IL-1 receptor-associated kinase 1 participates in the modulation of the NLRP3 inflammasome by tumor-associated macrophages in hepatocellular carcinoma
Introduction
Hepatocellular carcinoma (HCC) is a common tumor of the digestive system and is associated with high mortality worldwide (1). In 2022, an estimated 41,260 people will be diagnosed with HCC and 30,520 patients will die with HCC in the United States alone (2). Tumor-associated macrophages (TAMs) are macrophages infiltrating tumor tissues, mainly differentiated from monocytes (3). Chemokines, such as colony-stimulating factor 1 (CSF1) and C-C motif chemokine ligand 2 (CCL2), secreted by tumor cells can recruit monocytes in peripheral blood to tumor microenvironment (TME) and differentiate monocytes into macrophages (3). Previous study reported that TAMs are the most abundant immune cells in the TME and play a pivotal role in tumor progression of inflammation-related cancer, including HCC (4). There are two polarizations of TAMs in TME: M1-type macrophages and M2-type macrophages (3). M1-type macrophages can kill tumor cells and resist the invasion of pathogens, while M2-type macrophages mainly play a role in promoting tumor growth, invasion and metastasis (5). Among them, M2-type macrophages are main macrophages in TME, which exert tumor-promoting and immunosuppressive role via multiple pathways (3,5), such as accelerating tumor cell proliferation via secreting epidermal growth factor (EGF) and platelet-derived growth factor (PDGF), participating in tumor invasion and metastasis through releasing matrix metalloproteinases (MMPs, such as MMP2 and MMP9), joining in tumor cell immune escape via producing interleukin 10 (IL-10), prostaglandin E2 (PGE2) and tumor transforming growth factor β (TGFβ), as well as taking part in the growth of tumor micro-vessels and lymphatic vessels by expressing vascular endothelial growth factor (VEGF). For HCC, previous literature reported that an increased M2-type macrophage population was related to the poor prognosis of HCC (6). Moreover, it was discovered that TAMs took part in the modulation of HCC proliferation, angiogenesis, epithelial-mesenchymal transition (EMT) and metastasis, as well as reduced adaptive immunity (7).
The Toll-like receptor (TLR)/interleukin-1 (IL-1) receptor protein (IL-1R) signaling pathway is a major component of the innate immune system (8). As a key mediator of the TLR/IL-1R signaling pathway, IL-1 receptor-associated kinase 1 (IRAK1) can trigger a series of signaling events which ultimately results in the elevated expression of inflammatory-related genes (9). Indeed, elevated expression of IRAK1 has been widely observed in multiple human cancers (10), including in HCC (11). Cheng et al. (12) reported that IRAK1 promotes cancer stemness and drug resistance of HCC cells via modulating the activator protein 1 (AP-1)/aldo-keto reductase family 1 member 10 (AKR1B10) pathway. Ye et al. (11) demonstrated that HCC patients with high IRAK1 expression had larger tumor size and metastatic rate, and this may represent a valuable target for HCC diagnosis and therapy. However, it remains unclear whether there is an interaction between TAMs and IRAK1 in modulating the development of HCC.
The NLR family pyrin domain-containing 3 (NLRP3) inflammasomes play a key role in innate immunity by identifying multiple pathogenic microorganisms and endogenous modulatory molecules, which can activate caspase-1 to induce the maturation and release of IL-1β and IL-18 (13). The aberrant expression of NLRP3 has been shown to participate in the pathogenesis of inflammation-related human diseases via facilitating a chronic inflammatory response (14). Considering that inflammation has been shown to be involved in the progression of multiple human cancers including HCC, the regulatory effects of NLRP3 on cancer growth and metastasis has been become a hotspot in molecular biology research (15,16). Chen et al. (17) reported that IRAK1 silencing could suppress the malignant transformation of HCC cells via inhibiting the MAPKs/NLRP3/IL-1β pathway.
In the current research, the expressions of IRAK1, NLRP3, and macrophage markers were assessed in HCC tissues and compared with that of adjacent healthy tissues. The modulatory roles of IRAK1 on HCC cell proliferation, migration, and invasion, as well as the effects of IRAK1 on the expression of NLRP3 and pro-inflammatory cytokines were examined. Furthermore, whether IRAK1 participated in the regulation of TAMs on HCC cell functions were investigated. This report provides insights into the association between IRAK1, NLRP3, and TAMs in the regulation of HCC growth and metastasis. The following article is presented in accordance with the MDAR reporting checklist (available at https://jgo.amegroups.com/article/view/10.21037/jgo-22-471/rc).
Methods
Clinical samples
A total of 30 patients (18 male and 12 female, 27–73 years old) who were diagnosed with HCC and did not receive any therapy before were recruited for this study in People’s Hospital of Huadu District (Guangzhou, China). Samples of the tumor tissues and adjacent tumor tissues (distance over 3 cm from tumor tissues) were obtained during surgery. The study was conducted in accordance with the Declaration of Helsinki (as revised in 2013). This study was approved by the institutional ethics board of the People’s Hospital of Huadu District (No. 2020043) and informed consent was obtained from all patients.
Cell culture and transfection
The HCC cell lines, HepG2 and Huh7, were purchased from Procell Inc. (CL-0103, CL-0120, Wuhan, China) and subjected to STR identification. Cells were cultured in Dulbecco’s Modified Eagle Medium (DMEM; D0819, Sigma-Aldrich, MO, USA), supplemented with 10% fetal bovine serum (FBS; 12103C, Sigma-Aldrich) and 1% penicillin-streptomycin solution (PB180120, Procell Inc., Wuhan, China), at 37 ℃ with 5% CO2. Small interfering RNA targeting IRAK1 (si-IRAK1) were constructed to silence IRAK1 expression in HepG2 and Huh7 cells. The sequence of the si-IRAK1 was 5'-GCCCGAAGAAAGUGAUGAAUU-3'. Cell transfection was performed using Lipo2000 Transfection Reagent (11668-500, Invitrogen, CA, USA) according to the manufacturer’s instructions.
Induction of tumor-associated macrophages (TAMs)
The induction of TAMs was performed as previously reported (18). Briefly, HCC-conditioned medium was prepared by culturing 1×107 HepG2 cells in culture flasks for 24 hours. After culture in DMEM containing 10% human AB serum (34005, Gibco, CA, USA) for 48 hours, the culture supernatant was collected. Human peripheral blood was acquired from healthy volunteers (male, aged 20–30 years) and CD14+ PBMCs were harvested using the auto-MACS Pro Separator (Miltenyi Biotec GmbH, Germany). PBMCs were induced to convert TAMs by stimulating with 25 ng/mL human macrophage colony-stimulating factor (hM-CSF; 102-09, Sciencell, CA, USA) for 6 days, followed by 50% HCC-conditioned medium for 2 days.
Quantitative real-time polymerase chain reaction (qRT-PCR)
Total RNA was isolated from tissues and cells using Trizol Reagent (9109, Takara Biotechnology, Beijing, China). The Ultra-trace UV analyzer (Q6000UV, Quawell Technology, CA, USA) was used to determine the total RNA concentration and 1 µg total RNA was used to synthesize the cDNA using the Bestar qPCR RT Kit (2220, DBI Bioscience, Shanghai, China). The Bestar qPCR MasterMix (2043, DBI Bioscience) was used for detection of IRAK1 and NLRP3 mRNA expression. Glyceraldehyde 3-phosphate dehydrogenase (GAPDH) expression was used as the internal control and relative expression of mRNA was calculated using the 2−ΔΔCt method. The primer’s sequences are displayed in Table 1.
Table 1
Name | Sequence (5'-3') | Product length (bp) |
---|---|---|
IRAK1 forward | TGAGGAACACGGTGTATGCTG | 119 |
IRAK1 reverse | GTTTGGGTGACGAAACCTGGA | |
NLRP3 forward | GATCTTCGCTGCGATCAACAG | 81 |
NLRP3 reverse | CGTGCATTATCTGAACCCCAC | |
GAPDH forward | TGTTCGTCATGGGTGTGAAC | 154 |
GAPDH reverse | ATGGCATGGACTGTGGTCAT |
PCR, polymerase chain reaction; IRAK1, interleukin-1 receptor-associated kinase 1; NLRP3, NLR family pyrin domain-containing 3; GAPDH, glyceraldehyde 3-phosphate dehydrogenase.
Immunohistochemistry (IHC) assay
IHC was performed to detect the expression of CD68, CD163, and CD169 in tumor tissues and the adjacent healthy tissues. Briefly, following de-paraffinization and hydration, 6 µm paraffin-embedded sections were incubated with EDTA antigen retrieval solution (P0085, Beyotime Biotechnology, Shanghai, China) for 20 minutes at 95 ℃ and 0.5% TritonX-100 solution for 20 minutes at 25 ℃. To quench the internal peroxidase activity, sections were treated with 3% hydrogen peroxide solution for 10 minutes. After incubation with 3% bovine serum albumin (BSA; ST023, Beyotime Biotechnology) for 30 minutes, sections were incubated with an anti-CD68 antibody (ab955), anti-CD163 antibody (ab182422), or anti-CD169 antibody (ab18619, Abcam Biotechnology, MA, USA) at 4 ℃ for 12 hours, followed by incubation with the relevant horse-radish peroxidase (HRP)-conjugated secondary antibody (A0208, A0216) for 1 hour. Relative expressions were visualized using DAB solution (P0202, Beyotime Biotechnology). Hematoxylin solution was used for staining the cell nucleus. The results were observed under light microscopy (Leica, Germany).
Cell counting kit-8 (CCK-8) assay
Following si-IRAK1 transfection and/or co-culture with TAMs, the viability of HepG2 and Huh7 cells was assessed using the CCK-8 assay (C0038, Beyotime Biotechnology). Briefly, 1×104 cells were seeded into 96-well plates for 0, 24, or 48 hours. Subsequently, 10 µL CCK-8 solution was added into the culture medium for 1 hour. The absorbance of each well at 450 nm was measured.
Cell proliferation assay
Following the relevant stimulation, the proliferation of HepG2 and Huh7 cells was determined using the Edu incorporation assay (CA1170, Solarbio, Beijing, China). Briefly, 1×104 cells were cultivated on cover glasses in 6-well plates overnight. Thereafter, 50 µM Edu solution was added into the culture medium and incubated at 37 ℃ for 2 hours. After fixation with 4% paraformaldehyde solution for 15 minutes, cells were incubated with 0.1% TritonX-100 solution for 10 minutes. Cells were then stained with Apollo solution for 30 minutes in the dark. Hoechst 33324 was used to dye the cell nucleus. Results were observed under a fluorescence inverted microscope (OLYMPUS, Tokyo, Japan).
Cell migration and invasion assay
Following the relevant stimulation, the cell migration and invasion ability of HepG2 and Huh7 cells was assessed using the two-chamber transwell assay (Costar, Coring Incorporated, NY, USA). The transwell membrane was pre-coated with Matrigel (BD Incorporated, NJ, USA) for the detection of cell invasion ability. Briefly, 4×102 cells in 200 µL FBS-free culture medium were seeded in the upper chamber. The lower chamber was filled with 600 µL complete culture medium. After incubation at 37 ℃ for 48 hours, the non-migrated cells (or non-invaded cells) in the upper chamber were removed. The migrated (or invaded) cells were then fixed using 4% paraformaldehyde solution for 30 minutes and stained with 0.1% crystal violet solution for 10 minutes. The results were visualized under light microscopy (Leica, Germany).
Enzyme linked immunosorbent assay (ELISA)
ELISAs were performed to measure the concentration of IL-1β, IL-18, and monocyte chemotactic protein 1 (MCP-1) in the culture supernatant of HepG2 and Huh7 cells after different stimuli. The ELISA kits were purchased from Solarbio (SEKH-0002, SEKH-0028 and SEKH-0236) and conducted in accordance with the manufacturer’s protocols.
Western blotting
After different stimuli, the total proteins were isolated from cells using RIPA lysis buffer (Beyotime Biotechnology). Western blotting was conducted as previously described (19). The primary antibodies, anti-IRAK1 (ab238) and anti-NLRP3 (ab263899), as well as the secondary antibody, HRP-conjugated goat anti-rabbit IgG H&L (ab205718), were obtained from Abcam Biotechnology (MA, USA).
Statistical analysis
Data are shown as mean ± standard deviation (SD) from three repeated experiments using GraphPad Prism 8.0 software. The P values were calculated using Student’s t-test, one-way analysis of variance (ANOVA), or two-way ANOVA with a significance level set at P<0.05.
Results
IRAK1, NLRP3, and macrophage markers were all highly expressed in HCC tissues
Both IRAK1 and NLRP3 mRNA levels were notably increased in tumor tissues compared to adjacent healthy tissues (P<0.01 or P<0.001; Figure 1A,1B). Furthermore, the expression of IRAK1 was positively correlated to NLRP3 expression in hepatic cancer tissues (P<0.0001; Figure 1C). IHC assays demonstrated that the expression of the macrophage markers CD68, CD163, and CD169 was significantly elevated in tumor tissues compared to adjacent healthy tissues (Figure 1D). These results demonstrated that HCC tissues showed elevated expression of IRAK1, NLRP3, and macrophage markers.
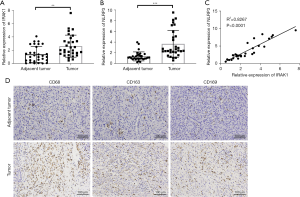
IRAK1 silencing suppressed HCC cell proliferation, migration, and invasion
Transfection of si-IRAK1 in HepG2 and Huh7 cells markedly lowered both the mRNA and protein expression of IRAK1 (P<0.001 for mRNA expression; Figure 2A,2B). Furthermore, si-IRAK1 transfection lowered the viability of both HepG2 and Huh7 cells (P<0.05 and P<0.01, respectively; Figure 3A,3B). Moreover, relative to the negative control (NC) cells, si-IRAK1 significantly reduced cell proliferation, migration, and invasion of HepG2 and Huh7 cells (P<0.01; Figure 3C-3E). These results demonstrated that IRAK1 silencing inhibited HCC cell proliferation, migration, and invasion.
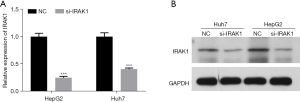
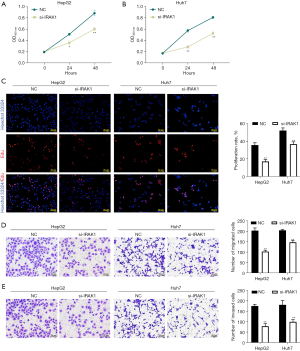
IRAK1 silencing reduced the expression of NLRP3 and pro-inflammatory factors
IRAK1 silencing lowered the NLRP3 protein levels in HepG2 and Huh7 cells (Figure 4A). In addition, cells transfected with si-IRAK1 showed reduced concentrations of IL-1β, IL-18, and MCP-1 in the culture supernatant compared to NC cells (P<0.001; Figure 4B-4D). These outcomes suggested that IRAK1 silencing reduced NLRP3 expression and the expression of pro-inflammatory factors in HCC cells.
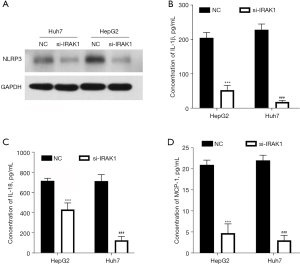
IRAK1 silencing reversed the TAM-induced increase in HCC cell proliferation, migration, and invasion
TAMs have been shown to be involved in the modulation of HCC cell growth and metastasis (20). Co-culture of HepG2 and Huh7 cells with TAMs increased cell viability (P<0.05; Figure 5A), however, si-IRAK1 transfection reversed the TAM-induced increase in cell viability (P<0.05 or P<0.01 compared to NC + TAMs group). The proliferation, migration, and invasion of HepG2 and Huh7 cells were all notably increased after co-culture with TAMs compared to NC cells that were not co-cultured with TAMs (P<0.01; Figure 5B-5D). However, si-IRAK1 cells co-cultured with TAMs showed reduced proliferation, migration, and invasion compared to NC cells co-cultured with TAMs (P<0.01). These results suggested that IRAK1 silencing reversed the increases in HCC cell proliferation, migration, and invasion induced by co-culture with TAMs.
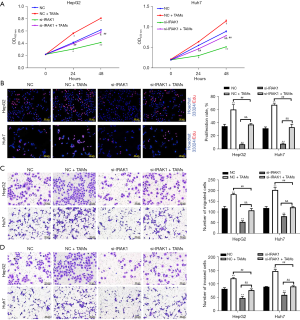
IRAK1 silencing reversed the TAM-induced increase in expression of NLRP3 and pro-inflammatory factors in HCC cells
Co-culture with TAMs enhanced the protein expression of NLRP3 in HepG2 and Huh7 cells (Figure 6A). In addition, the levels of IL-1β, IL-18, and MCP-1 in the culture supernatant of cells co-cultured with TAMs was elevated compared to NC cells (P<0.001; Figure 6B-6D). However, si-IRAK1 cells co-cultured with TAMs showed remarkably reduced NLRP3 protein expression, as well as reduced IL-1β, IL-18, and MCP-1 levels compared to the NC + TAMs group (P<0.001). These results suggested that IRAK1 silencing reversed the TAM-induced increase in NLRP3 expression and pro-inflammatory factors in HCC cells.
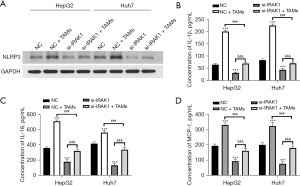
Discussion
As a common digestive system cancer, HCC threatens the morbidity and mortality of many individuals (1). In recent years, the regulatory role of inflammation on cancer initiation and development have gained much attention (21). Indeed, HCC has been shown to be an inflammation-related cancer and over 90% of HCC is associated with inflammation (22,23). Chronic and persistent inflammation damages hepatic cells, results in fibrosis and cirrhosis, and eventually leads to HCC (23). A review article by Yu et al. (24) reported that inflammation may advance HCC cell survival, proliferation, invasion, and metastasis; induce angiogenesis and development of cancer stem cells; evade immune surveillance; as well as trigger genomic instability of HCC cells.
IRAK1 plays a key role in the innate immune system by acting as a mediator of the TLRs/IL-1Rs signaling pathway to induce the expression of multiple pro-inflammatory cytokines (9). Earlier literature reported that the upregulation of IRAK1 in HCC tissues was associated with the progression of HCC (25). IRAK1 upregulation has been demonstrated to promote HCC cell proliferation and limit cell apoptosis, as well as boost HCC stemness and drug resistance (26). This current study demonstrated that IRAK1 was highly expressed in HCC tissues. Furthermore, IRAK1 silencing lowered the proliferation, migration, and invasion of the HCC cells HepG2 and Huh7. These findings further confirmed the oncogenic role of IRAK1 in HCC.
The NLRP3 inflammasome is a multiprotein complex, consisting of a sensor (NLRP3), an adaptor (apoptosis-associated speck-like protein containing CARD, ASC, also known as PYCARD), and an effector (caspase 1) (27). It is involved in the mammalian cell response to multiple stresses and participates in caspase-dependent pyroptosis (28). Earlier literature reported that there was two signal model responsible for NLRP3 inflammasome activation (29). One is stimulated by microbial molecules or endogenous cytokines and activate NLRP3 inflammasome via nuclear factor kappa B (NF-κB) pathway (30). Caspase 8 and FAS-associated death domain protein (FADD) are involved in the activation of NF-κB pathway (29,31). Another is stimulated by multiple stimuli, such as ATP or viral RNA, and activate NLRP3 inflammasome through massive K+ efflux (29). Mitochondrial dysfunction and Ca2+ signaling are associated with activation of NLRP3 inflammasome (16). While previous study has demonstrated that NLRP3 inflammasome is associated with the pathogenesis of several human cancers (32), its role in HCC progression remains controversial. Wei et al. (28) reported that activation of NLRP3 inflammasome promotes HCC cell pyroptosis and suppresses cell autophagy. Fan et al. (33) showed that inhibition of NLRP3 reduced the proliferation and metastasis of Hep3B and SNU-449 HCC cells. However, Wei et al. (34) demonstrated that upregulation of NLRP3 suppressed the malignant behavior of HCC cells. Previous literature reported that IRAK1 participates in the activation of NLRP3 both in the MyD88 pathway and the TLRs/IL-1R/interferon β (IFN-β) pathway in macrophages (35). Moreover, Chen et al. (17) discovered that IRAK1 silencing induced the inactivation of NLRP3 in HCC cells. Herein, we demonstrated that NLRP3 is also highly expressed in HCC tissues, which support the oncogenic role of NLRP3 in HCC. More importantly, we found that the expression of IRAK1 was positively correlated to NLRP3 expression in HCC tissues. IRAK1 silencing reduced the expression of NLRP3 in HCC cells, as well as the expression of pro-inflammatory cytokines IL-1β, IL-18, and MCP-1. These discoveries proposed the regulatory activity of IRAK1 on NLRP3 inflammasome. The findings of our research provide new theoretical and experimental basis for further understanding the regulatory mechanism of NLRP3 inflammasome.
TAMs are the most common immune cells in TME, which plays critical role in tumor-related inflammatory responses (4). Recent years, some studies have focused on the regulatory role of TAMs on HCC progression (20,36). Wan et al. (37) discovered that TAMs promoted expansion of human HCC stem cells via producing interleukin 6 (IL-6) and regulating signal transducer and activator of transcription 3 (STAT3) pathway. Ao et al. (38) found that the blockade of CSF1 receptor suppressed HCC growth via altering the polarization of TAMs in TME. For the modulation between TAMs, IRAK1 and NLRP3 inflammasome, Wang et al. (39) found that TAM-derived exosomes inhibited endometrial cancer development via the IRAK1/NF-κB pathway. Hofbauer et al. (40) revealed that the activation of the NLRP3 inflammasome in TAMs accelerated the development of multiple myeloma. CD68, CD163, and CD168 are main indicators of M2-like TAMs (41,42). In this current research, the CD68, CD163, and CD168 expressions were all increased in HCC tissues, suggesting the presence of M2-like TAMs in HCC tissues. Moreover, co-culture with TAMs promoted HCC cell proliferation, migration, and invasion, while IRAK1 silencing reversed the effects of TAM co-culture on HCC cell functions. This suggested that IRAK1 is involved in the regulation of HCC cell function by TAMs. In addition, co-culture with TAMs increased the expression of NLRP3, IL-1β, IL-18, and MCP-1 in HCC cells, suggesting that TAMs could activate the NLRP3 inflammasome in HCC cells. IRAK1 silencing reversed the influence of TAMs on NLRP3, IL-1β, IL-18, and MCP-1 expressions in HCC cells, suggesting that IRAK1 participates in the regulation of HCC cell function by TAMs via influencing the activation of the NLRP3 inflammasome.
In conclusion, this current investigation verified the roles of IRAK1, NLRP3, and TAMs on HCC progression. The expression of IRAK1, NLRP3, and M2-like TAMs were all elevated in HCC. IRAK1 was involved in the modulation of HCC growth and metastasis, as well as activation of the NLRP3 inflammasome. The ability of TAMs to promote HCC growth and metastasis may be mediated by the activation of the NLRP3 inflammasome and the expression of IRAK1. Currently, the tumor therapeutic strategies targeting TAMs have three main directions: inhibition the recruitment and M2 polarization of macrophages, suppression angiogenesis and tissue remodeling, limitation the immunosuppressive effects and restore the anti-tumor cytotoxicity of TAMs. We revealed the regulatory mechanism between TAMs, IRAK1 and NLRP3 inflammasome, which may provide new biomarker for HCC therapy targeting TAMs. Future investigations are warranted to further explore the effects of IRAK1, NLRP3, and TAMs on HCC development.
Acknowledgments
Funding: This study was supported by the Guangzhou Science and Technology Project (No.202102080618).
Footnote
Reporting Checklist: The authors have completed the MDAR reporting checklist. Available at https://jgo.amegroups.com/article/view/10.21037/jgo-22-471/rc
Data Sharing Statement: Available at https://jgo.amegroups.com/article/view/10.21037/jgo-22-471/dss
Conflicts of Interest: All authors have completed the ICMJE uniform disclosure form (available at https://jgo.amegroups.com/article/view/10.21037/jgo-22-471/coif). The authors have no conflicts of interest to declare.
Ethical Statement: The authors are accountable for all aspects of the work in ensuring that questions related to the accuracy or integrity of any part of the work are appropriately investigated and resolved. The study was conducted in accordance with the Declaration of Helsinki (as revised in 2013). The study was approved by institutional ethics board of the People’s Hospital of Huadu District (No. 2020043) and informed consent was obtained from all the participants.
Open Access Statement: This is an Open Access article distributed in accordance with the Creative Commons Attribution-NonCommercial-NoDerivs 4.0 International License (CC BY-NC-ND 4.0), which permits the non-commercial replication and distribution of the article with the strict proviso that no changes or edits are made and the original work is properly cited (including links to both the formal publication through the relevant DOI and the license). See: https://creativecommons.org/licenses/by-nc-nd/4.0/.
References
- Sukowati CHC. Heterogeneity of Hepatic Cancer Stem Cells. In: Birbrair A. editor. Stem Cells Heterogeneity in Cancer. Cham: Springer International Publishing; 2019:59-81.
- Siegel RL, Miller KD, Fuchs HE, et al. Cancer statistics, 2022. CA Cancer J Clin 2022;72:7-33. [Crossref] [PubMed]
- Chen Y, Song Y, Du W, et al. Tumor-associated macrophages: an accomplice in solid tumor progression. J Biomed Sci 2019;26:78. [Crossref] [PubMed]
- Wang J, Li D, Cang H, et al. Crosstalk between cancer and immune cells: Role of tumor-associated macrophages in the tumor microenvironment. Cancer Med 2019;8:4709-21. [Crossref] [PubMed]
- Noy R, Pollard JW. Tumor-associated macrophages: from mechanisms to therapy. Immunity 2014;41:49-61. [Crossref] [PubMed]
- Shirabe K, Mano Y, Muto J, et al. Role of tumor-associated macrophages in the progression of hepatocellular carcinoma. Surg Today 2012;42:1-7. [Crossref] [PubMed]
- Capece D, Fischietti M, Verzella D, et al. The inflammatory microenvironment in hepatocellular carcinoma: a pivotal role for tumor-associated macrophages. Biomed Res Int 2013;2013:187204. [Crossref] [PubMed]
- Narayanan KB, Park HH. Toll/interleukin-1 receptor (TIR) domain-mediated cellular signaling pathways. Apoptosis 2015;20:196-209. [Crossref] [PubMed]
- Gottipati S, Rao NL, Fung-Leung WP. IRAK1: a critical signaling mediator of innate immunity. Cell Signal 2008;20:269-76. [Crossref] [PubMed]
- Rhyasen GW, Starczynowski DT. IRAK signalling in cancer. Br J Cancer 2015;112:232-7. [Crossref] [PubMed]
- Ye ZH, Gao L, Wen DY, et al. Diagnostic and prognostic roles of IRAK1 in hepatocellular carcinoma tissues: an analysis of immunohistochemistry and RNA-sequencing data from the cancer genome atlas. Onco Targets Ther 2017;10:1711-23. [Crossref] [PubMed]
- Cheng BY, Lau EY, Leung HW, et al. IRAK1 Augments Cancer Stemness and Drug Resistance via the AP-1/AKR1B10 Signaling Cascade in Hepatocellular Carcinoma. Cancer Res 2018;78:2332-42. [Crossref] [PubMed]
- Tschopp J, Schroder K. NLRP3 inflammasome activation: The convergence of multiple signalling pathways on ROS production? Nat Rev Immunol 2010;10:210-5. [Crossref] [PubMed]
- Sharma BR, Kanneganti TD. NLRP3 inflammasome in cancer and metabolic diseases. Nat Immunol 2021;22:550-9. [Crossref] [PubMed]
- Moossavi M, Parsamanesh N, Bahrami A, et al. Role of the NLRP3 inflammasome in cancer. Mol Cancer 2018;17:158. [Crossref] [PubMed]
- Liu H, Wang M, Jin Z, et al. FNDC5 induces M2 macrophage polarization and promotes hepatocellular carcinoma cell growth by affecting the PPARγ/NF-κB/NLRP3 pathway. Biochem Biophys Res Commun 2021;582:77-85. [Crossref] [PubMed]
- Chen W, Wei T, Chen Y, et al. Downregulation of IRAK1 Prevents the Malignant Behavior of Hepatocellular Carcinoma Cells by Blocking Activation of the MAPKs/NLRP3/IL-1β Pathway. Onco Targets Ther 2020;13:12787-96. [Crossref] [PubMed]
- Hashimoto O, Yoshida M, Koma Y, et al. Collaboration of cancer-associated fibroblasts and tumour-associated macrophages for neuroblastoma development. J Pathol 2016;240:211-23. [Crossref] [PubMed]
- Li R, Yin F, Guo YY, et al. Knockdown of ANRIL aggravates H2O2-induced injury in PC-12 cells by targeting microRNA-125a. Biomed Pharmacother 2017;92:952-61. [Crossref] [PubMed]
- Arvanitakis K, Koletsa T, Mitroulis I, et al. Tumor-Associated Macrophages in Hepatocellular Carcinoma Pathogenesis, Prognosis and Therapy. Cancers (Basel) 2022;14:226. [Crossref] [PubMed]
- Murata M. Inflammation and cancer. Environ Health Prev Med 2018;23:50. [Crossref] [PubMed]
- Singh D, Singh M, Yadav E, et al. Amelioration of diethylnitrosamine (DEN)-induced hepatocellular carcinogenesis in animal models via knockdown oxidative stress and proinflammatory markers by Madhuca longifolia embedded silver nanoparticles. RSC Adv 2018;8:6940-53. [Crossref] [PubMed]
- Vasudevan S, Venkataraman A, Research D. Liposome Encapsulated Astaxanthin Altered Biochemical Profile in Diethylnitrosamine (DEN) Induced Hepato Carcinoma on Swiss Albino Mice. International Journal of Pharmaceutical Sciences and Drug Research 2020;12:344-52. [Crossref]
- Yu LX, Ling Y, Wang HY. Role of nonresolving inflammation in hepatocellular carcinoma development and progression. NPJ Precis Oncol 2018;2:6. [Crossref] [PubMed]
- Li N, Jiang J, Fu J, et al. Targeting interleukin-1 receptor-associated kinase 1 for human hepatocellular carcinoma. J Exp Clin Cancer Res 2016;35:140. [Crossref] [PubMed]
- Gao ZH, Li RF, Lv XZ, et al. Saikosaponin-b2 inhibits tumor growth and inflammation of hepatocellular carcinoma by regulating STK4 / IRAK1 / NF-κB signaling in H22 tumor-bearing mice. FASEB J 2019;33:816.7.
- Lin TY, Tsai MC, Tu W, et al. Role of the NLRP3 Inflammasome: Insights Into Cancer Hallmarks. Front Immunol 2020;11:610492. [Crossref] [PubMed]
- Wei Q, Zhu R, Zhu J, et al. E2-Induced Activation of the NLRP3 Inflammasome Triggers Pyroptosis and Inhibits Autophagy in HCC Cells. Oncol Res 2019;27:827-34. [Crossref] [PubMed]
- He Y, Hara H, Núñez G. Mechanism and Regulation of NLRP3 Inflammasome Activation. Trends Biochem Sci 2016;41:1012-21. [Crossref] [PubMed]
- Mezzasoma L, Antognelli C, Talesa VN. Atrial natriuretic peptide down-regulates LPS/ATP-mediated IL-1β release by inhibiting NF-kB, NLRP3 inflammasome and caspase-1 activation in THP-1 cells. Immunol Res 2016;64:303-12. [Crossref] [PubMed]
- Antonopoulos C, Russo HM, El Sanadi C, et al. Caspase-8 as an Effector and Regulator of NLRP3 Inflammasome Signaling. J Biol Chem 2015;290:20167-84. [Crossref] [PubMed]
- Hamarsheh S, Zeiser R. NLRP3 Inflammasome Activation in Cancer: A Double-Edged Sword. Front Immunol 2020;11:1444. [Crossref] [PubMed]
- Fan SH, Wang YY, Lu J, et al. Luteoloside suppresses proliferation and metastasis of hepatocellular carcinoma cells by inhibition of NLRP3 inflammasome. PLoS One 2014;9:e89961. [Crossref] [PubMed]
- Wei Q, Guo P, Mu K, et al. Estrogen suppresses hepatocellular carcinoma cells through ERβ-mediated upregulation of the NLRP3 inflammasome. Lab Invest 2015;95:804-16. [Crossref] [PubMed]
- Fernandes-Alnemri T, Kang S, Anderson C, et al. Cutting edge: TLR signaling licenses IRAK1 for rapid activation of the NLRP3 inflammasome. J Immunol 2013;191:3995-9. [Crossref] [PubMed]
- Fu XT, Song K, Zhou J, et al. Tumor-associated macrophages modulate resistance to oxaliplatin via inducing autophagy in hepatocellular carcinoma. Cancer Cell Int 2019;19:71. [Crossref] [PubMed]
- Wan S, Zhao E, Kryczek I, et al. Tumor-associated macrophages produce interleukin 6 and signal via STAT3 to promote expansion of human hepatocellular carcinoma stem cells. Gastroenterology 2014;147:1393-404. [Crossref] [PubMed]
- Ao JY, Zhu XD, Chai ZT, et al. Colony-Stimulating Factor 1 Receptor Blockade Inhibits Tumor Growth by Altering the Polarization of Tumor-Associated Macrophages in Hepatocellular Carcinoma. Mol Cancer Ther 2017;16:1544-54. [Crossref] [PubMed]
- Wang Y, Ma H, Li Y, et al. MiR-192-5p-Modified Tumor-Associated Macrophages-Derived Exosome Suppressed Endometrial Cancer Progression Through Targeting IRAK1/NF-κB Signaling. Reprod Sci 2022;29:436-47. [Crossref] [PubMed]
- Hofbauer D, Mougiakakos D, Broggini L, et al. β2-microglobulin triggers NLRP3 inflammasome activation in tumor-associated macrophages to promote multiple myeloma progression. Immunity 2021;54:1772-1787.e9. [Crossref] [PubMed]
- Tiainen S, Tumelius R, Rilla K, et al. High numbers of macrophages, especially M2-like (CD163-positive), correlate with hyaluronan accumulation and poor outcome in breast cancer. Histopathology 2015;66:873-83. [Crossref] [PubMed]
- Aras S, Zaidi MR. TAMeless traitors: macrophages in cancer progression and metastasis. Br J Cancer 2017;117:1583-91. [Crossref] [PubMed]
(English Language Editor: J. Teoh)