Circular RNA ZFR promotes cell cycle arrest and apoptosis of colorectal cancer cells via the miR-147a/CACUL1 axis
Introduction
Colorectal cancer (CC) is one of the most common malignancies worldwide and its fatality rate ranks second among all cancers, accounting for 9.2% of all cancer deaths (1). The symptoms of CC are not obvious in the early stages of the disease and the majority of the CC cases are diagnosed at the middle and advanced stages. Patients with advanced CC have a poor prognosis, which seriously endangers their life and health. However, research on the pathogenicity of CC and the underlying molecular mechanisms is still concentrated on its early stages (2). Therefore, the current study focused on the molecular mechanisms underlying CC progression.
Currently, circular RNAs (circRNAs) that have been detected in human tissues have been shown to be involved in different cellular processes, including senescence, cell proliferation, and apoptosis (3,4). CircRNAs are stable, diverse, and conserved RNA molecules that play indispensable roles in RNA interaction networks (4). Their potential clinical value in tumor diagnosis, treatment, and prognosis has been examined in detail. However, in spite of great advances in the study of circRNAs, several aspects still demand elaboration before it can be put into clinical practice (5). For instance, the impacts of circRNAs on cancer etiology are still not fully distinct, particularly in terms of drug resistance (5). Also, the clinical use of exosomal circRNAs remains challenging (5). Circular ZFR (circZFR) is a newly discovered circRNA that has been substantiated to hinder gastric cancer cell proliferation and promote apoptosis by regulating the microRNA (miR)-130a/miR-107/PTEN axis (6). CircZFR promotes the progression of bladder cancer by upregulating Wnt family member 5A and sponging miR-545 and miR-1270 (7). These results show that circZFR plays an important role in the development of digestive tract tumors. However, the involvement of circZFR in cell cycle progression and apoptosis in CC has not been reported so far.
CircRNAs act as ‘sponges’ for miRs and competitively inhibit their biological effects on downstream mRNAs (8). The expression of miRs is widely distributed across living organisms, suggesting that miRs are ancient and important regulators of cell function (9). The binding of miR-147a to circZFR was predicted via ENCORI database. Previous studies have shown abnormal expression of miR-147a in a variety of malignant tumors, such as non-small cell lung cancer (NSCLC) (10), cervical cancer (11), and ovarian cancer (12), indicating that it may be involved in the process of tumor formation and development. The influence of miR-147a on CC has been unclear to date.
This study predicted that CDK2 associated cullin domain 1 (CACUL1) could bind to miR-147a using the ENCORI database. CACUL1 is a novel cell cycle-associated gene, which has been proposed to serve as a tumor promoter in CC as well as lung cancer. A previous study demonstrated that the expression levels of CACUL1 in CC tissues were significantly higher than those noted in normal tissues. Moreover, the expression levels of CACUL1 were found to be significantly higher in drug-resistant CC cells, whereas knockout of CACUL1 expression inhibited the progression of CC drug-resistant cells from the G1 to the S phase of the cell cycle and increased their drug sensitivity by inducing apoptosis (13). However, the specific mechanism of action of CACUL1 in CC has not been reported to date.
In the present study, the regulatory effects of the circZFR/miR-147a/CACUL1 axis on cell cycle progression and apoptosis of CC cells were investigated. The findings may provide a theoretical basis for the mechanism of action of circZFR in CC. Further, this molecular mechanism can be used as a therapeutic target to augment the treatment of CC and improve the prognosis of CC patients. We present the following article in accordance with the MDAR reporting checklist (available at https://jgo.amegroups.com/article/view/10.21037/jgo-22-672/rc).
Methods
Cell culture
The normal colonic epithelial cell line FHC and the CC cell lines Caco-2, HCT8, LoVo, HCT116, and SW480 were obtained from BeNa Culture Collection. The cells were cultured in Dulbecco’s modified Eagle’s medium (DMEM; Gibco, CA, USA) with 10% fetal bovine serum (FBS; Gibco) in a humidified incubator containing 5% CO2 at 37 ℃.
Cell transfection
SW480 cells were plated in 6-well plates (1×106 cell/well) and transfected with RNAs or plasmids using Lipofectamine® 3000 (Invitrogen; Thermo Fisher Scientific, Inc., Boston, MA, USA). CircZFR specific short hairpin RNAs (shRNA-circZFR#1/2) as well as control (shRNA-NC), miR-147a mimic and its matching control (NC mimic), miR-147a inhibitor and its matching control (NC inhibitor), and CACUL1 plasmid (pcDNA3.1-CACUL1) and its matching empty vector (pcDNA3.1-NC) were obtained from Shanghai GenePharma Co., Ltd. (Shanghai, China). The cells were grouped as follows: control, shRNA-NC, shRNA-circZFR, shRNA-circZFR + NC inhibitor, shRNA-circZFR + miR-147a inhibitor, shRNA-circZFR + pcDNA3.1-NC, and shRNA-circZFR + pcDNA3.1-CACUL1.
Reverse transcription-quantitative PCR (RT-qPCR)
Following extraction of RNA with TRIzol® reagent (Thermo Fisher Scientific, Inc.), the single-stranded cDNAs were synthesized from 1 µg of RNA. The expression levels of mRNAs and miRs were quantified by RT-qPCR with SYBR-Green I (Thermo Fisher Scientific, Inc.). The specific primer sequences used in the present study were as follows: circZFR (forward, 5'-TCACACAGTTACTGCTGCCT-3'; reverse, 5'-GGGGGTGGTGGTGGTGGTGC-3') (14), miR-147a (forward, 5'-CCCCTATCACGATTAGCATTAA-3'; reverse, 5'-CCCAAGCTTTTATGTGGTTGTT-3'), U6 (a housekeeping gene for miRs and circZFR; forward, 5'-AAAGCAAATCATCGGACGACC-3'; reverse, 5'-GTACAACACATTGTTTCCTCGGA-3') (14), CACUL1 (forward, 5'-TGGGTTCAGA TGGCTCCAAC TCTATTTTC-3'; reverse, 5'-GACTGGTCAC CCCTTGTAAA ACCATTCTG-3'), and GAPDH (a housekeeping gene for mRNAs; forward, 5'-TGTTCGTCATGGGTGTGAAC-3'; reverse, 5'-ATGGCATGGACTGTGGTCAT-3') (15). The quantification was performed using the 2−ΔΔCq method (16).
Cell Counting Kit-8 (CCK-8) assay
SW480 cells were plated in 96-well plates (8×103 cell/well). Following the corresponding treatment, the survival rate of the cells was detected with the CCK-8 kit (Beyotime Institute of Biotechnology, Shanghai, China). Subsequently, 10 µL CCK-8 solution was added to each well and incubated for 2 h according to the manufacturer’s instructions. OD450 nm value was judged adopting a microplate reader (Omega Bio-Tek, Inc., Norcross, GA, USA).
Cell colony formation assay
SW480 cells were plated in 6-well plates (5×103 cell/well). The cells were treated accordingly, maintained in normal medium, and allowed to proliferate for 3 weeks. Subsequently, they were fixed with 4% paraformaldehyde and stained with 0.1% crystal violet solution. The colonies were observed and counted by light microscopy (SZX7; Olympus Corporation, Tokyo, Japan).
Flow cytometry assay
SW480 cells were plated in 6-well plates (1×106 cells/well). Following transfection, the cells were digested using 0.25% trypsin without EDTA and harvested by centrifugation at 300 ×g at 4 ℃ for 5 min following washing with cold phosphate-buffered saline (PBS). A total of 300 µL bovine serum albumin (BSA; 5%; ST025-5g, Beyotime Biological Technology Co., Ltd., Shanghai, China) and 700 µL pre-cooled alcohol (70%) were stored at −20 ℃ overnight following washing twice with 5% BSA. The following day, the cells were extracted by centrifugation (300 ×g for 5 min at 4 ℃) and resuspended in PBS. Finally, the cells were incubated with 1 µL RNAse A (10 mg/µL) for 20 min and measured by a FACSCantoTM II flow cytometer (BD Biosciences, Franklin Lakes, NJ, USA).
Transferase dUTP nick end labeling (TUNEL) assay
SW480 cells were plated in 6-well plates (5×105 cells/well). Following transfection, 4% paraformaldehyde was used to fix the cells for 30 min at 37 ℃. Subsequently, proteinase K was incubated with the cells for 30 min at 37 ℃ and 0.1% Triton X-100 was added for an additional incubation for 5 min. DAPI was used to stain the nuclei. Finally, the cells were observed using light microscopy (magnification 200×, Carl Zeiss AG, Berlin, Germany). ImageJ software (version 146, National Institutes of Health, Bethesda, MD, USA) was used to count the total number of cells and the number of TUNEL-positive cells.
Western blotting
RIPA lysis buffer (Beyotime, Shanghai, China) was used to lyse the cells on ice and extract the proteins. The BCA assay kit (Sigma-Aldrich; Merck KGaA, Darmstadt, Germany) was used to detect protein concentration. PVDF membranes (Thermo Fisher Scientific, Inc.) were to shift a total of 30 µg protein separated with 10–12% sodium dodecyl sulfate-polyacrylamide gels. Five percent skimmed milk powder was supplemented for non-specific binding. Primary antibodies and secondary antibodies (1:5,000; cat. no. ab181658; Abcam, Cambridge, UK) were added to the membranes overnight at 4 ℃ and for 2 h at room temperature separately in the light of the manufacturer’s instructions. The protein bands were obtained using Super Signal ECL (Pierce; Thermo Fisher Scientific, Inc.).
Luciferase assay
SW480 cells were transfected with miR-147a mimics, circZFR mutant, and luciferase reporter constructs using Lipofectamine 2000 (Invitrogen, Thermo Fisher Scientific, Inc.). Prior to the measurement of the relative luciferase activity using a microplate reader (BD Biosciences) in the Dual Luciferase Reporter Gene Assay System (Promega Corporation, Madison, WI, USA) which was normalized to Renilla luciferase activity in compliance with the manufacturer’s protocol, transfected cells were subjected to 48 h of culture.
Statistical analysis
The results are given based on mean ± standard deviation. All experiments were independently conducted in triplicate and all experimental data were biologically repeated in triplicate. Statistical analysis was performed with one-way ANOVA followed by Tukey’s post hoc test for multiple group comparisons and a paired independent t-test was used to compare the differences between 2 groups using SPSS 17.0 statistical software. It was denoted as statistical significance at P<0.05.
Results
Knockdown of circZFR expression inhibits the proliferation of CC cells
RT-qPCR was to test circZFR expression levels. The results indicated that circZFR expression levels were fortified in CC cell lines relative to those in FHC cells (Figure 1A). The most prominent increase was noted in SW480 cells (Figure 1A). Therefore, SW480 cells were selected for subsequent experiments. CircZFR interference plasmids were constructed by cell transfection and the transduction efficacy was examined with RT-qPCR. The expression levels of circZFR were significantly decreased in the shRNA-circZFR #2 group compared with those in the shRNA-NC group (Figure 1B). Therefore, shRNA-circZFR #2 was chosen for the ensuing experiments. The cells were grouped into control, shRNA-NC, and shRNA-circZFR groups. CCK-8 and colony formation assays indicated that cellular activity was remarkably reduced in the shRNA-circZFR group relative to that in the shRNA-NC group (Figure 1C). Cell proliferation was significantly reduced in the shRNA-circZFR group (Figure 1D,1E).
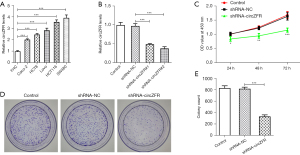
Interference of circZFR expression stimulates CC cell G1/S cell cycle arrest and apoptosis
Flow cytometry and western blot analyses were used to detect the cell cycle profile and the expression levels of cell cycle-associated proteins. The results indicated that cell cycle arrest of CC cells was induced by inhibition of circZFR expression (Figure 2A,2B). This was accompanied by a significant decrease in the expression levels of the cyclin-dependent kinase (CDK) enzymes CDK4, CDK6, and CDK2 and the cyclin D1 protein (Figure 2C). Subsequently, the TUNEL assay and western blot analysis were used to assess cell apoptosis. The percentage of apoptosis was prominently increased in the shRNA-circZFR group relative to that in the shRNA-NC group (Figure 2D-2F). This was accompanied by increased expression levels of Bax, c-poly (ADP-ribose) polymerase (PARP), and c-caspase 9 and decreased expression levels of Bcl-2 (Figure 2D-2F). The results indicated that interference with circZFR expression potentiated CC G1/S cell cycle arrest and apoptosis.
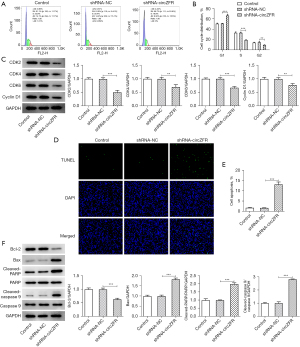
Knockdown of circZFR expression downregulates the expression levels of CACUL1 by sponging miR-147a
The ENCORI database predicted the binding sites between circZFR and miR-147a (Figure 3A). Subsequently, the expression levels of miR-147a in SW480 cells were detected by RT-qPCR analysis and the results indicated that they were abnormally altered (Figure 3B). Transfection efficiency was determined by RT-qPCR analysis following construction and transfection of the miR-147a-overexpressing plasmid (Figure 3C). The binding affinity between circZFR and miR-147a was assessed by the luciferase reporter gene assay (Figure 3D). Moreover, the expression levels of miR-147a were significantly increased following inhibition of the expression of circZFR in cells (Figure 3E). In addition, the ENCORI database predicted potential binding of miR-147a with CACUL1 (Figure 4A). However, the expression levels of CACUL1 were abnormally elevated in SW480 cells (Figure 4B,4C). Luciferase reporter assay analysis confirmed that miR-147a and CACUL1 displayed specific binding sites (Figure 4D). Moreover, the expression levels of CACUL1 were significantly decreased in both miR-147a-overexpressing cells and circZFR-knockdown cells (Figure 4E-4H). These findings indicated that knockdown of circZFR expression might downregulate the expression levels of CACUL1 by sponging miR-147a.
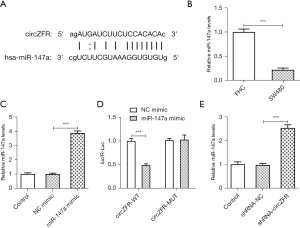
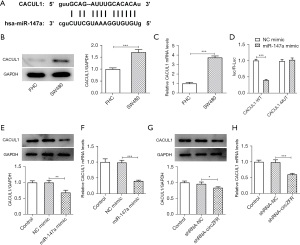
CircZFR/miR-147a/CACUL1 signaling regulates the cell cycle and apoptosis to promote the progression of CC
The miR-147a inhibitor and the CACUL1-overexpressing plasmid were constructed by cell transfection. These sequences were transfected into CC cells and the transfection efficiency was detected by RT-qPCR and western blot analyses (Figure 5A-5C). The shRNA-circZFR + miR-147a inhibitor group showed a significant increase in cell viability (Figure 5D) and cell proliferative ability compared with the shRNA-circZFR + NC inhibitor group (Figure 5E,5F). The decrease in cell cycle arrest was accompanied by increased expression of cyclin-associated proteins (Figure 6), decreased apoptosis (Figure 7A,7B), increased Bcl-2 expression, and decreased apoptotic proteins Bax, c-PARP, and c-caspase 9 expression (Figure 7C). The trend in cell proliferation, cell cycle arrest, and apoptosis in the shRNA-circZFR + pcDNA3.1-CACUL1 group was consistent with the trend in the shRNA-circZFR + miR-147a inhibitor compared with that of the shRNA-circZFR + pcDNA3.1-NC group. These results indicated that circZFR downregulated the expression levels of CACUL1 by sponging miR-147a, thereby regulating cell cycle progression and apoptosis and promoting the progression of CC.
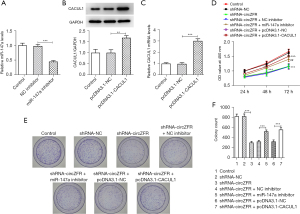
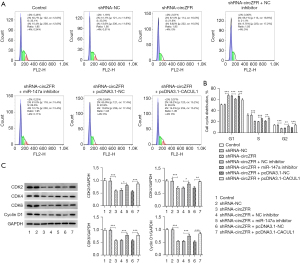
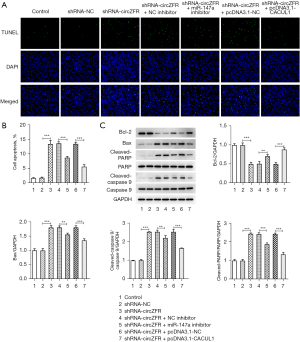
Discussion
The pathological mechanism of CC has not been thoroughly studied and effective therapeutic targets and biomarkers are not currently available. Therefore, it is crucial to further assess the pathogenesis of this disease and screen potential therapeutic targets and biomarkers. In our study, we examined the role of circZFR in cell cycle arrest and apoptosis in CC and explored the mechanisms involved. Our results showed that circZFR was highly expressed in CC cells, and downregulation of circZFR induced cycle arrest and apoptosis in CC cells, which was achieved through regulation of the miR-147a/CACUL1 axis. Therefore, circZFR might be a therapeutic target and biomarker for CC. Previous studies have shown that upregulation or downregulation of circRNA expression can be used as potential tumor promoters or suppressors. CircRNAs display abnormal expression in various digestive tract tumors, CC is also included. More importantly, circRNAs are closely related to the malignant progression of CC and may be used as a molecular target for the treatment of CC. For instance, circRNA_001569 modulates cell proliferation, and invasion in CC by sponging miR-145 (17). Circ3823 drives growth, metastasis and angiogenesis of CC via targeting miR-30c-5p/TCF7 axis (18). By acting as miR sponges, RNA-binding protein sponges, or even coding polypeptides or proteins, circRNAs participate in biological processes involved in tumor cell proliferation, invasion, metastasis, apoptosis, and epithelial-to-mesenchymal transition. A previous study indicated that circRNA_0000285 promoted the proliferation and metastasis of cervical cancer cells by upregulating FUS, which was considered as a potential therapeutic target for cervical cancer (19). CircRNA_002178 can enhance programmed death-ligand 1 expression by sponging miR-34 to induce T-cell failure in lung adenocarcinoma cells (20). In addition, circRNA_002178 can be detected in plasma exosomes of patients with lung adenocarcinoma and can be used as a biomarker for early diagnosis of lung adenocarcinoma (20). CircZFR is a novel circRNA discovered in recent years, which is involved in the progression of various cancer types (21). In NSCLC, the expression of circZFR is significantly increased, and knockdown of circZFR can play a positive role in overcoming paclitaxel (PTX) resistance by regulating the miR-195-5p/KPNA4 axis (22). CircZFR expression was distinctly augmented in esophageal squamous cell carcinoma tissues and cells, and it promoted the proliferation, migration, and invasion of esophageal squamous cell carcinoma cells through the absorption of miR-377 (23). In the present study, the expression of circZFR was also found to be abnormally elevated in CC cell lines. Subsequently, its expression was knocked down in CC cells and the data indicated that inhibition of circZFR expression impeded CC cell proliferation and facilitated G1/S cell cycle arrest and apoptosis. These results suggested that downregulation of circZFR expression might serve as a potential tumor suppressor in CC cells. The potential role of circZFR as a marker in the diagnosis, treatment, and biological progression of CC has also been highlighted.
By using the ENCORI database, it was predicted that circZFR and miR-147a could bind to each other. This binding was confirmed by luciferase reporter gene assays and other experimental models. Moreover, miR-147a levels were significantly increased following inhibition of circZFR expression. It has also been shown that hnRNPK-regulated LINC00263 plays an important role in the progression of tumor malignancy by acting as a miR-147a decoy in CC cells, which in turn causes upregulation of CAPN2 expression. These results indicated that miR-147a expression was significantly decreased in CC cell lines, which was consistent with the results of the present study.
Subsequently, the ENCORI database predicted that CACUL1 could bind to miR-147a. The regulatory association between these 2 molecules was confirmed by luciferase reporter assays. The expression levels of CACUL1 in CC tissues were significantly higher compared with those noted in normal tissues. In addition, miR-199a-5p has been shown to regulate CACUL1 expression and to act as a tumor suppressor in CC. In the present study, the results indicated that the expression levels of CACUL1 in CC cell lines were significantly increased. Knockdown of circZFR expression upregulated miR-147a and inhibited the expression of CACUL1, thereby inhibiting the proliferation of CC cells and inducing cell cycle arrest and apoptosis. Furthermore, the results demonstrated that CACUL1 might play a regulatory role as a tumor promoter in CC.
The present study has certain limitations. The experiments performed were based on in vitro experimental models and did not include analysis of circZFR and miR-147a expression levels in specimens of patients with CC. The expression levels of these 2 molecules in patients with CC will be assessed in subsequent experiments. Also, further functional research of circZFR/miR-147a/CACUL1 axis in CC needs to be supplemented. Whether signaling pathways are involved in the regulatory mechanism of circZFR in CC also demands exploration. In addition, the regulatory mechanism of circZFR will be examined in animal models in future studies. In conclusion, the present study demonstrated that circZFR promoted the progression of CC by arresting cell cycle progression, and inducing apoptosis. The ceRNA regulatory network of circZFR could target miR-147a and CACUL1, which indicated that they could serve as biomarkers for the targeted therapy and prognosis among CC patients. The findings of the present study may provide a theoretical basis for the involvement of circZFR in CC progression.
Acknowledgments
Funding: The study was supported by Shaanxi Provincial Science and Technology Plan for 2020 (No. 2020JM-369) and Beijing Science and Technology Innovation Medical Development Foundation (No. KC2021-JX-0186-88).
Footnote
Reporting Checklist: The authors have completed the MDAR reporting checklist. Available at https://jgo.amegroups.com/article/view/10.21037/jgo-22-672/rc
Data Sharing Statement: Available at https://jgo.amegroups.com/article/view/10.21037/jgo-22-672/dss
Conflicts of Interest: All authors have completed the ICMJE uniform disclosure form (available at https://jgo.amegroups.com/article/view/10.21037/jgo-22-672/coif). All authors report that they received funding from Shaanxi Provincial Science and Technology Plan for 2020 (No. 2020JM-369) and Beijing Science and Technology Innovation Medical Development Foundation (No. KC2021-JX-0186-88). The authors have no other conflicts of interest to declare.
Ethical Statement: The authors are accountable for all aspects of the work in ensuring that questions related to the accuracy or integrity of any part of the work are appropriately investigated and resolved.
Open Access Statement: This is an Open Access article distributed in accordance with the Creative Commons Attribution-NonCommercial-NoDerivs 4.0 International License (CC BY-NC-ND 4.0), which permits the non-commercial replication and distribution of the article with the strict proviso that no changes or edits are made and the original work is properly cited (including links to both the formal publication through the relevant DOI and the license). See: https://creativecommons.org/licenses/by-nc-nd/4.0/.
References
- Bray F, Ferlay J, Soerjomataram I, et al. Global cancer statistics 2018: GLOBOCAN estimates of incidence and mortality worldwide for 36 cancers in 185 countries. CA Cancer J Clin 2018;68:394-424. [Crossref] [PubMed]
- Ba S, Xuan Y, Long ZW, et al. MicroRNA-27a Promotes the Proliferation and Invasiveness of Colon Cancer Cells by Targeting SFRP1 through the Wnt/β-Catenin Signaling Pathway. Cell Physiol Biochem 2017;42:1920-33. Retracted in: Cell Physiol Biochem 2021;55:140. [Crossref] [PubMed]
- Chen J, Gu J, Tang M, et al. Regulation of cancer progression by circRNA and functional proteins. J Cell Physiol 2022;237:373-88. [Crossref] [PubMed]
- Panda AC, Abdelmohsen K, Gorospe M. RT-qPCR Detection of Senescence-Associated Circular RNAs. Methods Mol Biol 2017;1534:79-87. [Crossref] [PubMed]
- Tang X, Ren H, Guo M, et al. Review on circular RNAs and new insights into their roles in cancer. Comput Struct Biotechnol J 2021;19:910-28. [Crossref] [PubMed]
- Liu T, Liu S, Xu Y, et al. Circular RNA-ZFR Inhibited Cell Proliferation and Promoted Apoptosis in Gastric Cancer by Sponging miR-130a/miR-107 and Modulating PTEN. Cancer Res Treat 2018;50:1396-417. [Crossref] [PubMed]
- Luo L, Miao P, Ming Y, et al. Circ-ZFR Promotes Progression of Bladder Cancer by Upregulating WNT5A Via Sponging miR-545 and miR-1270. Front Oncol 2020;10:596623. [Crossref] [PubMed]
- Khan S, Jha A, Panda AC, et al. Cancer-Associated circRNA-miRNA-mRNA Regulatory Networks: A Meta-Analysis. Front Mol Biosci 2021;8:671309. [Crossref] [PubMed]
- Uzuner E, Ulu GT, Gürler SB, et al. The Role of MiRNA in Cancer: Pathogenesis, Diagnosis, and Treatment. Methods Mol Biol 2022;2257:375-422. [Crossref] [PubMed]
- Lu Y, Luan XR. miR-147a suppresses the metastasis of non-small-cell lung cancer by targeting CCL5. J Int Med Res 2020;48:300060519883098. [Crossref] [PubMed]
- Ma Z, Cai Y, Zhang L, et al. LINC00319 Promotes Cervical Cancer Progression Via Targeting miR-147a/IGF1R Pathway. Cancer Biother Radiopharm 2020; Epub ahead of print. [Crossref] [PubMed]
- Ding J, Wang Q, Guo N, et al. CircRNA circ_0072995 promotes the progression of epithelial ovarian cancer by modulating miR-147a/CDK6 axis. Aging (Albany NY) 2020;12:17209-23. [Crossref] [PubMed]
- Chen N, Kong Y, Wu Y, et al. CAC1 knockdown reverses drug resistance through the downregulation of P-gp and MRP-1 expression in colorectal cancer. PLoS One 2019;14:e0222035. [Crossref] [PubMed]
- Tan Z, Cao F, Jia B, et al. Circ_0072088 promotes the development of non-small cell lung cancer via the miR-377-5p/NOVA2 axis. Thorac Cancer 2020;11:2224-36. [Crossref] [PubMed]
- Jang MJ, Park UH, Kim JW, et al. CACUL1 reciprocally regulates SIRT1 and LSD1 to repress PPARγ and inhibit adipogenesis. Cell Death Dis 2017;8:3201. [Crossref] [PubMed]
- Livak KJ, Schmittgen TD. Analysis of relative gene expression data using real-time quantitative PCR and the 2(-Delta Delta C(T)) Method. Methods 2001;25:402-8. [Crossref] [PubMed]
- Xie H, Ren X, Xin S, et al. Emerging roles of circRNA_001569 targeting miR-145 in the proliferation and invasion of colorectal cancer. Oncotarget 2016;7:26680-91. [Crossref] [PubMed]
- Guo Y, Guo Y, Chen C, et al. Circ3823 contributes to growth, metastasis and angiogenesis of colorectal cancer: involvement of miR-30c-5p/TCF7 axis. Mol Cancer 2021;20:93. [Crossref] [PubMed]
- Chen RX, Liu HL, Yang LL, et al. Circular RNA circRNA_0000285 promotes cervical cancer development by regulating FUS. Eur Rev Med Pharmacol Sci 2019;23:8771-8. [PubMed]
- Wang J, Zhao X, Wang Y, et al. circRNA-002178 act as a ceRNA to promote PDL1/PD1 expression in lung adenocarcinoma. Cell Death Dis 2020;11:32. [Crossref] [PubMed]
- Liu L, Wang H, Yu S, et al. An Update on the Roles of circRNA-ZFR in Human Malignant Tumors. Front Cell Dev Biol 2021;9:806181. [Crossref] [PubMed]
- Li J, Fan R, Xiao H. Circ_ZFR contributes to the paclitaxel resistance and progression of non-small cell lung cancer by upregulating KPNA4 through sponging miR-195-5p. Cancer Cell Int 2021;21:15. [Crossref] [PubMed]
- Fang N, Shi Y, Fan Y, et al. Circ_0072088 Promotes Proliferation, Migration, and Invasion of Esophageal Squamous Cell Cancer by Absorbing miR-377. J Oncol 2020;2020:8967126. [Crossref] [PubMed]
(English Language Editor: C. Betlazar-Maseh)