leading cause of death worldwide, and the incidence of cancer is increasing. Although there have been great advances in treatments for cancer, their efficacy remains limited (26). Autophagy is an evolutionarily conserved lysosomal degradation pathway prevalent in eukaryotic cells, through which autophagy can remove damaged organelles, maintain the stability of the intracellular environment and genome, and suppress inflammatory responses and oxidative stress (27). Under physiological conditions, cellular autophagic activity is sustained at low levels, which helps maintain cell survival and energy homeostasis (28,29). However, certain conditions can lead to excessive activation of autophagy. Autophagy exerts an inhibitory effect on tumor formation by reducing the accumulation of useless or damaged organelles and proteins and inhibiting processes such as oxidative stress. In this way, promoting autophagic activity or even inducing autophagic death in tumor cells can have an antitumor effect (30). One of the characteristics of autophagy is the production of autophagic vesicles with a bilayer membrane structure (31). The results of this study showed that after DMDD treatment in cells, the level of cellular autophagic vesicles was significantly increased by rapid detection using the MDC fluorescent probe. LC3, Beclin-1, and P62 are important markers of autophagy and can effectively respond to the level of autophagy (32,33). The results of Western blot analysis in this study showed that DMDD significantly increased Beclin-1, LC3 Ⅱ/I, and P62 protein expression compared with the blank control group. The above results suggest that DMDD may have the effect of inducing autophagy in QBC939 cells.
PI3K/AKT/mTOR is an important signaling pathway that regulates cell proliferation, migration, apoptosis, and autophagy. PI3K belongs to a family of lipid kinases that can be activated by many cytokine receptors. PI3K activation leads to AKT phosphorylation, which further activates downstream signaling molecules such as mTOR, thereby inhibiting autophagy (34). In contrast, mTOR is usually highly expressed in tumor cells, and inhibition of mTOR function, which inactivates the PI3K/AKT/mTOR signaling pathway, induces autophagy (35). Metastasis of CCA is an important cause of death in patients, and there is no effective method to predict and prevent metastasis of CCA. DMDD has a significant inhibitory effect on the migration and invasion of QBC939 cells. Blocking this cascade reaction may inhibit the migration and invasion of CCA cells (36,37). The PI3K/AKT/mTOR pathway is a central regulator of cell growth, survival and metabolism, and is involved in the development of biliary tract cancer. The mutations in PI3K, AKT, mTOR, and alterations in gene copy number are associated with poorer survival outcomes (38). Several preclinical studies have shown that PI3K/AKT/mTOR pathway inhibitors play a therapeutic role in biliary tract cancer (39).The results of Western blot analysis in this study showed that the expression levels of PI3K, AKT, and mTOR gradually decreased with the increase of DMDD concentration. The above results suggest that DMDD may have an inhibitory effect on the PI3K/AKT/mTOR signaling pathway in QBC939 cells. The inhibitory effect of DMDD on the migration and invasion of QBC939 cells may be through the inhibition of this signaling cascade.
There is an increasing number of antitumor studies on natural active substances associated with autophagy that are possibly involved in tumor immune escape. Ginsenoside Rh2, the main active component of ginseng, could inhibit cisplatin-induced autophagy and PD-L1 expression in tumor cells by increasing superoxide dismutase activity to enhance the antitumor function of cisplatin (40). Baicalein could increase the expression of autophagy initiator BECN1 and the autophagy protein LC3, and downregulate the expression of p-AKT and p-mTOR, thus inducing autophagy in breast cancer cells (41). In addition, strychnine was found to inhibit tumor cell autophagy through the ERK1/2-mTOR signaling pathway, while on the other hand, it could promote the proliferation of CD8+ T cells and induce immunogenic cell death in mice (42). All these studies suggest that some natural active substances can induce tumor cell autophagy and may be involved in regulating the immune response. In this study, it was found for the first time that DMDD can induce autophagy in CCA cells, and it may be used as a potential therapeutic agent. More anti-tumor mechanisms, as well as whether DMDD is involved in regulating immune responses, need to be confirmed by subsequent studies. DMDD may be helpful in the prevention and treatment of patients with CCA, but its application in the clinical setting still requires extensive research.
Conclusions
In summary, DMDD significantly induced cell cycle arrest and autophagy in QBC939 cells, and the specific molecular mechanism may be the inhibition of PI3K, AKT, and mTOR protein expression, which in turn downregulates the PI3K/AKT/mTOR signaling pathway.
Acknowledgments
Funding: This study was supported by the National Natural Science Foundation of China (Nos. 81560535, 81802874, 81072321) and the Doctoral research startup fund projects in natural sciences of Guangxi University of Chinese Medicine (No. 2018BS07).
Footnote
Reporting Checklist: The authors have completed the ARRIVE reporting checklist. Available at https://jgo.amegroups.com/article/view/10.21037/jgo-22-298/rc
Data Sharing Statement: Available at https://jgo.amegroups.com/article/view/10.21037/jgo-22-298/dss
Conflicts of Interest: All authors have completed the ICMJE uniform disclosure form (available at https://jgo.amegroups.com/article/view/10.21037/jgo-22-298/coif). The authors have no conflicts of interest to declare.
Ethical Statement: The authors are accountable for all aspects of the work in ensuring that questions related to the accuracy or integrity of any part of the work are appropriately investigated and resolved. The animal experiments were approved by the Ethics Committee of the First Affiliated Hospital of Guangxi Medical University (No. 2022 (KY-E-031)). Animal ethics review followed the national standard GB/T35892-2018 published by the Ministry of Science and Technology of the People’s Republic of China on the Guidance on the Handling of Laboratory Animals and the Guide to Ethical Review of Laboratory Animals-Animal Welfare.
Open Access Statement: This is an Open Access article distributed in accordance with the Creative Commons Attribution-NonCommercial-NoDerivs 4.0 International License (CC BY-NC-ND 4.0), which permits the non-commercial replication and distribution of the article with the strict proviso that no changes or edits are made and the original work is properly cited (including links to both the formal publication through the relevant DOI and the license). See: https://creativecommons.org/licenses/by-nc-nd/4.0/.
References
- Gatto M, Alvaro D. New insights on cholangiocarcinoma. World J Gastrointest Oncol 2010;2:136-45. [Crossref] [PubMed]
- Goral V. Cholangiocarcinoma: New Insights Asian Pac J Cancer Prev 2017;18:1469-73. [PubMed]
- Banales JM, Marin JJG, Lamarca A, et al. Cholangiocarcinoma 2020: the next horizon in mechanisms and management. Nat Rev Gastroenterol Hepatol 2020;17:557-88. [Crossref] [PubMed]
- Khan SA, Tavolari S, Brandi G. Cholangiocarcinoma: Epidemiology and risk factors. Liver Int 2019;39:19-31. [Crossref] [PubMed]
- Doherty B, Nambudiri VE, Palmer WC. Update on the Diagnosis and Treatment of Cholangiocarcinoma. Curr Gastroenterol Rep 2017;19:2. [Crossref] [PubMed]
- Razumilava N, Gores GJ. Classification, diagnosis, and management of cholangiocarcinoma. Clin Gastroenterol Hepatol 2013;11:13-21.e1; quiz e3-4. [Crossref] [PubMed]
- White E. The role for autophagy in cancer. J Clin Invest 2015;125:42-6. [Crossref] [PubMed]
- Li YJ, Lei YH, Yao N, et al. Autophagy and multidrug resistance in cancer. Chin J Cancer 2017;36:52. [Crossref] [PubMed]
- Ong CK, Subimerb C, Pairojkul C, et al. Exome sequencing of liver fluke-associated cholangiocarcinoma. Nat Genet 2012;44:690-3. [Crossref] [PubMed]
- Rizvi S, Khan SA, Hallemeier CL, et al. Cholangiocarcinoma - evolving concepts and therapeutic strategies. Nat Rev Clin Oncol 2018;15:95-111. [Crossref] [PubMed]
- Sasaki M, Nitta T, Sato Y, et al. Autophagy may occur at an early stage of cholangiocarcinogenesis via biliary intraepithelial neoplasia. Hum Pathol 2015;46:202-9. [Crossref] [PubMed]
- Qu X, Sheng J, Shen L, et al. Autophagy inhibitor chloroquine increases sensitivity to cisplatin in QBC939 cholangiocarcinoma cells by mitochondrial ROS. PLoS One 2017;12:e0173712. [Crossref] [PubMed]
- Huang T, Song X, Yang Y, et al. Autophagy and Hallmarks of Cancer. Crit Rev Oncog 2018;23:247-67. [Crossref] [PubMed]
- Chen HT, Liu H, Mao MJ, et al. Crosstalk between autophagy and epithelial-mesenchymal transition and its application in cancer therapy. Mol Cancer 2019;18:101. [Crossref] [PubMed]
- Zhang SF, Wang XL, Yang XQ, et al. Autophagy-associated targeting pathways of natural products during cancer treatment. Asian Pac J Cancer Prev 2014;15:10557-63. [Crossref] [PubMed]
- Shakeri A, Cicero AFG, Panahi Y, et al. Curcumin: A naturally occurring autophagy modulator. J Cell Physiol 2019;234:5643-54. [Crossref] [PubMed]
- Sui X, Chen R, Wang Z, et al. Autophagy and chemotherapy resistance: a promising therapeutic target for cancer treatment. Cell Death Dis 2013;4:e838. [Crossref] [PubMed]
- Onorati AV, Dyczynski M, Ojha R, et al. Targeting autophagy in cancer. Cancer 2018;124:3307-18. [Crossref] [PubMed]
- Xu Z, Han X, Ou D, et al. Targeting PI3K/AKT/mTOR-mediated autophagy for tumor therapy. Appl Microbiol Biotechnol 2020;104:575-87. [Crossref] [PubMed]
- Liu YJ, Hounye AH, Wang Z, et al. Identification and Validation of Three Autophagy-Related Long Noncoding RNAs as Prognostic Signature in Cholangiocarcinoma. Front Oncol 2021;11:780601. [Crossref] [PubMed]
- Aredia F, Giansanti V, Mazzini G, et al. Multiple effects of the Na(+)/H (+) antiporter inhibitor HMA on cancer cells. Apoptosis 2013;18:1586-98. [Crossref] [PubMed]
- Zheng N, Lin X, Wen Q, et al. Effect of 2-dodecyl-6-methoxycyclohexa-2,5-diene-1,4-dione, isolated from Averrhoa carambola L. (Oxalidaceae) roots, on advanced glycation end-product-mediated renal injury in type 2 diabetic KKAy mice. Toxicol Lett 2013;219:77-84. [Crossref] [PubMed]
- Xie Q, Zhang S, Chen C, et al. Protective Effect of 2-Dodecyl-6-Methoxycyclohexa-2, 5-Diene-1, 4-Dione, Isolated from Averrhoa Carambola L., Against Palmitic Acid-Induced Inflammation and Apoptosis in Min6 Cells by Inhibiting the TLR4-MyD88-NF-κB Signaling Pathway. Cell Physiol Biochem 2016;39:1705-15. [Crossref] [PubMed]
- Zhou X, Wu X, Qin L, et al. Anti-Breast Cancer Effect of 2-Dodecyl-6-Methoxycyclohexa-2,5-Diene-1,4-Dione in vivo and in vitro Through MAPK Signaling Pathway. Drug Des Devel Ther 2020;14:2667-84. [Crossref] [PubMed]
- Wang L, Cao J, Xu Q, et al. 2-Dodecyl-6-Methoxycyclohexa-2,5-Diene-1,4-Dione Ameliorates Diabetic Cognitive Impairment Through Inhibiting Hif3α and Apoptosis. Front Pharmacol 2021;12:708141. [Crossref] [PubMed]
- Sung H, Ferlay J, Siegel RL, et al. Global Cancer Statistics 2020: GLOBOCAN Estimates of Incidence and Mortality Worldwide for 36 Cancers in 185 Countries. CA Cancer J Clin 2021;71:209-49. [Crossref] [PubMed]
- Yun CW, Lee SH. The Roles of Autophagy in Cancer. Int J Mol Sci 2018;19:3466. [Crossref] [PubMed]
- Wu QQ, Liu C, Cai Z, et al. High-mobility group AT-hook 1 promotes cardiac dysfunction in diabetic cardiomyopathy via autophagy inhibition. Cell Death Dis 2020;11:160. [Crossref] [PubMed]
- Byrnes K, Blessinger S, Bailey NT, et al. Therapeutic regulation of autophagy in hepatic metabolism. Acta Pharm Sin B 2022;12:33-49. [Crossref] [PubMed]
- Enshell-Seijffers D, Lindon C, Morgan BA. The serine protease Corin is a novel modifier of the Agouti pathway. Development 2008;135:217-25. [Crossref] [PubMed]
- Mowers EE, Sharifi MN, Macleod KF. Autophagy in cancer metastasis. Oncogene 2017;36:1619-30. [Crossref] [PubMed]
- Amaravadi R, Kimmelman AC, White E. Recent insights into the function of autophagy in cancer. Genes Dev 2016;30:1913-30. [Crossref] [PubMed]
- Ashkenazi A, Bento CF, Ricketts T, et al. Polyglutamine tracts regulate beclin 1-dependent autophagy. Nature 2017;545:108-11. [Crossref] [PubMed]
- Manthari RK, Tikka C, Ommati MM, et al. Arsenic induces autophagy in developmental mouse cerebral cortex and hippocampus by inhibiting PI3K/Akt/mTOR signaling pathway: involvement of blood-brain barrier's tight junction proteins. Arch Toxicol 2018;92:3255-75. [Crossref] [PubMed]
- Kim SH, Son KM, Kim KY, et al. Deoxypodophyllotoxin induces cytoprotective autophagy against apoptosis via inhibition of PI3K/AKT/mTOR pathway in osteosarcoma U2OS cells. Pharmacol Rep 2017;69:878-84. [Crossref] [PubMed]
- Chen G, Yue Y, Qin J, et al. Plumbagin suppresses the migration and invasion of glioma cells via downregulation of MMP-2/9 expression and inaction of PI3K/Akt signaling pathway in vitro. J Pharmacol Sci 2017;134:59-67. [Crossref] [PubMed]
- Zhu P, Liu Z, Zhou J, et al. Tanshinol inhibits the growth, migration and invasion of hepatocellular carcinoma cells via regulating the PI3K-AKT signaling pathway. Onco Targets Ther 2019;12:87-99. [Crossref] [PubMed]
- Corti F, Nichetti F, Raimondi A, et al. Targeting the PI3K/AKT/mTOR pathway in biliary tract cancers: A review of current evidences and future perspectives. Cancer Treat Rev 2019;72:45-55. [Crossref] [PubMed]
- Wu CE, Chen MH, Yeh CN. mTOR Inhibitors in Advanced Biliary Tract Cancers. Int J Mol Sci 2019;20:500. [Crossref] [PubMed]
- Chen Y, Zhang Y, Song W, et al. Ginsenoside Rh2 Improves the Cisplatin Anti-tumor Effect in Lung Adenocarcinoma A549 Cells via Superoxide and PD-L1. Anticancer Agents Med Chem 2020;20:495-503. [Crossref] [PubMed]
- Yan W, Ma X, Zhao X, et al. Baicalein induces apoptosis and autophagy of breast cancer cells via inhibiting PI3K/AKT pathway in vivo and vitro. Drug Design Development and Therapy 2018;12:3961-72. [Crossref] [PubMed]
- Ishimwe N, Wei P, Wang M, et al. Autophagy Impairment through Lysosome Dysfunction by Brucine Induces Immunogenic Cell Death (ICD). Am J Chin Med 2020;48:1915-40. [Crossref] [PubMed]