Expression of CircATXN7 in esophageal cancer tissues and its effect on cell proliferation and invasion
Introduction
Esophageal cancer (EC) is the most frequently diagnosed clinical malignant tumor of the digestive tract and the 7th most frequently diagnosed cancer worldwide (1). In China, EC incidence and mortality continue to rise, threatening national health (2). Although EC-related diagnosis and treatment methods continue to improve, because of poor prognosis and a high degree of malignancy, the 5-year survival rates remain poor (3). To improve the treatment and survival rate of EC, it is necessary to find new therapeutic targets and biomarkers that have the potential to affect EC occurrence and development, providing a foundation for clinical diagnosis and treatment.
Circular RNAs (circRNAs) are produced via reverse splicing from covalently closed loops and exhibit great abundance, stability, conservation, and tissue specificity in eukaryotic cells (4). With the rapid advancement of high-throughput sequencing and bioinformatics, researchers have discovered that circRNAs perform a variety of biological functions, including involvement in the incidence and advance of various human diseases (5). Existing studies have shown that circRNAs participate in tumor biological behaviors at various levels, including transcriptional and posttranscriptional (6,7). Chen et al. identified circPVT1 and found that its expression was upregulated in tissues associated with gastric cancer (GC), suggesting that circPVT1 was a new proliferation factor that could serve as a prognostic marker for GC (8). Kong et al. showed that upregulation of circSMARCA5 in prostate cancer acted as an oncogene to facilitate the cell cycle and retard cell apoptosis (9). Therefore, the role of circRNAs in the incidence and progress of cancer can provide novel targets for cancer diagnosis and also new directions for related treatments. The study of circRNAs has become a new research direction, following the focus on long noncoding RNAs (lncRNAs) and microRNAs (miRNAs). This study investigated the biological function of circATXN7 in EC to further the development of a novel biomarker for EC. We present the following article in accordance with the MDAR reporting checklist (available at https://jgo.amegroups.com/article/view/10.21037/jgo-22-481/rc).
Methods
EC tissue specimens
Tissue specimens of EC and corresponding paracancerous tissue (≥5 cm from the tumor tissue edge, verified by biopsy) were obtained from 58 patients treated in Jiangsu Cancer Hospital between January 2019 and June 2019. The inclusion criteria were: (I) patients who met the criteria for the diagnosis of EC, confirmed by postoperative biopsy; (II) first-time diagnosed patients; and (III) no history of radiotherapy, chemotherapy, or esophageal surgery. The exclusion criteria were: (I) patients with Barrett's esophagitis or reflux esophagitis, (II) patients with primary malignant tumors in other areas of the body, (III) patients with decompensated liver or kidney functions, and (IV) patients with abnormal congenital immune deficiencies. The study participants included 47 male and 11 female patients, with an age range of 41−70 (57.96±6.12) years. The clinical characteristics of the patients are shown in Table 1. The study was conducted in accordance with the Declaration of Helsinki (as revised in 2013). The study was approved by the Medical Ethics Committee of Jiangsu Cancer Hospital (No. 2020 Section-011). Signed informed consent was obtained from the patients or their family members.
Table 1
Clinical characteristics | Patients (n=58) |
---|---|
Sex | |
Male | 47 |
Female | 11 |
Age, years | |
<60 | 17 |
≥60 | 41 |
Differentiation | |
Poorly-differentiated | 43 |
Well-differentiated | 15 |
Smoking | |
Yes | 35 |
No | 23 |
Depth of invasion | |
T1–T2 | 30 |
T3–T4a | 28 |
TNM staging | |
I–II | 26 |
III | 32 |
Lymph node metastasis | |
No | 34 |
Yes | 24 |
Cell culture
EC cell lines (Eca-109, KYSE-30, EC-9706, TE-1, and KYSE-150) and normal esophageal cell line (HET-1A) were purchased from Shanghai Cell Resource Center, Chinese Academy of Sciences and preserved in our laboratory. The cell culture medium consisted of 90% Dulbecco’s Modified Eagle Medium (DMEM; 4 mM glutamine and 1% penicillin-streptomycin) and 10% fetal bovine serum (FBS). Cells were cultured at 37 °C in a 5% carbon dioxide (CO2) environment. When the cell density reached 80–90%, cells were subcultured at a ratio of 1:2.
Quantitative real-time polymerase chain reaction (qRT-PCR)
Total RNA was extracted from tissue samples as follows. EC tissue and paracancerous tissue freshly obtained during surgery were transfered to −80 °C refrigeration for storage after quick freezing in liquid nitrogen. EC tissue and paracancerous normal tissue were then ground under liquid nitrogen. Total RNA was extracted from HET-1A, Eca-109, EC-9706, KYSE-150, KYSE-30, and TE-1 cells with TRIzol reagent, followed by quantification via UV spectrophotometer.
Total RNA was reverse-transcribed to complementary DNA (cDNA) according to the PrimeScript RT Reagent Kit instructions. The reverse transcription conditions were 42 and 70 °C for 60 and 5 minutes, respectively. Using cDNA as a template, PCR amplification was conducted according to the instructions of the SYBR Green PCR Master Mix Kit. The design and synthesis of all primer sequences were performed by Beijing Tiangen Biochemical Technology Co., Ltd. Primer sequence: circATXN7 upstream primer: 5'-CCTAGGGACAGAATTGGACGA-3', downstream primer: 5'-GCCCGCTCCGACATTCTT-3'. β-actin upstream primer: 5'-GAAATCGTGGACATTAA-3', downstream primer: 5'-AAGGAAGGCTGGAAGAGTG-3'. PCR amplification system totaled 20 µL: 10 µL 2×SYBR Master Mix, 2 µL cDNA template, 0.4 µL each upstream and downstream primers, and 7.2 µL distilled water. The reaction conditions were: 5 minutes at 95 °C, 5 seconds at 95 °C, 20 seconds at 60 °C, and 15 seconds at 72 °C, with a total of 40 cycles. After the amplification reaction, we performed melt curve analysis. Calculation of the expression of the target gene was carried out using the 2-ΔΔCT method. The experiment was conducted in triplicate and the average value was recorded as the result.
Cell transfection
Based on the results of qRT-PCR, Eca-109 cells with the highest expression level of circATXN7 (overexpression experiments) and EC-9706 cells with the lowest expression levels (knockdown experiments) were selected for transfection. An overexpression lentiviral vector (circATXN7) and a blank control group (an empty vector without circATXN7 gene sequence) were synthesized. Two knockdown vectors [short hairpin RNA (shRNA)#1 and shRNA#2] targeting the circATXN7 sequence and a blank control vector (shRNA control) were designed at the same time. The transfection method was as follows. Cells (1×106 cells/well) were seeded in a 24-well plate, and Lipofectamine 2000 reagent was used to transfer the transfected RNA into EC cells with a confluence of 60%. Lipofectamine 2000 (1 µL) was mixed with serum-free medium (50 µL) and incubated for 5 minutes. Mixtures of 20 pmol of transfected RNA and 50 µL of the serum-free medium were prepared and incubated for 5 minutes each. The above 2 mixtures were then mixed and left to stand for 20 minutes at room temperature to obtain RNA and liposome complexes. Next, a culture dish containing cells was added, and the culture medium was changed to a complete medium after 6 hours of transfection. Determination of circATXN7 expression was carried out after 48 hours of transfection using qRT-PCR to verify whether the transfection was successful.
MTT assay
Cell proliferation was determined through MTT assay. After transfection, 96-well plates were seeded with the transfected cells at 5×103/well density. After 48 hours, MTT solution (20 µL) was added to each well and left to incubate for 4 hours, followed by the addition of dimethyl sulfoxide (150 µL) and shaking for 10 minutes to crystallize the solution. The absorbance [optical density (OD)] value was detected at 490 nm with a microplate reader.
Colony assay
After transfection, the cells of each group were seeded in 6-well plates and placed in a 37 °C, 5% CO2 cell incubator. Every three days, the medium was changed and if visible clusters formed in the culture on days 10–14, they were terminated from the study. After 21 days the culture medium was discarded, and cells were gently rinsed with phosphate-buffered saline (PBS) 3 times. Next, 1 mL of 4% paraformaldehyde was added to each well, and the plates were stored at 4 °C in the refrigerator for 30 minutes. The cells were washed again with PBS 3 times, and 1 mL of 0.1% crystal violet was added to each well. The cells underwent staining for 30 minutes at 4 °C and were then washed a final time with double-distilled water. The number of clones formed was counted. Each experiment was repeated 3 times. The colony formation rate was calculated as follows: colony formation rate (%) = (number of clones/total number of inoculated cells) × 100%.
Flow-Cytometric Analysis
Cells for cell-cycle analysis were stained with Cell cycle detection Kit following the manufacturer’s protocol and analyzed by FACScan. The percent of the cells in G0/G1, S and G2/M phases were counted and compared.
Transwell migration assay
We used transwell chambers to measure the invasion and migration ability of cells. Transwell chambers coated (invasion assays) or uncoated (migration assays) with matrigel were placed in 24-well plates. A serum-free medium was used to adjust the cell concentration. The upper chamber of the transwell insert was seeded with cell suspension (200 µL) of approximately 2×105 cells, while 500 µL of medium containing FBS (20%) was placed into the lower chamber. After 24 hours of incubation, 1% formaldehyde was used to fix the invaded and migrated cells at the bottom of the transwell chamber, which were then stained with crystal violet. Cell invasion and migration were observed through an inverted microscope, and 5 fields of view were randomly selected for counting and taking pictures, with the mean value representing the number of invaded and migrated cells.
Statistical analysis
SPSS 22.0 and GraphPad Prism 5.0 were used for data analysis. Measurement data in the study are expressed as mean ± standard deviation (). Comparison of 2 groups was carried out using t-test. Count data are represented by n, and the χ2 test was used for comparison between 2 groups. P<0.05 indicated that the difference was statistically significant.
Results
Expression levels of circATXN7 in esophageal cancer tissues and cell lines
CircATXN7 level was determined by qRT-PCR, with the results showing significantly higher circATXN7 level in EC tissues (P<0.01) compared with normal paracancerous tissues (Figure 1A). The results indicated the involvement of circATXN7 in the incidence and development of EC. In addition, circATXN7 expression levels were tested in normal esophageal and EC cell lines. The findings showed higher expression in EC cell lines, among which EC-9706 had the highest expression level, followed by KYSE-150, TE-1, KYSE-30, and Eca-109 (Figure 1B). The higher expression of circATXN7 in EC cell lines suggested the gene was involved in regulating the development of EC.
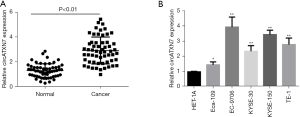
Correlation analysis of circATXN7 expression in esophageal cancer tissues and clinical and pathological characteristics of patients
To further investigate the relationship between circATXN7 expression and patient clinical and pathological characteristics, we divided patients into 2 distinct groups based on circATXN7 level: a high expression group (≥2.76) and low expression group (<2.76). Each group was further divided according to tumor invasion depth, smoking status, lymph node metastasis, and tumor/lymph nodes/metastasis (TNM) stage. The results showed a significant correlation for circATXN7 expression with lymph node metastasis and TNM staging, but not with age, gender, or differentiation of EC patients (Table 2).
Table 2
Clinical feature | CircATXN7 | P | |
---|---|---|---|
Low (n=21) | High (n=37) | ||
Sex | |||
Male | 15 | 32 | 0.1814 |
Female | 6 | 5 | |
Age, years | |||
<60 | 3 | 14 | 0.0758 |
≥60 | 18 | 23 | |
Level of differentiation | |||
Poorly-differentiated | 14 | 29 | 0.3629 |
Highly-differentiated | 7 | 8 | |
Smoking | |||
Yes | 10 | 25 | 0.1684 |
No | 11 | 12 | |
Depth of invasion | |||
T1–T2 | 16 | 14 | 0.1376 |
T3–T4a | 5 | 23 | |
TNM staging | |||
I–II | 14 | 12 | 0.0151 |
III | 7 | 25 | |
Lymph node | |||
No | 17 | 17 | 0.0127 |
Yes | 4 | 20 |
EC, esophageal cancer.
CircATXN7 overexpression and knockdown in EC cell lines
EC cell line EC-9706 had the highest circATXN7 expression level and Eca-109 had the lowest, and thus they were selected for knockdown and overexpression experiments, respectively, to further reveal the specific biological functions and corresponding molecular regulatory network of circATXN7 in EC cells. The results indicated that compared to blank control (vector), circATXN expression level in Eca-109 cells was significantly increased after transfection (P<0.01; Figure 2A). In EC-9706 cells, transfection with knockdown vectors (shRNA#1 and shRNA#2) significantly reduced the level of circATXN7 compared to blank control vector (shRNA control) (P<0.01; Figure 2B). The above results showed that EC cell line overexpression and knockdown of circATXN7 was successfully constructed in this study, providing an experimental basis for subsequent functional studies.
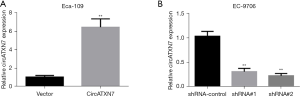
Effects of overexpression or knockdown of circATXN7 on the proliferation of esophageal cancer cells
Based on the overexpression and knockdown cell lines constructed above, MTT cell viability assay was performed to examine the influence of overexpression or knockdown of circATXN7 on the proliferation of EC cells (Eca-109 and EC-9706). The results showed that in circATXN7 vector-transfected Eca-109 cells, compared with the blank control group (vector), 72 hours or 96 hours culture significantly promoted cell proliferation (P<0.05; Figure 3A). In EC-9706 cells transfected with lentiviruses containing circATXN7 interfering sequence 1 (shRNA#1) and circATXN7 interfering sequence 2 (shRNA#2), compared with the blank control group (shRNA control), 72 hours and 96 hours culture decreased the proliferative ability of EC cells (Figure 3B; P<0.05).
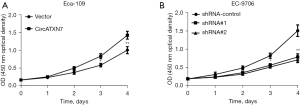
Effects of overexpression or knockdown of circATXN7 on colony formation assay in esophageal cancer cells
Colony formation assay was conducted to further examine the effect of overexpression or knockdown of circATXN7 on colony formation in EC Eca-109 and EC-9706 cells. The results showed that in overexpressing circATXN7 Eca-109 cells, compared to the control group (vector), the number of colonies formed by EC cells significantly increased after the addition of transfected cells to 6-well plates for 21 days (P<0.01), suggesting an increase in cell proliferation (Figure 4A). In EC-9706 cells, the transfected cells were also seeded into 6-well plates and cultured for the same time duration. Compared with the blank control group (shRNA control), transfection containing circATXN7 interfering sequence 1 (shRNA#1) and circATXN7 interfering sequence 2 (shRNA#2) significantly inhibited the number of colonies formed in EC cells (Figure 4B; P<0.01).
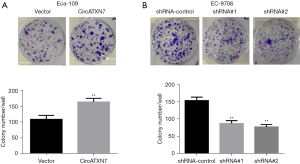
Effects of overexpression or knockdown of circATXN7 on the cycle distribution of EC cells
In Eca-109 cells, transfection of circATXN7 overexpressing vector can significantly affect the cycle distribution of esophageal cancer cells: compared with the blank control group (Vector), overexpression of circATXN7 can significantly inhibit the percentage of esophageal cancer cells in G0/G1 phase, and correspondingly significantly promote the percentage of cells in S phase and G2/M phase (Figure 5A). In EC-9706 cells, transfection and knockdown of the circATXN7 sequence significantly affected the cycle distribution of esophageal cancer cells: Compared with the blank control group (shRNA control), transfection of lentiviruses containing circATXN7 interfering sequence 1 (shRNA#1) and circATXN7 interfering sequence 2 (shRNA#2) can significantly promote the percentage of esophageal cancer cells in G0/G1 phase, the corresponding percentage of significantly suppressed cells in S phase (Figure 5B), while the ratio of cells to G2/M phase was also decreased, but the difference was not statistically significant. The above results suggest that overexpression or knockdown of circATXN7 can significantly affect the cycle distribution of esophageal cancer cells.
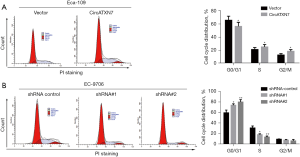
Effects of overexpression or knockdown of circATXN7 on invasion and migration of esophageal cancer cells
Transwell migration assay revealed that compared with the blank control group (vector), the circATXN7 vector promoted the invasion and migration of Eca-109 cells considerably (Figure 6). In EC-9706 cells, knockdown of circATXN7 significantly inhibited EC cell invasion and migration compared to the shRNA control (Figure 7).
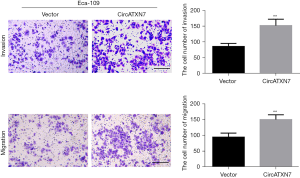
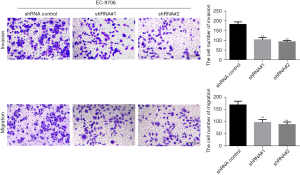
Discussion
EC is considered one of the most severe malignant tumors of the upper gastrointestinal tract worldwide (10). Based on the shape of the cancer cells under the microscope, EC has 2 distinct types: adenocarcinoma (EAC) and squamous cell carcinoma (ESCC), with ESCC accounting for 90% of the total incidence (11). With rapid development of multiomics and molecular biology in recent years, high-throughput sequencing can be used to analyze the mutation map of the ESCC gene, revealing extensive genomic alterations in the ESCC genome. For example, whole-genome and whole-exome sequencing and array comparative genomic hybridization showed that 83% of ESCCs contained somatic mutation tumor protein 53 (TP53), while in 2–10% of ESCCs, genes related to cell cycle control such as cyclin-dependent kinase inhibitor 2A (CDKN2A), nuclear factor erythroid 2-like factor 2 (NFE2L2), and retinoblastoma (RB1) were found. Both checkpoint kinase 1 (CHEK1) and CHEK2 and the classic differentiation-related pathways NOTCH1 and NOTCH3 are frequently mutated (12,13). Although significant advancement has been made in the early diagnosis and targeted therapy of EC, 40–50% of patients have developed local invasion or distant metastasis at the time of diagnosis, resulting in a 15–20% 5-year survival rate (14,15). In addition, recurrence and metastasis of EC are key factors affecting the prognosis of patients. Due to the strong heterogeneity of EC, the driving genes and molecular mechanisms of recurrence and invasion are still unclear, and as a result, the development and clinical application of molecularly targeted drugs for EC has been stagnant. However, a detailed study of the molecular mechanism of EC occurrence, development, and metastasis will help to provide new targets for EC therapy.
More than half of the DNAs of higher organisms are transcribed into RNAs, and most of them are noncoding RNAs (ncRNAs), which are RNAs that are unable to code protein. These include circRNAs, lncRNAs, and miRNAs (16,17). A study has shown that ncRNAs have been identified as tumor promoters or suppressors and exert a key regulatory role in the existence and development of various tumors (18). Among them, circRNAs, an entirely new type of RNA which is distinct from linear RNA, have unique characteristics and diverse cellular functions that are currently being explored (19). CircRNAs have closed-loop structures and are abundant in eukaryotic transcriptomes due to their insensitivity to nucleases (20,21). Therefore, circRNAs are more stable than linear RNAs and have high tissue-specific expression in the eukaryotic transcriptome, making them a promising molecular target for atherosclerotic vascular disease risk, eclampsia, neurological diseases, prions disease, osteoarthritis, and diabetes (22,23). In addition, circRNAs show abnormal expression in various types of cancers, including EC, GC, hepatocellular carcinoma, and pancreatic ductal adenocarcinoma. So far, people's understanding of circRNA is still very limited, and many aspects such as its formation mechanism, transcription and translation regulation need to be further improved and explored. It is believed that with the development of bioinformatics and the in-depth study of circRNA by researchers, the questions about circRNA will be gradually answered and better applied in clinical practice.
When this study commenced in 2019, there were no reports published on circATXN7, and now 3 articles on the function of circATXN7 can be found in PubMed. For example, a 2019 study found that compared to paracancerous tissue, the expression level of circATXN7 was higher in non-small cell lung carcinoma cells. Compared with non-small cell lung carcinoma patients with low circATXN7 expression, patients with high levels of circATXN7 had a shorter survival time, although no statistical significance was observed (P>0.05). Furthermore, circATXN7 silencing by small interfering RNA retarded the non-small cell lung cancer cells’ proliferation and invasion (in vitro) (24). A 2020 study of GC found that the expression of circATXN7 was upregulated in GC tissues and cell lines, and silencing of circATXN7 suppressed GC cell proliferation and invasion and promoted cell apoptosis (25). Elevated levels of circATXN7 were found in breast cancer tissues and cells in 2021. CircATXN7 silencing inhibits doxorubicin resistance and breast cancer development (26). We observed results that were consistent with the previously published reports. Our analysis of 58 cases of EC tissues, paracancerous tissues, and EC cells showed that circATXN7 was overexpressed in EC tissues and cell lines. Correlation analysis of circATXN7 with clinical and pathological characteristics of patients with EC revealed significant correlation of circATXN7 expression with lymph node metastasis and TNM staging of patients with EC. In addition, this study constructed circATXN7 overexpression and knockdown cell lines, respectively. Cell proliferation experiments demonstrated that an elevated level of circATXN7 could significantly promote EC cell proliferation, while circATXN7 knockdown might have inhibited their proliferation. The effect of circATXN7 on cell cycle distribution was detected by flow cytometry, and it was found that overexpression of circATXN7 could significantly promote the percentage of cells in S phase and G2/M phase, while knockdown of CircATXN7 can significantly inhibit the percentage of cells in S phase and G2/M phase. Invasion and migration experiments further proved that overexpression of circATXN7 could significantly promote EC cell migration and invasion, while knockdown of circATXN7 significantly inhibited the invasion and migration of EC cells. CircATXN7 affects the proliferation, cell cycle, invasion and migration of EC cells, which can be used as a biomarker for the diagnosis of esophageal cancer and the evaluation of patient prognosis, and has the potential as a therapeutic target for patients with radioresistant EC.
In conclusion, the results of this study preliminarily demonstrated higher circATXN7 expression in EC tissues and cell lines and exhibited a significant correlation with EC patients’ clinical and pathological characteristics. The findings of the current study elucidated the role of circATXN7 in EC incidence and development, and provided molecular targets and a theoretical basis for early EC diagnosis and targeted therapy.
Acknowledgments
Funding: The study was funded by the Natural Science Foundation of Jiangsu Province (No. BK20201496) and scientific research project of Jiangsu Cancer Hospital (No. ZM202012).
Footnote
Reporting Checklist: The authors have completed the MDAR reporting checklist. Available at https://jgo.amegroups.com/article/view/10.21037/jgo-22-481/rc
Data Sharing Statement: Available at https://jgo.amegroups.com/article/view/10.21037/jgo-22-481/dss
Conflicts of Interest: All authors have completed the ICMJE uniform disclosure form (available at https://jgo.amegroups.com/article/view/10.21037/jgo-22-481/coif). The authors have no conflicts of interest to declare.
Ethical Statement: The authors are accountable for all aspects of the work in ensuring that questions related to the accuracy or integrity of any part of the work are appropriately investigated and resolved. The study was conducted in accordance with the Declaration of Helsinki (as revised in 2013). The study was approved by the Medical Ethics Committee of Jiangsu Cancer Hospital (No. 2020 Section-011). Signed informed consent was obtained from the patients or their family members.
Open Access Statement: This is an Open Access article distributed in accordance with the Creative Commons Attribution-NonCommercial-NoDerivs 4.0 International License (CC BY-NC-ND 4.0), which permits the non-commercial replication and distribution of the article with the strict proviso that no changes or edits are made and the original work is properly cited (including links to both the formal publication through the relevant DOI and the license). See: https://creativecommons.org/licenses/by-nc-nd/4.0/.
References
- Ghafouri-Fard S, Shoorei H, Dashti S, et al. Expression profile of lncRNAs and miRNAs in esophageal cancer: Implications in diagnosis, prognosis, and therapeutic response. J Cell Physiol 2020;235:9269-90. [Crossref] [PubMed]
- Feng RM, Zong YN, Cao SM, et al. Current cancer situation in China: good or bad news from the 2018 Global Cancer Statistics? Cancer Commun (Lond) 2019;39:22. [Crossref] [PubMed]
- DiSiena M, Perelman A, Birk J, et al. Esophageal Cancer: An Updated Review. South Med J 2021;114:161-8. [Crossref] [PubMed]
- Chen LL. The expanding regulatory mechanisms and cellular functions of circular RNAs. Nat Rev Mol Cell Biol 2020;21:475-90. [Crossref] [PubMed]
- Chen L, Wang C, Sun H, et al. The bioinformatics toolbox for circRNA discovery and analysis. Brief Bioinform 2021;22:1706-28. [Crossref] [PubMed]
- Xu T, Wang M, Jiang L, et al. CircRNAs in anticancer drug resistance: recent advances and future potential. Mol Cancer 2020;19:127. [Crossref] [PubMed]
- Chen L, Shan G. CircRNA in cancer: Fundamental mechanism and clinical potential. Cancer Lett 2021;505:49-57. [Crossref] [PubMed]
- Chen J, Li Y, Zheng Q, et al. Circular RNA profile identifies circPVT1 as a proliferative factor and prognostic marker in gastric cancer. Cancer Lett 2017;388:208-19. [Crossref] [PubMed]
- Kong Z, Wan X, Zhang Y, et al. Androgen-responsive circular RNA circSMARCA5 is up-regulated and promotes cell proliferation in prostate cancer. Biochem Biophys Res Commun 2017;493:1217-23. [Crossref] [PubMed]
- Tay SW, Li JW, Fock KM. Diet and cancer of the esophagus and stomach. Curr Opin Gastroenterol 2021;37:158-63. [Crossref] [PubMed]
- Watanabe M, Otake R, Kozuki R, et al. Recent progress in multidisciplinary treatment for patients with esophageal cancer. Surg Today 2020;50:12-20. [Crossref] [PubMed]
- Huang FL, Yu SJ. Esophageal cancer: Risk factors, genetic association, and treatment. Asian J Surg 2018;41:210-5. [Crossref] [PubMed]
- Joo MK, Park JJ, Chun HJ. Impact of homeobox genes in gastrointestinal cancer. World J Gastroenterol 2016;22:8247-56. [Crossref] [PubMed]
- Domper Arnal MJ, Ferrández Arenas Á, Lanas Arbeloa Á. Esophageal cancer: Risk factors, screening and endoscopic treatment in Western and Eastern countries. World J Gastroenterol 2015;21:7933-43. [Crossref] [PubMed]
- Uhlenhopp DJ, Then EO, Sunkara T, et al. Epidemiology of esophageal cancer: update in global trends, etiology and risk factors. Clin J Gastroenterol 2020;13:1010-21. [Crossref] [PubMed]
- Anastasiadou E, Jacob LS, Slack FJ. Non-coding RNA networks in cancer. Nat Rev Cancer 2018;18:5-18. [Crossref] [PubMed]
- Zhou B, Yang H, Yang C, et al. Translation of noncoding RNAs and cancer. Cancer Lett 2021;497:89-99. [Crossref] [PubMed]
- Slack FJ, Chinnaiyan AM. The Role of Non-coding RNAs in Oncology. Cell 2019;179:1033-55. [Crossref] [PubMed]
- Patop IL, Kadener S. circRNAs in Cancer. Curr Opin Genet Dev 2018;48:121-7. [Crossref] [PubMed]
- Kristensen LS, Hansen TB, Venø MT, et al. Circular RNAs in cancer: opportunities and challenges in the field. Oncogene 2018;37:555-65. [Crossref] [PubMed]
- Li J, Sun D, Pu W, et al. Circular RNAs in Cancer: Biogenesis, Function, and Clinical Significance. Trends Cancer 2020;6:319-36. [Crossref] [PubMed]
- Shafabakhsh R, Mirhosseini N, Chaichian S, et al. Could circRNA be a new biomarker for pre-eclampsia? Mol Reprod Dev 2019;86:1773-80. [Crossref] [PubMed]
- Shi Y, Jia X, Xu J. The new function of circRNA: translation. Clin Transl Oncol 2020;22:2162-9. [Crossref] [PubMed]
- Huang Q, Wang S, Li X, et al. Circular RNA ATXN7 is upregulated in non-small cell lung cancer and promotes disease progression. Oncol Lett 2019;17:4803-10. [Crossref] [PubMed]
- Zhang Z, Wu H, Chen Z, et al. Circular RNA ATXN7 promotes the development of gastric cancer through sponging miR-4319 and regulating ENTPD4. Cancer Cell Int 2020;20:25. [Crossref] [PubMed]
- Wang H, Shan S, Wang H, et al. CircATXN7 contributes to the progression and doxorubicin resistance of breast cancer via modulating miR-149-5p/HOXA11 pathway. Anticancer Drugs 2022;33:e700-10. [Crossref] [PubMed]