Knockdown of Obg-like ATPase 1 enhances sorafenib sensitivity by inhibition of GSK-3β/β-catenin signaling in hepatocellular carcinoma cells
Introduction
Hepatocellular carcinoma (HCC) represented the third leading cause of cancer worldwide in 2020, with approximately 830,000 deaths (1). Curative surgery is the most effective treatment for HCC patients who are diagnosed at an early stage, while those at the advanced stages are not eligible. The most common treatment for advanced HCC patients is sorafenib (2-4). However, limited effectiveness and drug resistance has restricted the clinical use of sorafenib in advanced HCC (5). Overcoming sorafenib resistance will bring benefits to advanced HCC patients.
The Wnt/β-catenin signaling pathway plays an essential role in cancer progression through initiating cell proliferation, migration, invasion, metastasis, stemness, and drug resistance (6,7). Activation of the Wnt/β-catenin pathway occurs in various tumors, including those of liver and intestinal cell origin (8,9). β-catenin is the core component of this pathway, and the multiprotein β-catenin degradation complex tightly controls its accumulation and nuclear translocation. Cytoplasmic β-catenin is phosphorylated by 2 Ser/Thr kinases, namely glycogen synthase kinase 3 (GSK-3) and casein kinase 1 (CK1), and is then recognized by ubiquitin ligase β-transducin repeat-containing protein (β-TrCP), which mediates β-catenin proteasomal degradation (10). Disruption of the degradation complex can prevent β-catenin degradation, resulting in accumulated β-catenin translocating to the nucleus. β-catenin, which is located in the nucleus, interacts with transcription factors of the lymphoid enhancer factor/T-cell factor (LEF/TCF) family and initiates the transcription of several protooncogenes, such as c-Myc and cyclin D1 (8,9,11). Accumulating evidence has demonstrated that aberrant activation of Wnt/β-catenin contributes to sorafenib resistance, and inhibition of Wnt/β-catenin eliminates sorafenib resistance and enhances sorafenib sensitivity (12-14). For this reason, identification of the regulator of Wnt/β-catenin involved in sorafenib resistance and targeting this regulator will be useful for improving the clinical outcome of sorafenib treatment.
Obg-like ATPase 1 (OLA1), an identified member of the Obg family and the YchF subfamily of p-loop GTPases, is affiliated with the translation-factor-related (TRAFAC) class and is highly conserved from bacteria to humans (15,16). P-loop GTPases are involved in monitoring various cellular processes, such as intracellular transport, signal transduction, protein translation, stress responses, and cell proliferation (17-20). OLA1 also works as an intrinsic stress response regulator, including oxidative stress (21) and heat shock (22,23). OLA1 was shown to be highly expressed in most cancers, lowly expressed under DNA damage, and reduces doxorubicin sensitivity in colon cancer cells (24). More evidence showed that reduction of OLA1 suppressed breast cancer cell migration and invasion, and was involved in epithelial-mesenchymal transition (EMT) in different tumor cells (25,26). OLA1 could be a potential biomarker of endometrial cancer (27). A recent study showed that OLA1 was also overexpressed in HCC and OLA1 promoted HCC development (28). However, there is no study showing whether OLA1 is related to HCC sorafenib resistance. A previous study reported that OLA1 can bind to GSK-3β and phosphorylate GSK-3β at Ser9, which is an inactivate form, failing to phosphorylate β-catenin and resulting in β-catenin accumulation (26). Previous works showed that β-catenin activation contributed to chemoresistance and inhibition of β-catenin signaling enhanced by sorafenib sensitivity (29,30). The phosphorylation of β-catenin at Thr41, Ser37, and Ser33 by GSK-3β was reported to promote β-catenin degradation, prevent β-catenin nuclear translocation, and inhibit β-catenin transactivation (31). OLA1 phosphorylated GSK-3β at Ser9 and blocked its activation (26,32). Evidence also showed that GSK-3β/β-catenin contributed to sorafenib resistance, blocking GSK-3β/β-catenin signaling to overcome sorafenib resistance and enhance sorafenib sensitivity (10,33). Therefore, we hypothesized that OLA1 may contribute to sorafenib resistance in HCC by activation of GSK-3β/β-catenin signaling.
In the current work, we showed that OLA1 was positively correlated with sorafenib resistance by activating the GSK-3β/β-catenin signaling pathway in HCC. Our data suggests that targeting OLA1 can improve the sensitivity of sorafenib in HCC. We present the following article in accordance with the MDAR reporting checklist (available at https://jgo.amegroups.com/article/view/10.21037/jgo-22-458/rc).
Methods
Reagents and antibodies
The chemicals sorafenib, crystal violet, propidium iodide (PI), and protease inhibitor cocktail were obtained from MedChemExpress (MCE, Shanghai, China). The antibodies used in this study are listed as follows: OLA1 [Abclonal; Wuhan, China; Cat#A11671; 1:1,000 for western blot (WB)], phospho-GSK-3β-S9 (Abclonal; Cat#AP1088; 1:1,000 for WB), GSK-3β (Abclonal; Cat#A6164; 1:1,000 for WB), Cyclin D1 (Abcam, Waltham, MA, USA; Cat#ab134175; 1:1,000 for WB), β-catenin (Cell Signaling Technology, Danvers, MA, USA; Cat#8480; 1:1,000 for WB), c-Myc (Cell Signaling Technology; Cat#18583; 1:1,000 for WB), cleaved PARP Cell Signaling Technology; Cat#5625; 1:1,000 for WB), cleaved caspase-3 (Cell Signaling Technology; Cat#9664; 1:1,000 for WB), and β-actin (Cell Signaling Technology; Cat#3700; 1:2,000 for WB).
Clinical survival analysis of sorafenib treated patients
The survival rates of HCC patients who received sorafenib treatment were analyzed by Kaplan-Meier plotter (https://kmplot.com/analysis/index.php?p=service&cancer=liver_rnaseq) (34). OLA1 low or high expression group was divided by the best separation of survival.
Cell lines
HCC cells Huh7 and HCCLM3, along with HEK293T cells, were obtained from the Cell Bank of Type Culture Collection of the Chinese Academy of Sciences (Shanghai, China), and maintained in Dulbecco’s Modified Eagle Medium (DMEM) (Hyclone, Cat#SH30243.01) supplemented with 10% fetal bovine serum (Hyclone, Cat#SV30087.03) and 1% penicillin-streptomycin (MCE; Cat#HY-K1006) at 37 ℃ with 5% CO2.
Establishment of stable OLA1-knockdown cell lines
Two specific sequences targeting OLA1 were inserted into the pLKO.1 vector to generate OLA1 short hairpin RNA (shRNA) plasmids: shOLA1-1, 5'-GCCTTGGAACTCAAGTTGCAA-3'; shOLA1-2, 5'-CCAGATGAAAGGTTTGACTTT-3'. The lentivirus constructs and packaged plasmids (pMDL, VSV-G, and REV) were transfected into HEK293T cells to produce lentivirus using HighGene Transfection reagent (Abclonal; Cat#RM09014) according to the manufacturer’s instructions. At 48–72 h after transfection, lentiviruses were collected and then contaminating cells were removed by centrifugation for 30 min at 2,500 rpm. Huh7 and HCCLM3 cells were transfected with the virus plus 10 µg/mL of polybrene (MCE; Cat#HY-112735). Stable OLA1-knockdown cell lines were selected by puromycin (1 µg/mL). WB was used to confirm the knockdown efficiency.
Real-time quantitative polymerase chain reaction (RT-qPCR)
TRIzol (Invitrogen) was used to isolate total RNA and gDNA Erase and PrimeScript RT reagent kits (TAKARA Biotechnology, Dalian, China) were used to synthesize cDNA with 2 µg of total RNA following the manufacturer’s instructions. SYBR Green PCR master mix kit was used to quantify mRNA levels. β-actin was used as an internal control gene. The specific primer sequences were as follows: c-Myc: forward, 5'-GGCTCCTGGCAAAAGGTCA-3', reverse, 5'-CTGCGTAGTTGTGCTGATGT-3'; cyclin D1: forward, 5'-GCTGCGAAGTGGAAACCATC-3', reverse, 5'-CCTCCTTCTGCACACATTTGAA-3'; β-actin: forward, 5'-AGCGAGCATCCCCCAAAGTT-3', reverse, 5'-GGGCACGAAGGCTCATCATT-3'.
WB
Protein expression was measured by the WB assay. Briefly, cells were treated with sorafenib for 24 h, washed with cooled phosphate-buffered saline (PBS), and lysed in RIPA buffer with protease inhibitor cocktail. The BCA assay was used to determine the total protein concentration (Pierce, Rockford, IL, USA), and SDS-PAGE gel was used to separate each sample (20–50 µg total proteins). Proteins were transferred to PVDF membranes (Millipore, Bedford, MA, USA), incubated with appropriate primary antibodies overnight at 4 ℃, and then incubated with corresponding horseradish peroxidase (HRP)-conjugated secondary antibodies. The enhanced chemiluminescence (ECL) WB kit (Pierce) was used to visualize the primary and second antibody complexes.
Cell counting kit-8 (CCK)-8 assay
Cell viability was measured by the CCK-8 assay. Briefly, 5,000 control and OLA1 knockdown Huh7 and HCCLM3 cells were seeded into 96-well plates overnight, then the medium was changed with or without sorafenib (10 µM). After 24 h incubation, CCK-8 (10 µL) solution was added into each well and maintained for 1 h. Optical density (OD) values were measured at 450 nm wavelength.
Clone formation assay
A total of 500 control and OLA1 knockdown Huh7 and HCCLM3 cells were seeded into 6-well plates overnight, and the medium was changed with or without sorafenib (10 µM) lasting for 2 weeks. The sorafenib incubation medium was renewed every other day. At the end of treatment, 0.1% crystal violet was used to stain the cells, and then the cell numbers were counted.
Cell death count
Control and OLA1 knockdown Huh7 and HCCLM3 cells were seeded into 6-well plates overnight, and the medium was changed with or without sorafenib (10 µM) for 24 h. After treatment, cells were harvested, and then PI and trypan blue staining were used to determine cell death. For PI staining, cells were incubated in PBS with PI at 1 µg/mL, and flow cytometry was used to detect dead cells. For trypan blue staining, 20 µL of cells were incubated with 20 µL of 0.4% trypan blue for 5 min, and then dead cells were counted under a microscope.
Statistical analysis
Mean ± standard deviation (SD) was used to present the results of 3 or more independent experiments. Student’s t-test, two-way analysis of variance (ANOVA), and Pearson r were used to perform statistical analyses with GraphPad Prism 8 (GraphPad Software, San Diego, CA, USA), and P<0.05 was considered statistically significant.
Results
OLA1 was positively correlated with clinical sorafenib resistance in HCC
Owing to the serious side effects, the use of sorafenib to treat HCC has been limited. Previous work reported that OLA1 was overexpressed and promoted tumorigenesis in HCC (28). To clarify whether OLA1 contributes to sorafenib resistance in HCC, we analyzed the survival of HCC patients treated with sorafenib according to different expression profiles of OLA1 in a public database (https://kmplot.com/analysis/index.php?p=service&cancer=liver_rnaseq) (35). The survival plot exhibited that patients who expressed high levels of OLA1 had a shorter overall survival (OS) (Figure 1A), recurrence-free survival (RFS) (Figure 1B), and disease-specific survival (DSS) (Figure 1C) than those who expressed low levels of OLA1. These results indicated that OLA1 may promote sorafenib resistance in HCC.
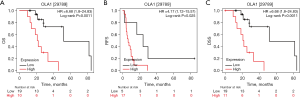
Knockdown of OLA1 enhanced cell proliferation inhibition induced by sorafenib
To evaluate the effects of OLA1 on sorafenib treated HCC cells, OLA1 knockdown Huh7 and HCCLM3 HCC cell lines were established and then stimulated with sorafenib (10 µM) for 24 h. Cell viabilities were detected by the CCK-8 assay. As shown in Figure 2A,2B, OLA1 knockdown enhanced HCC cancer cell proliferation inhibition induced by sorafenib in Huh7 (Figure 2A) and HCCLM3 cells (Figure 2B). In addition, the clone formation abilities were analyzed by the clone formation assay. The results showed that OLA1 knockdown cells formed markedly fewer clones compared with control cells both with or without sorafenib treatment in Huh7 (Figure 2C) and HCCLM3 cells (Figure 2D). These results suggested that reduction of OLA1 augmented HCC cell proliferation inhibition induced by sorafenib.
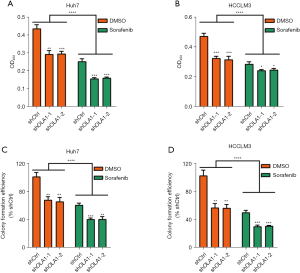
Knockdown of OLA1 enhanced HCC cell death induced by sorafenib
As cell death can also be induced by sorafenib, we determined whether OLA1 contributed to HCC cell death induced by sorafenib (36,37). OLA1 knockdown and control Huh7 and HCCLM3 cells were incubated with sorafenib (10 µM) for 24 h. PI and trypan blue staining were used to measure cell death. The results of PI staining showed that both with or without sorafenib treatment, knockdown of OLA1 markedly increased the number of PI positive Huh7 (Figure 3A) and HCCLM3 cells (Figure 3B). Next, we also used trypan blue staining to confirm our PI staining results. As expected, the results from trypan blue staining similarly demonstrated that OLA1 knockdown significantly enhanced HCC cell death induced by sorafenib in Huh7 (Figure 3C) and HCCLM3 cells (Figure 3D). These results showed that OLA1 knockdown augmented HCC cell death induced by sorafenib.
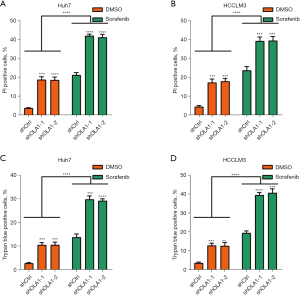
Knockdown of OLA1 augmented the reduction of proliferation-related protein expression and increased apoptosis-associated protein expression induced by sorafenib
To evaluate the mechanism of OLA1 knockdown in enhancing HCC cell proliferation inhibition and cell death induced by sorafenib, we determined the expression of proliferation- and apoptosis-associated proteins by WB. OLA1 knockdown and control Huh7 and HCCLM3 cells were incubated with sorafenib (10 µM) for 24 h. First, the expression of proliferation-associated proteins, such as c-Myc and cyclin D1, was measured. Consistent with the previous results, OLA1 knockdown enhanced cell proliferation inhibition, while knockdown of OLA1 significantly decreased c-Myc and cyclin D1 expression in Huh7 (Figure 4A) and HCCLM3 cells (Figure 4B). Furthermore, apoptosis-associated proteins were also analyzed. As shown in Figure 4C,4D, both with or without sorafenib treatment, OLA1 knockdown enhanced the expression of cleaved PARP and cleaved caspase-3 in Huh7 (Figure 4C) and HCCLM3 cells (Figure 4D). These data demonstrated that OLA1 knockdown augmented the sorafenib-induced reduction of proliferation-related proteins and increase of apoptosis-associated proteins.
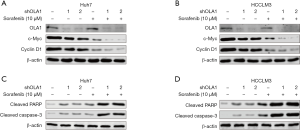
Knockdown of OLA1 enhanced the inhibition of GSK-3β/β-catenin induced by sorafenib
Evidences have demonstrated that GSK-3β/β-catenin signaling contributes to sorafenib resistance. First, we detected the function of OLA1 in GSK-3β/β-catenin signaling. Lithium chloride (LiCl), a GSK3β inhibitor, was used to induce GSK3β/β-catenin activation. As expected, GSK3β/β-catenin signaling was reduced in OLA1 downregulated Huh7 (Figure S1A) and HCCLM3 (Figure S1B) cells. Then, we tested whether knockdown of OLA1 enhanced sorafenib sensitivity through suppressing GSK-3β/β-catenin signaling. Control and OLA1 knockdown Huh7 and HCCLM3 cells were treated with sorafenib for 24 h, and then GSK-3β/β-catenin signaling was analyzed. The results showed that knockdown of OLA1 significantly reduced the phosphorylation of GSK-3β at Ser9 and the expression of β-catenin both with or without sorafenib treatment compared with controls in Huh7 (Figure 5A) and HCCLM3 (Figure 5B) cells. In addition, the mRNA levels of β-catenin downstream genes, such as c-Myc and cyclin D1, were also detected. As expected, OLA1 knockdown reduced the mRNA levels of c-Myc and cyclin D1 in Huh7 (Figure 5C) and HCCLM3 (Figure 5D) cells. Taken together, these results indicated that knockdown of OLA1 suppressed GSK-3β/β-catenin signaling upon sorafenib treatment.
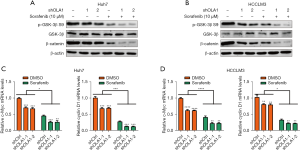
Discussion
In the current work, we proved that OLA1 was a positive regulator of sorafenib resistance (Figure 6). OLA1 knockdown increased cell proliferation inhibition and cell death induced by sorafenib (Figures 2,3). Moreover, we indicated that knockdown of OLA1 suppressed GSK-3β/β-catenin signaling activation (Figure 5).
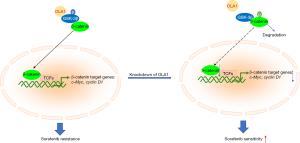
Accumulating reports have shown that OLA1 is highly expressed in most cancers, such as HCC and breast cancer (28,38,39). Given the essential functions of OLA1 in stress responses, it is reasonable to suggest that OLA1 may be a potential therapeutic target for cancer. A key hallmark of cancer cells is the de novo or acquired resistance to diverse stresses such as drug treatment, heat shock, and hypoxia (21,22,38). HIF1α activation-induced by hypoxic conditions contributed sorafenib resistance in HCC, inhibition of HIF1α attenuated sorafenib resistance (40,41). Liu et al. (42) reported that OLA1 induced HIF1α activation in colorectal cancer. So HIF1α activation may also involve in OLA1-induced sorafenib resistance. To evaluate the functional role of OLA1 in sorafenib resistance, we determined the impact of loss-of-function of OLA1 on sorafenib-induced cell proliferation inhibition and cell death. Our data indicated that knockdown of OLA1 enhanced sorafenib sensitivity in HCC, with increased cell proliferation inhibition and cell death. Previous studies have shown that OLA1 mediates the phosphorylation of GSK-3β at Ser9 (26,32,43). β-catenin is phosphorylated by GSK-3β at Ser33, Ser37, and Thr41 (31), and then the phosphorylated β-catenin is specifically recognized by E3 ubiquitin ligase β-TrCP and broken down by the proteasome system (44,45). In the present work, we discovered that sorafenib reduced the phosphorylation of GSK-3β at Ser9 in both Huh7 and HCCLM3 HCC cells. Concurrently, we also observed a decrease of β-catenin, thus preventing β-catenin nuclear translocation, where it is involved in LEF/TCF mediated transcription (8). c-Myc and Cyclin D1, which are the major targets of β-catenin, were also reduced in OLA1 knockdown cells. OLA1 knockdown could enhance the changes induced by sorafenib, indicating that OLA1 is involved in sorafenib resistance through GSK-3β/β-catenin signaling.
In summary, we proved that OLA1 contributed to sorafenib resistance by reducing cell proliferation inhibition and cell death induced by sorafenib. Furthermore, GSK-3β/β-catenin signaling, which augmented sorafenib resistance, was suppressed in OLA1 knockdown HCC cells. Overall, OLA1 enhanced sorafenib resistance, and targeting OLA1 may overcome sorafenib resistance in HCC.
Acknowledgments
Funding: This study was supported by grants from Shaoxing City Program of Science and Technology Program Funding (No. 2018C30144), Zhejiang Provincial Program of Medical and Health Science Funding (No. 2019PY016), and Zhejiang Provincial Public Welfare Technology Research Project (No. LGF20H30001).
Footnote
Reporting Checklist: The authors have completed the MDAR reporting checklist. Available at https://jgo.amegroups.com/article/view/10.21037/jgo-22-458/rc
Data Sharing Statement: Available at https://jgo.amegroups.com/article/view/10.21037/jgo-22-458/dss
Conflicts of Interest: All authors have completed the ICMJE uniform disclosure form (available at https://jgo.amegroups.com/article/view/10.21037/jgo-22-458/coif). The authors have no conflicts of interest to declare.
Ethical Statement: The authors are accountable for all aspects of the work in ensuring that questions related to the accuracy or integrity of any part of the work are appropriately investigated and resolved.
Open Access Statement: This is an Open Access article distributed in accordance with the Creative Commons Attribution-NonCommercial-NoDerivs 4.0 International License (CC BY-NC-ND 4.0), which permits the non-commercial replication and distribution of the article with the strict proviso that no changes or edits are made and the original work is properly cited (including links to both the formal publication through the relevant DOI and the license). See: https://creativecommons.org/licenses/by-nc-nd/4.0/.
References
- Sung H, Ferlay J, Siegel RL, et al. Global Cancer Statistics 2020: GLOBOCAN Estimates of Incidence and Mortality Worldwide for 36 Cancers in 185 Countries. CA Cancer J Clin 2021;71:209-49. [Crossref] [PubMed]
- Zhu YJ, Zheng B, Wang HY, et al. New knowledge of the mechanisms of sorafenib resistance in liver cancer. Acta Pharmacol Sin 2017;38:614-22. [Crossref] [PubMed]
- Tang W, Chen Z, Zhang W, et al. The mechanisms of sorafenib resistance in hepatocellular carcinoma: theoretical basis and therapeutic aspects. Signal Transduct Target Ther 2020;5:87. [Crossref] [PubMed]
- Wang M, Wang Z, Zhi X, et al. SOX9 enhances sorafenib resistance through upregulating ABCG2 expression in hepatocellular carcinoma. Biomed Pharmacother 2020;129:110315. [Crossref] [PubMed]
- Spinzi G, Paggi S. Sorafenib in advanced hepatocellular carcinoma. N Engl J Med 2008;359:2497-8; author reply 2498-9. [Crossref] [PubMed]
- Wang W, Smits R, Hao H, et al. Wnt/β-Catenin Signaling in Liver Cancers. Cancers (Basel) 2019;11:926. [Crossref] [PubMed]
- Carotenuto P, Fassan M, Pandolfo R, et al. Wnt signalling modulates transcribed-ultraconserved regions in hepatobiliary cancers. Gut 2017;66:1268-77. [Crossref] [PubMed]
- MacDonald BT, Tamai K, He X. Wnt/beta-catenin signaling: components, mechanisms, and diseases. Dev Cell 2009;17:9-26. [Crossref] [PubMed]
- Clevers H. Wnt/beta-catenin signaling in development and disease. Cell 2006;127:469-80. [Crossref] [PubMed]
- Liu Y, Zhuang H, Cao F, et al. Shc3 promotes hepatocellular carcinoma stemness and drug resistance by interacting with β-catenin to inhibit its ubiquitin degradation pathway. Cell Death Dis 2021;12:278. [Crossref] [PubMed]
- Valenta T, Hausmann G, Basler K. The many faces and functions of β-catenin. EMBO J 2012;31:2714-36. [Crossref] [PubMed]
- Lin HH, Feng WC, Lu LC, et al. Inhibition of the Wnt/β-catenin signaling pathway improves the anti-tumor effects of sorafenib against hepatocellular carcinoma. Cancer Lett 2016;381:58-66. [Crossref] [PubMed]
- Liu Y, Ye X, Zhang JB, et al. PROX1 promotes hepatocellular carcinoma proliferation and sorafenib resistance by enhancing β-catenin expression and nuclear translocation. Oncogene 2015;34:5524-35. [Crossref] [PubMed]
- Huang M, Chen C, Geng J, et al. Targeting KDM1A attenuates Wnt/β-catenin signaling pathway to eliminate sorafenib-resistant stem-like cells in hepatocellular carcinoma. Cancer Lett 2017;398:12-21. [Crossref] [PubMed]
- Gradia DF, Rau K, Umaki AC, et al. Characterization of a novel Obg-like ATPase in the protozoan Trypanosoma cruzi. Int J Parasitol 2009;39:49-58. [Crossref] [PubMed]
- Koller-Eichhorn R, Marquardt T, Gail R, et al. Human OLA1 defines an ATPase subfamily in the Obg family of GTP-binding proteins. J Biol Chem 2007;282:19928-37. [Crossref] [PubMed]
- Verstraeten N, Fauvart M, Versées W, et al. The universally conserved prokaryotic GTPases. Microbiol Mol Biol Rev 2011;75:507-42. second and third pages of table of contents. [Crossref] [PubMed]
- Leipe DD, Wolf YI, Koonin EV, et al. Classification and evolution of P-loop GTPases and related ATPases. J Mol Biol 2002;317:41-72. [Crossref] [PubMed]
- Chen T, Yeh HW, Chen PP, et al. BARD1 is an ATPase activating protein for OLA1. Biochim Biophys Acta Gen Subj 2022;1866:130099. [Crossref] [PubMed]
- Landwehr V, Milanov M, Hong J, et al. The Role of the Universally Conserved ATPase YchF/Ola1 in Translation Regulation during Cellular Stress. Microorganisms 2021;10:14. [Crossref] [PubMed]
- Zhang J, Rubio V, Lieberman MW, et al. OLA1, an Obg-like ATPase, suppresses antioxidant response via nontranscriptional mechanisms. Proc Natl Acad Sci U S A 2009;106:15356-61. [Crossref] [PubMed]
- Mao RF, Rubio V, Chen H, et al. OLA1 protects cells in heat shock by stabilizing HSP70. Cell Death Dis 2013;4:e491. [Crossref] [PubMed]
- Dannenmaier S, Desroches Altamirano C, Schüler L, et al. Quantitative proteomics identifies the universally conserved ATPase Ola1p as a positive regulator of heat shock response in Saccharomyces cerevisiae. J Biol Chem 2021;297:101050. [Crossref] [PubMed]
- Sun H, Luo X, Montalbano J, et al. DOC45, a novel DNA damage-regulated nucleocytoplasmic ATPase that is overexpressed in multiple human malignancies. Mol Cancer Res 2010;8:57-66. [Crossref] [PubMed]
- Zhang JW, Rubio V, Zheng S, et al. Knockdown of OLA1, a regulator of oxidative stress response, inhibits motility and invasion of breast cancer cells. J Zhejiang Univ Sci B 2009;10:796-804. [Crossref] [PubMed]
- Bai L, Yu Z, Zhang J, et al. OLA1 contributes to epithelial-mesenchymal transition in lung cancer by modulating the GSK3β/snail/E-cadherin signaling. Oncotarget 2016;7:10402-13. [Crossref] [PubMed]
- Dong Y, Yin A, Xu C, et al. OLA1 is a potential prognostic molecular biomarker for endometrial cancer and promotes tumor progression. Oncol Lett 2021;22:576. [Crossref] [PubMed]
- Huang S, Zhang C, Sun C, et al. Obg-like ATPase 1 (OLA1) overexpression predicts poor prognosis and promotes tumor progression by regulating P21/CDK2 in hepatocellular carcinoma. Aging (Albany NY) 2020;12:3025-41. [Crossref] [PubMed]
- Lin Z, Sun L, Xie S, et al. Chemotherapy-Induced Long Non-coding RNA 1 Promotes Metastasis and Chemo-Resistance of TSCC via the Wnt/β-Catenin Signaling Pathway. Mol Ther 2018;26:1494-508. [Crossref] [PubMed]
- Nagel C, Armeanu-Ebinger S, Dewerth A, et al. Anti-tumor activity of sorafenib in a model of a pediatric hepatocellular carcinoma. Exp Cell Res 2015;331:97-104. [Crossref] [PubMed]
- Kimelman D, Xu W. beta-catenin destruction complex: insights and questions from a structural perspective. Oncogene 2006;25:7482-91. [Crossref] [PubMed]
- Xu D, Song R, Wang G, et al. Obg-like ATPase 1 regulates global protein serine/threonine phosphorylation in cancer cells by suppressing the GSK3β-inhibitor 2-PP1 positive feedback loop. Oncotarget 2016;7:3427-39. [Crossref] [PubMed]
- Panka DJ, Cho DC, Atkins MB, et al. GSK-3beta inhibition enhances sorafenib-induced apoptosis in melanoma cell lines. J Biol Chem 2008;283:726-32. [Crossref] [PubMed]
- Nagy Á, Lánczky A, Menyhárt O, et al. Validation of miRNA prognostic power in hepatocellular carcinoma using expression data of independent datasets. Sci Rep 2018;8:9227. [Crossref] [PubMed]
- Menyhárt O, Nagy Á, Győrffy B. Determining consistent prognostic biomarkers of overall survival and vascular invasion in hepatocellular carcinoma. R Soc Open Sci 2018;5:181006. [Crossref] [PubMed]
- Coriat R, Nicco C, Chéreau C, et al. Sorafenib-induced hepatocellular carcinoma cell death depends on reactive oxygen species production in vitro and in vivo. Mol Cancer Ther 2012;11:2284-93. [Crossref] [PubMed]
- Rahmani M, Davis EM, Crabtree TR, et al. The kinase inhibitor sorafenib induces cell death through a process involving induction of endoplasmic reticulum stress. Mol Cell Biol 2007;27:5499-513. [Crossref] [PubMed]
- Liu J, Miao X, Xiao B, et al. Obg-Like ATPase 1 Enhances Chemoresistance of Breast Cancer via Activation of TGF-β/Smad Axis Cascades. Front Pharmacol 2020;11:666. [Crossref] [PubMed]
- Liu J, Yang Q, Xiao KC, et al. Obg-like ATPase 1 inhibited oral carcinoma cell metastasis through TGFβ/SMAD2 axis in vitro. BMC Mol Cell Biol 2020;21:65. [Crossref] [PubMed]
- Feng J, Dai W, Mao Y, et al. Simvastatin re-sensitizes hepatocellular carcinoma cells to sorafenib by inhibiting HIF-1α/PPAR-γ/PKM2-mediated glycolysis. J Exp Clin Cancer Res 2020;39:24. [Crossref] [PubMed]
- Chen J, Jin R, Zhao J, et al. Potential molecular, cellular and microenvironmental mechanism of sorafenib resistance in hepatocellular carcinoma. Cancer Lett 2015;367:1-11. [Crossref] [PubMed]
- Liu Y, Kong XX, He JJ, et al. OLA1 promotes colorectal cancer tumorigenesis by activation of HIF1α/CA9 axis. BMC Cancer 2022;22:424. [Crossref] [PubMed]
- Narasimhan G, Henderson J, Luong HT, et al. OBG-like ATPase 1 inhibition attenuates angiotensin II-induced hypertrophic response in human ventricular myocytes via GSK-3beta/beta-catenin signalling. Clin Exp Pharmacol Physiol 2019;46:743-51. [Crossref] [PubMed]
- Orford K, Crockett C, Jensen JP, et al. Serine phosphorylation-regulated ubiquitination and degradation of beta-catenin. J Biol Chem 1997;272:24735-8. [Crossref] [PubMed]
- Aberle H, Bauer A, Stappert J, et al. beta-catenin is a target for the ubiquitin-proteasome pathway. EMBO J 1997;16:3797-804. [Crossref] [PubMed]
(English Language Editor: C. Betlazar-Maseh)