Low CPEB1 levels may predict the benefit of 5-fluorouracil treatment in patients with colon or stomach adenocarcinoma
Introduction
Colon adenocarcinoma and stomach adenocarcinoma are two of the most common cancers globally. Since its discovery in 1957, 5-fluorouracil (5-FU) has been used as a first-line treatment for colorectal cancer (CRC) (1-4). Nevertheless, the efficacy of 5-FU-based chemotherapy for CRC is disappointing, as most patients acquire drug resistance during treatment, resulting in a median survival time of only about 20 months (5,6). Furthermore, for patients with gastric cancer (GC), the benefits of palliative chemotherapy and supportive treatment are limited, with a response rate ranging from 25% to 50% and the median survival being 6 to 12 months (7). Therefore, chemoresistance is the main obstacle to improving the therapeutic effect of 5-FU in patients with CRC and GC.
Cytoplasmic polyadenylation element-binding protein 1 (CPEB1) binds to cytoplasmic polyadenylation elements (CPEs) of the specific mRNA 3'-untranslated region (UTR) by anchoring the atypical poly(A) polymerases Gld2 and Gld4 and the deadenylase poly(A)-specific ribonuclease (PARN) (8,9). This binding regulates the addition or removal of poly(A) tails, thereby promoting or inhibiting translation, and is particularly important for regulating mRNAs involved in cell cycle G2/M progression (10,11). Decreased CPEB1 levels are implicated in cell invasion and angiogenesis in several cancers (12). Knockout of CPEB1 results in metastasis-related mRNAs having shorter or longer poly(A) tails. In breast cancer, CPEB1 levels decrease when cells metastasize (13). There is also strong evidence that CPEB1 regulates the differentiation of glioma stem cells and inhibits the proliferation of glioblastoma cells (14,15). Moreover, recent study has shown that CPEB1 mediates stem cell chemoresistance in hepatocellular carcinoma (16). However, the relationship between CPEB1 expression and 5-FU treatment response in colon and gastric adenocarcinoma have not been examined.
In the present study, we analyzed the relationships between 5-FU targets and the prognosis of patients treated with this drug. Our results indicate that CPEB1 may be a new predictor of the efficacy of 5-FU in patients with colon or gastric adenocarcinoma. We present the following article in accordance with the MDAR reporting checklist (available at https://jgo.amegroups.com/article/view/10.21037/jgo-22-561/rc).
Methods
Patients and treatment
Nine patients with colorectal adenocarcinoma and six patients with gastric adenocarcinoma who were treated at Changhai Hospital were enrolled in this study. Tumor and adjacent normal tissues from these patients were subjected to quantitative real-time PCR (qRT-PCR) and m6A methylated RNA immunoprecipitation quantitative PCR (meRIP qPCR). The study was conducted in accordance with the Declaration of Helsinki (as revised in 2013). The study was approved by the Ethics Committee of Changhai Hospital (No. 16ZR1400800). Written informed consent was obtained from each participant.
Immunohistochemistry
Immunohistochemistry assays were performed as previously described (17). The staining intensity for each marker was scored visually using the following four-point scale: 0= no staining, 1= weak staining, 2= moderate staining, and 3= strong staining. Based on this score, the H-score was calculated for the percentage of positive cells, on a scale from 0 (no staining) to 300 (diffuse, strong staining).
RT-PCR
Total RNA isolation and RT-PCR were performed as previously described (17). All expression values were normalized to β-actin expression.
m6A meRIP qPCR
MeRIP analysis was performed according to the manufacturer’s instructions (Magna meRIP m6A). The beads were then washed and eluted with 5'-sodium monophosphate (m6A) to retrieve the bound RNA. The eluted RNA was purified using miRNeasy miniature kits (QIAGEN, German) and finally, qPCR was performed.
Cell lines
The CRC cell lines HCT116 and LOVO and the GC cell lines MGC-803 and GBC-803 were purchased from the American Type Culture Collection Center (ATCC; Manassas, VA, USA) and cultured at 37 ℃ in Eagle’s Minimum Essential Medium, as specified by the ATCC (catalog No. 30-2003; ATCC) with 10% fetal bovine serum (FBS; Sangon Biotech Co., Ltd., Shanghai, China).
Cell transfection
Synthesis of si-FTO, si-CPEB1, and si-Con was performed by Shanghai Gene Pharmaceutical Co., Ltd. Cells were transfected with the siRNAs (50 nM) at 37 ℃ using LipofectamineTM 2000 (Invitrogen, USA; Thermo Fisher Scientific, Inc., USA). At 48 hours after transfection, cells were collected for subsequent analyses. siRNA transfections were si-Con: 5'-UGAGGACCUGGGU-3'; si-CPEB1: 5'-AUCUGAUCCAGAGCUGAAGCC-3'; si-FTO 5'-UGUGAUCCAGAGCUGAAGCC-3'.
Cell migration and invasion assays
The migration and invasion abilities of GC and CRC cells were examined as previously described (16). The cells were inoculated into 500 µL FBS-free DMEM in the upper lumen (2×104/well) and DMEM with 10% FBS in the lower lumen. The cells were cultured at 37 ℃ and 5% CO2 for 1 day. For the Matrigel intrusion test, the Transwell chamber was coated with Matrigel (BD Biosciences, San Jose, CA, USA). A total of 5×104 transfected cells were inoculated into the upper lumen of Transwell in 200 µL medium without FBS, while 500 µL medium supplemented with 20% FBS was placed into the lower lumen. The cells were cultured at 37 ℃ for 24 h for migration test and 48 h for invasion test. In both tests, cells were immobilized with 100% methanol for 5 min (Haimen Beotimi Biotechnology Institute, China) and stained with 0.5% crystal violet for 5 min (Beotimi Biotechnology Institute, China). The remaining cells on the upper surface of the membrane are then carefully removed with a cotton swab. The migrating and invading cells were then counted using an inverted microscope (magnification ×200) in five randomly selected areas. Each experiment was repeated three times.
Statistical analysis
Statistical analysis was performed with GraphPad Prism 7 (GraphPad Software, San Diego, CA, USA). The baseline clinicopathological features of the patients were analyzed using the chi-squared test. Survival was estimated using the Kaplan-Meier method and compared by logarithmic rank testing. P value <0.05 was considered statistically significant.
Results
CPEB1 expression is increased in colon and stomach adenocarcinoma
Analysis of data from the GEPIA2 database (http://gepia2.cancer-pku.cn/#index) revealed that patients whose tumors expressed high levels of CPEB1 had shorter overall and disease-free survival (Figure 1A,1B). Results of IHC examination indicated that CPEB1 was also increased at the protein level in colon and stomach adenocarcinoma tissues relative to the adjacent normal tissues. Moreover, a high CPEB1 protein level was associated with poor 5-FU response in the 9 patients with colon adenocarcinoma and 6 with stomach adenocarcinoma (Figure 2A,2B). This observation evidences a clear association between the level of CPEB1 and 5-FU treatment benefit in patients with relapsed colon or stomach adenocarcinoma.
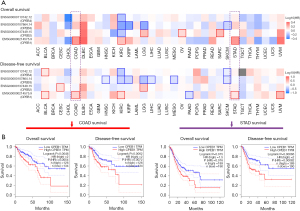
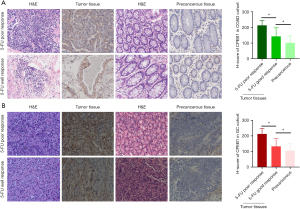
Effects of CPEB1 on metastatic potential and invasive behavior of GC and CRC cells in vitro
To functionally characterize the effects of CPEB1 in GC and CRC, the CRC cell lines HCT116 and LOVO and the GC cell lines MGC-803 and GBC-803 were selected for a loss-of-function study. To this end, GC and CRC cells were stably downregulated for CPEB1, and the knockdown of CPEB1 expression was confirmed by qRT-PCR (Figure S1A,S1B). Transwell assays revealed that knockdown of CPEB1 decreased the migration and invasion abilities of GC and CRC cells (Figure 3A-3D). These data indicate that CPEB1 may promote GC and CRC cell metastasis in vitro.
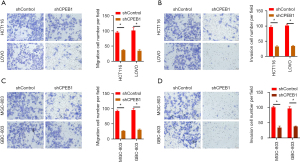
CPEB1 regulates the expression of matrix metalloprotein (MMP)2, 7, and 9 in GC and CRC cells
Tumor invasion is orchestrated by an increase in the proteolytic activity of MMPs, which degrades the neighboring stroma and facilitates the spread of tumor cells. Using the GEPIA2 database (http://gepia2.cancer-pku.cn/#index) we found that high CPEB1 expression was positively associated with the expression of MMP2, 7, and 9 in GC and CRC tissues (Figure 4A,4B). A marked decrease in MMP2, 7, and 9 protein expression was also observed in GC and CRC cell lines after CPEB1 knockdown (Figure 4C,4D). These observations suggest that, at least in part, tumor metastasis is positively influenced by CPEB1 via its regulation of MMP2, 7, and 9 expressions in GC and CRC cells.
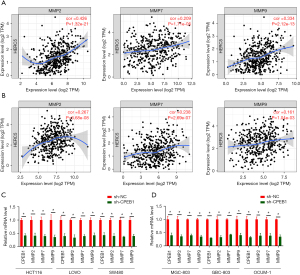
FTO-induced m6A modification is responsible for increasing CPEB1 expression in colon and stomach adenocarcinoma
Again, using the GEPIA2 database, we analyzed the correlations between the expression of CPEB1 and that of methylases (METTL3, METTL14, WTAP, and KIAA1429) and demethylases (ALKBH5 and FTO). Results showed that the m6A demethylase FTO was positively associated with CPEB1 in both colon and stomach adenocarcinoma (Figure 5A). Knockdown of FTO significantly decreased the expression of CPEB1 (Figure 5B), which suggested that m6A modification could be involved in the increase levels of CPEB1 in colon and stomach adenocarcinoma cells. In subsequent study, a RIP assay validated m6A modification of CPEB1 mRNA in colon and stomach adenocarcinoma cells and also confirmed the role played by FTO in this modification (Figure 5C). As shown in Figure 5D, the expression levels of FTO and CPEB1 were increased in tumor tissues compared to their adjacent normal tissues (Figure 5D,5E). Moreover, meRIP qPCR analysis of 9 paired colon and 6 paired stomach adenocarcinoma samples revealed that the m6A levels of CPEB1 were lower in tumor tissues than in the adjacent normal tissues (Figure 5F). Consistent with this result, a positive correlation between FTO expression and CPEB1 levels was observed (Figure 5G). These results suggest that FTO-induced m6A modification is responsible for the increased expression levels of CPEB1 in colon and stomach adenocarcinoma.
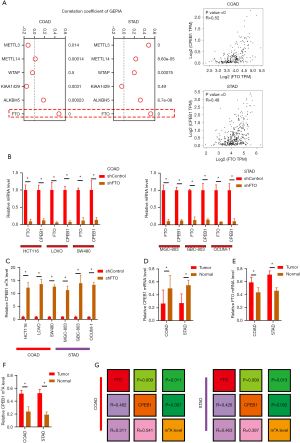
Discussion
The post-transcriptional regulatory factor CPEB1 is involved in cytoplasmic polyadenylation, which may influence tumorigenesis in various malignant tumors (18). However, the expression and role of CPEB1 in colon adenocarcinoma and stomach adenocarcinoma have remained largely unknown. Based on the results of the present study, we propose that CPEB1 is markedly upregulated in colon and stomach adenocarcinoma tissues via FTO-dependent m6A RNA demethylation machinery. We found that increased CPEB1 expression was correlated with poor survival in patients with colon and stomach adenocarcinoma. Moreover, patients with high CPEB1 expression exhibited a poor response to 5-FU treatment.
Although patient life expectancy can be significantly extended by 5-FU-based chemotherapy, drug resistance is a major barrier to the success of this treatment (19). Due to complex and heterogeneous biological processes, many of the mechanisms of chemoresistance remain elusive (20). Therefore, there is a need to identify novel biomarkers for patient selection to improve the efficacy of 5-FU. Our clinical investigation revealed that patients with colon or stomach adenocarcinoma who had low CPEB1 levels benefited from 5-FU therapy, whereas those with high CPEB1 levels did not. Collectively, these findings suggest that CPEB1 may serve as a predictor of 5-FU benefit in the personalized therapy of colon and stomach adenocarcinoma, which warrants further investigation.
Emerging studies have demonstrated that m6A modification of mRNAs plays a critical role in RNA fate, including mRNA stability, splicing, transport, localization, and translation (21-26). Previous studies indicated that N6-methyladenosine was associated with 5-FU resistance in CRC (27,28). Demethylation of m6A has been reported to be modulated by FTO and ALKBH5 (25-33). Based on the results of the present study, we propose that CPEB1 expression in colon and stomach adenocarcinoma is regulated by RNA methylation, as assessed using the GEPIA2 database and our observations that interference with FTO resulted in an increase of m6A leading to a decrease of CPEB1. Analysis of clinical samples of colon and stomach adenocarcinoma revealed that the levels of CPEB1 and FTO mRNA were positively correlated, while the m6A CPEB1 level was negatively correlated with the levels of CPEB1 and FTO mRNA. However, the molecular mechanisms underlying the decrease of CPEB1 induced by m6A modification in colon and stomach adenocarcinoma cells remain obscure. To the best of our knowledge, this is the first study to report the involvement of m6A modification in CPEB1 dysregulation in GC and CRC.
In conclusion, we have revealed the value of CPEB1 in predicting the benefit of 5-FU treatment in colon or stomach adenocarcinoma. On this basis, we propose that low CPEB1 expression could serve as a novel biomarker for the use of 5-FU in the personalized therapy of patients with colon or stomach adenocarcinoma.
Acknowledgments
The authors would like to express their gratitude to EditSprings (https://www.editsprings.cn/) for the expert linguistic services provided.
Funding: None.
Footnote
Reporting Checklist: The authors have completed the MDAR reporting checklist. Available at https://jgo.amegroups.com/article/view/10.21037/jgo-22-561/rc
Data Sharing Statement: Available at https://jgo.amegroups.com/article/view/10.21037/jgo-22-561/dss
Conflicts of Interest: All authors have completed the ICMJE uniform disclosure form (available at https://jgo.amegroups.com/article/view/10.21037/jgo-22-561/coif). The authors have no conflicts of interest to declare.
Ethical Statement: The authors are accountable for all aspects of the work in ensuring that questions related to the accuracy or integrity of any part of the work are appropriately investigated and resolved. The study was conducted in accordance with the Declaration of Helsinki (as revised in 2013). The study was approved by the Ethics Committee of Changhai Hospital (No. 16ZR1400800). Written informed consent was obtained from each participant.
Open Access Statement: This is an Open Access article distributed in accordance with the Creative Commons Attribution-NonCommercial-NoDerivs 4.0 International License (CC BY-NC-ND 4.0), which permits the non-commercial replication and distribution of the article with the strict proviso that no changes or edits are made and the original work is properly cited (including links to both the formal publication through the relevant DOI and the license). See: https://creativecommons.org/licenses/by-nc-nd/4.0/.
References
- Bray F, Ferlay J, Soerjomataram I, et al. Global cancer statistics 2018: GLOBOCAN estimates of incidence and mortality worldwide for 36 cancers in 185 countries. CA Cancer J Clin 2018;68:394-424. [Crossref] [PubMed]
- Fan A, Wang B, Wang X, et al. Immunotherapy in colorectal cancer: current achievements and future perspective. Int J Biol Sci 2021;17:3837-49. [Crossref] [PubMed]
- Zhang Q, Liu F, Chen W, et al. The role of RNA m5C modification in cancer metastasis. Int J Biol Sci 2021;17:3369-80. [Crossref] [PubMed]
- Li J, Wang F, Liu Y, et al. N6-methyladenosine (m6A) in pancreatic cancer: Regulatory mechanisms and future direction. Int J Biol Sci 2021;17:2323-35. [Crossref] [PubMed]
- Long Y, Zhao Q, Huang Y. RNF38 enhances 5-Fluorouracil resistance in colorectal cancer by activating the Wnt pathway. J BUON 2021;26:1246-51. [PubMed]
- Li G, Fang S, Shao X, et al. Curcumin Reverses NNMT-Induced 5-Fluorouracil Resistance via Increasing ROS and Cell Cycle Arrest in Colorectal Cancer Cells. Biomolecules 2021;11:1295. [Crossref] [PubMed]
- Zhao Y, Wang C, Goel A. Andrographis overcomes 5-fluorouracil-associated chemoresistance through inhibition of DKK1 in colorectal cancer. Carcinogenesis 2021;42:814-25. [Crossref] [PubMed]
- Hake MJ, Choowongkomon K, Kostenko O, et al. Specificity determinants of a novel Nck interaction with the juxtamembrane domain of the epidermal growth factor receptor. Biochemistry 2008;47:3096-108. [Crossref] [PubMed]
- Kronja I, Orr-Weaver TL. Translational regulation of the cell cycle: when, where, how and why? Philos Trans R Soc Lond B Biol Sci 2011;366:3638-52. [Crossref] [PubMed]
- D'Ambrogio A, Nagaoka K, Richter JD. Translational control of cell growth and malignancy by the CPEBs. Nat Rev Cancer 2013;13:283-90. [Crossref] [PubMed]
- Novoa I, Gallego J, Ferreira PG, et al. Mitotic cell-cycle progression is regulated by CPEB1 and CPEB4-dependent translational control. Nat Cell Biol 2010;12:447-56. [Crossref] [PubMed]
- Hansen CN, Ketabi Z, Rosenstierne MW, et al. Expression of CPEB, GAPDH and U6snRNA in cervical and ovarian tissue during cancer development. APMIS 2009;117:53-9. [Crossref] [PubMed]
- Nagaoka K, Fujii K, Zhang H, et al. CPEB1 mediates epithelial-to-mesenchyme transition and breast cancer metastasis. Oncogene 2016;35:2893-901. [Crossref] [PubMed]
- Yin J, Park G, Lee JE, et al. CPEB1 modulates differentiation of glioma stem cells via downregulation of HES1 and SIRT1 expression. Oncotarget 2014;5:6756-69. [Crossref] [PubMed]
- Galardi S, Petretich M, Pinna G, et al. CPEB1 restrains proliferation of Glioblastoma cells through the regulation of p27(Kip1) mRNA translation. Sci Rep 2016;6:25219. [Crossref] [PubMed]
- Yu T, Yu J, Lu L, et al. MT1JP-mediated miR-24-3p/BCL2L2 axis promotes Lenvatinib resistance in hepatocellular carcinoma cells by inhibiting apoptosis. Cell Oncol (Dordr) 2021;44:821-34. [Crossref] [PubMed]
- Patnaik MM. The importance of FLT3 mutational analysis in acute myeloid leukemia. Leuk Lymphoma 2018;59:2273-86. [Crossref] [PubMed]
- Shiga T, Hiraide M. Cardiotoxicities of 5-Fluorouracil and Other Fluoropyrimidines. Curr Treat Options Oncol 2020;21:27. [Crossref] [PubMed]
- Deng J, Wang Y, Lei J, et al. Insights into the involvement of noncoding RNAs in 5-fluorouracil drug resistance. Tumour Biol 2017;39:1010428317697553. [Crossref] [PubMed]
- Liao ZK, Hu SH, Han BY, et al. Pro-pigmentary action of 5-fluorouracil through the stimulated secretion of CXCL12 by dermal fibroblasts. Chin Med J (Engl) 2021;134:2475-82. [Crossref] [PubMed]
- Liu J, Eckert MA, Harada BT, et al. m6A mRNA methylation regulates AKT activity to promote the proliferation and tumorigenicity of endometrial cancer. Nat Cell Biol 2018;20:1074-83. [Crossref] [PubMed]
- Schwartz S, Mumbach MR, Jovanovic M, et al. Perturbation of m6A writers reveals two distinct classes of mRNA methylation at internal and 5' sites. Cell Rep 2014;8:284-96. [Crossref] [PubMed]
- Lin S, Choe J, Du P, et al. The m(6)A Methyltransferase METTL3 Promotes Translation in Human Cancer Cells. Mol Cell 2016;62:335-45. [Crossref] [PubMed]
- Li Z, Weng H, Su R, et al. FTO Plays an Oncogenic Role in Acute Myeloid Leukemia as a N6-Methyladenosine RNA Demethylase. Cancer Cell 2017;31:127-41. [Crossref] [PubMed]
- Yu F, Wei J, Cui X, et al. Post-translational modification of RNA m6A demethylase ALKBH5 regulates ROS-induced DNA damage response. Nucleic Acids Res 2021;49:5779-97. [Crossref] [PubMed]
- Jiang ZX, Wang YN, Li ZY, et al. The m6A mRNA demethylase FTO in granulosa cells retards FOS-dependent ovarian aging. Cell Death Dis 2021;12:744. [Crossref] [PubMed]
- Cui Y, Zhang C, Ma S, et al. RNA m6A demethylase FTO-mediated epigenetic up-regulation of LINC00022 promotes tumorigenesis in esophageal squamous cell carcinoma. J Exp Clin Cancer Res 2021;40:294. [Crossref] [PubMed]
- Qiu X, Yang S, Wang S, et al. M6A Demethylase ALKBH5 Regulates PD-L1 Expression and Tumor Immunoenvironment in Intrahepatic Cholangiocarcinoma. Cancer Res 2021;81:4778-93. [Crossref] [PubMed]
- Shen M, Li Y, Wang Y, et al. N6-methyladenosine modification regulates ferroptosis through autophagy signaling pathway in hepatic stellate cells. Redox Biol 2021;47:102151. [Crossref] [PubMed]
- Lan Q, Liu PY, Bell JL, et al. The Emerging Roles of RNA m6A Methylation and Demethylation as Critical Regulators of Tumorigenesis, Drug Sensitivity, and Resistance. Cancer Res 2021;81:3431-40. [Crossref] [PubMed]
- Hu Y, Gong C, Li Z, et al. Demethylase ALKBH5 suppresses invasion of gastric cancer via PKMYT1 m6A modification. Mol Cancer 2022;21:34. [Crossref] [PubMed]
- Zhang B, Jiang H, Wu J, et al. m6A demethylase FTO attenuates cardiac dysfunction by regulating glucose uptake and glycolysis in mice with pressure overload-induced heart failure. Signal Transduct Target Ther 2021;6:377. [Crossref] [PubMed]
- Zhang C, Chen L, Lou W, et al. Aberrant activation of m6A demethylase FTO renders HIF2αlow/- clear cell renal cell carcinoma sensitive to BRD9 inhibitors. Sci Transl Med 2021;13:eabf6045. [Crossref] [PubMed]
(English Language Editor: J. Reylonds)