PD-L1 testing in advanced gastric cancer—what physicians who treat this disease must know—a literature review
Introduction
Gastric cancer (GC) is the fifth most diagnosed cancer worldwide, and the fourth most lethal (1). Although systemic chemotherapy is the mainstay therapeutic strategy in metastatic GC, immunotherapy has recently modified the treatment landscape of the advanced disease. Based on remarkable findings from randomized clinical trials, immune checkpoint inhibitors (ICI), either alone or combined with chemotherapy, have been recently approved in several countries (2,3). However, the benefit of immunotherapy is far from being universal in GC and the identification of predictive biomarkers to assist in the selection of patients that might derive benefit from ICI is an unmet clinical need.
Immune evasion is one of the hallmarks of cancer. Cancer cells may evade host immunity in the tumor microenvironment by expression of immune inhibitory signaling proteins. Programmed cell death-1 (PD-1) and cytotoxic T-lymphocyte–associated antigen-4 (CTLA-4) are the principal immune checkpoints, and their blockade has led to a new era in cancer therapy. Several studies have shown that immune checkpoint blockade enhances T-cell response and mediates antitumor activity in several solid tumors (4,5).
It is estimated that 55% to 66% of the patients with advanced GC express programmed cell death-ligand 1 (PD-L1) (6,7). Randomized clinical trials have consistently shown that PD-L1-positive patients are more likely to respond to PD-1/PD-L1 inhibitors compared to the negative counterparts (7,8). Therefore, PD-L1, together with MSI, have become the most adopted biomarkers for selecting possible candidates for anti-PD-1 therapy. However, many questions remain unanswered. To date, the best diagnostic assay, PD-L1 expression cutoff and score for predicting ICI response have not been completely defined. In addition, PD-L1 expression has demonstrated relatively high inter- and intratumoral heterogeneity and interobserver variability (9).
As such, we conducted a broad search on PubMed and also abstract published in ASCO, ASCO GI, ESMO and ESMO GI annual meetings. For our search on English language articles and abstracts were considered using the terms “immunotherapy AND gastric cancer”; “immunotherapy AND gastroesophageal cancer”; “PD-L1 AND gastric cancer”; and “PD-L1 AND gastroesophageal cancer” as described in Table 1.
Table 1
Items | Specification |
---|---|
Date of search | 14th and 15th of September of 2021 (preliminary initial search); additional searches were conducted along the review construction and writing process |
Databases and other sources searched | PubMed; abstracts published in ASCO, ASCO GI, ESMO and ESMO GI annual meetings |
Search terms used | “immunotherapy AND gastric cancer”; “immunotherapy AND gastroesophageal cancer”; “PD-L1 AND gastric cancer”; and “PD-L1 AND gastroesophageal cancer” |
Timeframe | 2015–2022 |
Inclusion and exclusion criteria | English language, prospective and retrospective studies as well as case reports |
Selection process | All authors conducted the literature review search and selection |
In this review, we aim to address the advantages and disadvantages of adopting PD-L1 as a biomarker for predicting response to immunotherapy in GC, as well as to endeavor to establish how to better implement its use in clinical practice. We present this article in accordance with the Narrative Review reporting checklist (available at https://jgo.amegroups.com/article/view/10.21037/jgo-22-1133/rc).
Methods
Immunology and microenvironment in GC
Immune checkpoints
One of the main checkpoint pathways is mediated by the PD-1 and its ligand, PD-L1 (Figure 1). PD-1 is a co-inhibitory receptor mainly expressed by activated T cells, but also by B cells, dendritic cells, and natural killer (NK) cells, while PD-L1 is expressed on several different types of cells, including tumor cells (10,11). Naïve T cells are presented with antigens by the major histocompatibility complex (MHC) on the surface of cancer cells through their T-cell receptor (TCR). However, a single initial signal proves to be insufficient to initiate a T-cell response, and, as such, a second signal delivered by the B7 costimulatory molecules is necessary. Following T-cell activation, cytotoxic T lymphocyte-associated antigen (CTLA)-4 is then up-regulated and subsequently initiates negative regulation signaling on T cells during association with B7 molecules expressed by antigen-presenting cells (12). As said molecules bind to CD28, activation signals are fired; binding to CTLA-4, and providing inhibitory signals. Anti-CTLA-4, such as ipilimumab and tremelimumab, are monoclonal antibodies that prevent the interactivity between CTLA-4 and its ligands B7.1 and B7.2, enhancing, therefore, anti-tumor immune responses (13). The linkage between CTLA-4 and costimulatory molecules occur mainly in the initial phase of T-cell response within lymph nodes (Figure 1).
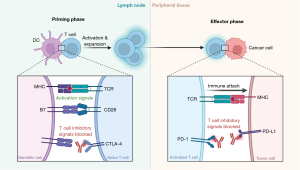
T-cells express PD-1 inhibitory receptor throughout long-term antigen exposure which results in negative regulation of T cells while ligation with PD-L1 and PD-L2 occurs, primarily expressed both in tumor microenvironment and inflamed tissues (12). Anti-PD-1, such as nivolumab and pembrolizumab, and anti-PD-L1, such as atezolizumab, avelumab and durvalumab, are monoclonal antibodies acting on the blockage of PD-1 and PD-L1, respectively (13). The obstruction of the interaction between PD-1 and its ligands PD-L1/L2 actives T-cell-mediated antitumor response. PD-1 interaction occurs in the effector phase of a T-cell response in peripheral tissues (14) (Figure 1).
Microenvironment and GC
The tumor microenvironment in GC is vital in propelling cell growth, defense against host immune mechanisms and treatment resistance. The array of cells in the milieu that favors GC progression include modified fibroblasts, known as cancer-associated fibroblasts (CAFs), immune cells, gastric myofibroblasts and endothelial cells, among others (Figure 2).
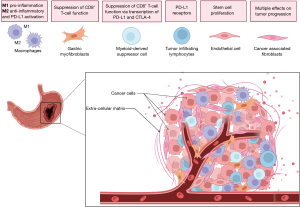
CAFs
CAFs are the dominant non-cancerous cell-type in GC stroma and play a central role in the tumorigenesis of the disease. The stroma in non-neoplastic tissues characteristically displays a limited number of fibroblasts. Typical CAFs are much more abundant in cancer environment and bear a strong expression of α-smooth muscle actin (α-SMA), which is a conspicuous marker of activated fibroblasts (15). The origin of CAFs is still debatable. Analysis in single-cell experiments have shown molecular heterogeneity in the CAFs compartment. This heterogeneity results in different protein expression pattern with consequent multiple effects on tumor progression (16). The vast heterogeneity of CAFs also suggests an origin from different cells. CAFs origin have been traced to bone marrow mesenchymal stem cells (MSCs), local normal fibroblasts, and local pericytes (17). A very interesting finding is that normal gastric myofibroblasts actively participate in the antrum stem cell development and differentiation through secretion of R-spondin3 (18). R-spondin3, in turn, increases the expression of Axin-2 in gastric stem cells which leads to a rapid proliferation of this niche and the generation of CAFs (19). Therefore, antrum myofibroblasts, via stem cell activation, are an alternative source of CAFs.
AFs genetic programming is very distinct from normal fibroblasts. Studies in mice and humans revealed genomic differences in several cancers (20). An active cross-talk among the tumor and the stroma modifies the gene expression of GC cells through soluble molecules in a paracrine fashion, such as basic fibroblast growth factor (bFGF), vascular endothelial growth factor (VEGF), platelet-derived growth factor (PDGF), epidermal growth factor receptor (EGFR) ligands, interleukins, and TGF-β (21). In a study with gastric cancer cell lines, CAFs promoted the growth of tumor cells by increased PD-L1 expression (22).
Endothelial cells
Supply of nutrition for cancer tissue growth is a well-known function provided by neo-angiogenesis. However, endothelial cells of tumor neovasculature have been shown to display a central role as a carcinogenetic niche (23). Endothelial cells are also able to stimulate paracrine mechanisms that activate Akt and Notch signaling in cancer progenitor cells (24). Recent research on fundic GC stem cells revealed a potential targetable mechanism involving the GC niche and the endothelial cells. Cancer endothelial cells are able to secrete CXCL12 which, in turn, activates CXCR4 signaling in innate lymphoid cells, creating an inflammatory cascade which leads to enhanced WNT expression in gastric isthmus stem cells. The continuous functioning of the synapsis CXCL12 endothelial cells/CXCR4 ILC is a powerful stimulus to stem cell proliferation in the cancer niche. The interruption of CXCL12/CXCR4 signaling interferes with cancer growth and metastasis initiation and progression (24,25). This complex regulatory mechanism was well reviewed by Oya et al. (26).
Ramucirumab, an antiangiogenic commonly used in the second-line setting of metastatic GC, binds to VEGFR2, blocks VEGF/VEGFR2 interaction, and inhibits VEGF-stimulated receptor phosphorylation in endothelial cell, leading to disruption of downstream signaling (27). In an interesting study with 20 GC patients, both PD-L1 expression and CD8+ T-cell infiltration increased after ramucirumab-containing therapies.
Macrophages
The vast and polymorphic group of immune cells may acquire specific characteristics in the GC microenvironment. Normal antigen presenting cells, such as macrophages, modify their biological programming and become cells called tumor-associated macrophages (TAMs). They are the most common immune cells in the cell-cancer niche and their infiltration in the tumor microenvironment can be used as prognostic index in several types of cancers (26). Tumor macrophages may be categorized by the pattern of immune response associated with them. The M1 macrophages display pro-inflammatory properties and produce various cytokines and chemokines, such as IL-12, CXCL8, CXCL9, and CXCL10, which recruit TH1 cells and amplify a type 1 response (28). Other important immune effects are postulated for M1 cells, including the recruitment of myeloid-derived suppressor cells (MDSC) by secretion of IL-1β and TNF-α (29,30) and the release of nitric oxide synthase (NOS) and/or reactive oxygen species (ROS) in the cancer niche, increasing the genetic damage in the gastric cells (31). The majority of macrophages in GC stroma are called M2. These cells are responsible for the production of anti-inflammatory cytokines, including TGF-β and IL-10. They are also involved in TH2 immune response and PD-L1 activation (via IL-10) (28,32-34).
A study analyzed fresh tumor tissues from 76 patients with GC and demonstrated that high level of CXCL8 was associated with decreased CD8+ T cells infiltration. CXCL8 inhibited CD8+ T cells function by inducing the expression of PD-L1 on macrophages (35).
MDSCs
MDSCs designate a group of myeloid progenitor and immature myeloid cells with properties of suppressing CD8+ T-cell function via transcription of PD-L1 and CTLA-4 proteins. They are considered one of the major immune suppressive cell populations in the tumor microenvironment, and therefore, MDSC in GC can be a very attractive target (36). It has been postulated that targeting MDSCs could potentiate PD-L1 blockade efficacy in GC (36). For instance, in murine models, the reduction of MDSC in the cancer microenvironment leads to an increase of intratumoral CD8+ T cells leading to apoptosis (37,38).
Lymphocytes
The adoptive anti-tumor immunity is performed mainly by a group of T-cells and B-cells known as tumor-infiltrating lymphocytes (TILs). In the GC microenvironment, the central component of anti-tumor response, T-cells targeted to cancer neoantigens, are progressively hampered by the upregulation of PD-L1 or CTLA-4, which create a state of anergy in these previously effective anti-tumor cells. This effect is mediated by NF-κB1 lack of expression leading to an excessive JAK-STAT signaling, a pathway that plays important roles in orchestrating of immune system, especially cytokine receptors (39). This, in turn, affects the main pathway by which T-cells recognize and establish an immune response, such as antigen presentation and dysregulation of immune checkpoints. The final result is the upregulation of PD-L1 in lymphocytes and other cells that work in support of the immune response (40). Therefore, it is not surprising that PD-L1 hyperexpression in GC relates to a shorter survival (41). A specific type of CD4+ T-cells, called regulatory T-cells, which are phenotypically identified as FOXP3+, CD25, GITR, Nrp1, Helios and CTLA-4, play an important role in inducing anergy and lack of effectiveness of TILs. They suppress T-cell proliferation and antigen presentation (42).
PD-L1 testing in gastric cancer
A number of clinical trials involving patients receiving ICIs have assessed the predictive value of PD-L1 expression on tumor cells and tumor-infiltrating immune cells (43,44). The evaluation of extent of PD-L1 expression may be occurs by two main methods: the tumor proportion score (TPS) and the combined positive score (CPS) (45). TPS is defined as the number of positive tumor cells divided by the total number of viable tumor cells multiplied by 100. Meanwhile, CPS is defined as the number of positive tumor cells, lymphocytes and macrophages, divided by the total number of viable tumor cells multiplied by 100 (46).
On account of the fact that PD-L1 expression in both the tumor cells and microenvironment cells (lymphocytes, monocytes and other components) impact on immunosuppression, it is possible that a combined PD-L1 score presents a stronger predictive value for immune checkpoint inhibition in gastroesophageal adenocarcinoma (GEA) (44,47). In addition, TPS assessment may be slightly inaccurate due to difficulties in distinguishing poorly differentiated adenocarcinoma cells from macrophages, both expressing PD-L1 (48). In a Japanese study, 191 GC patients who underwent curative gastrectomy had their tumors analyzed by double immunohistochemistry (IHC) of PD-L1 and ionized calcium binding adaptor molecule 1 (to distinguish PD-L1 expression between tumor cells and macrophages). PD-L1 positivity was detected in only 39 patients (20.4%) by TPS and in 137 patients (71.7%) by CPS (48). These results indicate that CPS has higher sensitivity. Therefore, TPS has been considered inadequate and CPS is currently the most suitable score for interpreting PD-L1 expression in GEA (45,49).
A number of PD-L1 IHC assays have been validated in different solid tumors in order to guide patient selection for treatment with immunotherapy (43,44). For instance, currently four PD-L1 IHC assays are registered in the Food and Drug Administration (FDA) with four different PD-L1 antibodies (22C3, 28-8, SP263, SP142) on two different IHC platforms (Dako and Ventana), with unique scoring systems. In GEA, the antibodies 22C3 and 28-8 have been used in the studies evaluating pembrolizumab and nivolumab, respectively (6-8,50). Inter-assay concordance between the 22C3 and 28-8 assays is uncertain, and discordant results have been reported (51-54). Caution is recommended when comparing the assays until stronger evidence is found.
The assessment of PD-L1 expression should be conducted by an experienced pathologist. Inadequate amount of tumor tissue on the biopsy specimen or tissue damage during manipulation may lead to false-negative results. A minimum of 100 viable tumor cells must be present in the PD-L1 stained slide for the specimen to be considered suitable for PD-L1 evaluation (55).
Another point of interest is the concordance rate of PD-L1 expression between primary tumors and metastases. Previous studies reported some inconsistency in the PD-L1 expression between primary tumor and metastases in other solid tumors. However, there are few studies in GC, possibly due to infrequent biopsies or resections of metastases in routine clinical practice (56-60). Data initially presented in 2019 from the comparison of PD-L1 expression in 30 primary GC samples matched with metastases showed equivalence between them, except for one pair (61). However, these findings have not been consistent in subsequent studies. A recently published study with 23 primary tumor samples paired with metastatic sites showed PD-L1 positivity in 52.2% of primary tumors versus 4.3% of metastases, even in the 16 patients with metachronous disease (56). Yet another recently published study also support this spatial heterogeneity. Pairing 62 samples of primary tumors with their respective metastases demonstrated a concordance rate of 61% by CPS. However, the rate increased to 88% if the primary tumors were PD-L1-negative, which suggests higher negative predictive value of the test (62). Likewise, meaningful temporal heterogeneity was noted. In the same study, comparison of the primary tumors from 83 patients with their metastases before and after chemotherapy revealed a concordance rate of only 57%. Three patients had conversion from PD-L1 negative to PD-L1 positive status after chemotherapy (62). Indeed, cytotoxic chemotherapies are known to remodel the tumor–immune microenvironment (63).
Intratumoral heterogeneity may also contribute to the low concordance rate in the PD-L1 assessment. When the analysis is done in biopsy specimens, it is recommended at least 4 to 5 fragments for an adequate interpretation (64,65). Interestingly, previous studies in GC patients showed higher PD-L1 expression in nodal metastases compared to the primary tumor: 54.4% versus 41.6% in a study with 174 patients (66), and 60.5% versus 41.9% in another study with 43 patients (67).
The higher expression observed in the primary tumor compared to the distant metastases could be due to tumor cell evasion from the immune system and the selective pressure that occurs during the disease progression with systemic treatment. As for the discrepancy between the primary tumor and regional nodal metastases, it might reflect the distinct biological behavior of lymphatic (versus hematogenous) dissemination, or simply the diversity of tests applied to assess PD-L1 expression in these studies.
Molecular subgroups of gastric cancer, tumor mutational burden and their relationship with PD-L1 expression
Four molecular subgroups of gastric cancer have been described: tumors positive for Epstein-Barr virus (EBV+), microsatellite unstable (MSI-H) tumors, genomically stable tumors, and tumors with chromosomal instability (68). Both EBV+ and MSI-H tumors are shown to present higher sensitivity to immunotherapy, probably due to their CD8+ T-cell rich microenvironment (7,69,70). In addition, those GC subtypes are more likely to express PD-L1 (71). Higher PD-L1 expression in EBV+ GC is also related to either high focal amplification of CD274 or IFN-γ-mediated signaling via activation of interferon regulatory factor 3 (IRF3) (72).
Mismatch repair (MMR) genes (MLH-1, PMS-2, MSH-2 and MSH-6) are paramount players in DNA repair pathways. MMR deficiency results from the loss of function of these gene products, leading to alterations in the size of microsatellites, a phenomenon known as MSI. MSI-H tumors have a higher frequency of frameshift mutations which generate a significant number of neoantigens, conferring a stronger immunogenicity (73). In some tumors, a strong correlation between MSI-H and high tumor mutational burden (TMB, defined by the number of mutations per megabase (muts/Mb) harbored by tumor cells in a given neoplasm) has been shown (74). Nevertheless, not all MSI-H tumors exhibit an increased TMB while microsatellite stable (MSS) tumors may harbor high TMB.
MSI-H status has been linked to long-term response to ICIs and improved prognosis in several malignancies, including GC (75). However, a subset of patients with MSI-H GC do not respond to ICIs and some genomic alterations have been previously associated with innate anti-PD-1 resistance in those cases (76). In an interesting phase II study, 19 MSI-H GC patients were treated with pembrolizumab in either second- or third-line therapy (76). Among them, 14 patients (87.5%) had CPS ≥1. The authors reported a greater benefit for patients with higher TMB, whereas PD-L1 did not influence on response (76).
A meta-analysis including the phase III trials KEYNOTE-062, CheckMate-649, JAVELIN Gastric 100 and KEYNOTE-061 evaluated the predictive role of MSI-H in GC patients treated with ICIs. MSI-H was reported in 4.8% of the cases and the HR for OS benefit with anti-PD-1-based regimens was 0.34 (95% CI: 0.21–0.54) for MSI-high GC versus 0.85 (95% CI: 0.71–1.00) for MSS tumors (75).
Several studies have consistently shown that TMB is also an independent biomarker of response to immunotherapy across different cancer types (77,78). Indeed, pembrolizumab received accelerated agnostic approval for the treatment of advanced solid tumors with high-TMB, although this decision has been debated and understanding the role of this biomarker in specific tumor types seems crucial (79,80).
TMB has been specifically studied in GC (68,81-83), but its predictive value for immunotherapy benefit has been controversial, especially among microsatellite stable tumors. Study with 80 GC patients failed to demonstrate correlation between TMB, calculated based on a panel sequencing, and response to immunotherapy (84). This finding could possibly be related to the method used in the study, since it has been already shown that this correlation could be more safely done in hypermutated tumors (85), which is not the case of GC (86). These findings raise the importance of better understanding the appropriated method of quantifying mutations. Whole exome sequencing is expensive; however, it might be necessary to properly evaluate TMB in GC.
In addition, a retrospective cohort with 1,678 patients with 16 different tumor types (including 67 GC patients, all of them microsatellite stable) treated with ICIs investigated the predictive value of TMB in terms of response to immunotherapy (87). Although, in general, tumors with TMB ≥10 mutations per megabase (considered TMB-high) presented better response rates with ICIs, such association was not demonstrated in GC. Indeed, among the 67 GC patients, only 5 were TMB-high. Response rates were 20% and 31% for TMB-high and TMB-low GC patients, respectively (87).
CPS is the most commonly used biomarker for immunotherapy in GC and, therefore, it is important to understand the relationship between CPS and TMB. A positive correlation between TMB and PD-L1 expression has been shown in a study including over 48,000 cancer cases, most of them of non-small cell lung cancer (88). A different finding was shown in a study with 6,668 advanced solid tumor specimens, with no significant correlation in most cancer types, though surprisingly with a positive, but weak association of PD-L1 and TMB in proficient MMR GC (89). Interestingly, smaller pan-cancer study has also demonstrated positive association between PD-L1 and TMB, specifically in gastric and endometrial cancer (90). Studies involving only advanced GC patients show different findings regarding this relationship. Study with 63 patients with advanced GC showed a positive relation between TMB and CPS (78). However, low correlation between TMB and CPS was seen in other studies. In the KEYNOTE-061 trial, 592 patients with GC were evaluated by whole exome sequencing (6) and low correlation between TMB and CPS in both immunotherapy and chemotherapy treatment groups were demonstrated (82).
Challenges in using TMB as a biomarker still remain, considering that there are different methods of assessing the number of mutations and cut-off points in the studies (47,49). What is clear, though, is that TMB must be better investigated as a biomarker and further studies are needed to better understand how it can be more consistently used in our daily practice in order to offer immunotherapy to the appropriated patients. Interestingly, studies have shown no correlation of high TMB status and EBV+ tumors, but a strong correlation of TMB-high with MSI-H GC (7,47).
Efficacy of immunotherapy according to PD-L1 expression in GC
Over the last few years, the landscape of immunotherapy in GC has showed remarkable progress. Considering the clinical trials which have addressed the efficacy of ICIs, PD-L1 expression emerges as a predictive biomarker of clinical benefit, despite some limitations (2,6,8,50,91-97) (Table 2).
Table 2
Acronym/reference/PD-L1 evaluation | Population | Intervention | Comparator | Outcome, HR (95% CI) | Comments |
---|---|---|---|---|---|
CheckMate-032, Phase I/II (79), TPS | Chemotherapy-refractory gastric, esophageal, or GEJ; USA and Europe (n=160) | Nivolumab or Nivolumab plus ipilimumab, in different doses | NA | ORR - NIVO3: 12% (5–23%) - NIVO1 + IPI3: 24% (13–39%) - NIVO3 + IPI1: 8% (2–19%) |
- Primary endpoint: ORR - PD-L1 (+ vs. -): ORR 13×4% - PD-L1 scoring: TC ≥1% |
KEYNOTE-059, Phase II, cohort 1 (81), CPS | Gastric or GEJ >2 lines; 17 countries including East Asia (n=259) | Pembrolizumab monotherapy | NA | ORR 11.6% (8.0–16.1%) | - Primary endpoint: ORR and safety - PD-L1 (+ vs. -): ORR 15.5×6.4% - PD-L1 (+ vs. -) 3rd line: ORR 22.7×8.6% - PD-L1 scoring: CPS ≥1% |
ATTRACTION-2, Phase III (76), CPS | Gastric or GEJ, >2 lines (refractivity or intolerance); Asia (n=493) | Nivolumab monotherapy | Placebo | OS 5.2×4.1 m, HR 0.63 (0.51–0.78; P<0.0001) | - Double-blind - Primary endpoint: OS - PD-L1+: OS 5.2×3.8 m, HR 0.75 (0.32–1.72) - PDL1-: OS 6.0×4.0 m, HR 0.70 (0.50–0.99) -PD-L1 scoring: TC ≥1% |
JAVELIN, Gastric 300, Phase III (82), CPS | Gastric or GEJ, 3rd line; global (n=371) | Avelumab monotherapy | Physician’s choice chemotherapy (paclitaxel, irinotecan or BSC) | OS 4.6×5.0 m, HR 1.1 (0.9–1.4; P=0.81) | - Open label - Primary endpoint: OS - PD-L1 (+ vs. −): OS 4.0×4.6 m - PD-L1 scoring: TC ≥1% |
KEYNOTE-061, Phase III (2,83), CPS | Gastric or GEJ, >1st line; global (n=395); enrollment restricted to CPS ≥1% after first interim analysis | Pembrolizumab monotherapy | Paclitaxel monotherapy | - OS 9.1×8.3 m, HR 0.82 (0.66–1.00) - PFS 1.5×4.1 m, HR 1.27 (1.03–1.57) |
- Open label - Primary endpoint: OS and PFS in CPS ≥1% - Exploratory data: CPS ≥5% OS 10.4×8.3 m HR 0.72; 95% (0.53–0.99); CPS ≥10% OS 10.4×8.3 m, HR 0.69; 95% (0.46–1.05) |
KEYNOTE-059, Phase II, cohort 2 (84), CPS | Gastric or GEJ 1st line; Japan, South Korea, USA, France and Israel (n=25) | Pembrolizumab plus cisplatin and 5-FU (capecitabine instead of 5-FU in Japan) | NA | ORR 60% (39–79%) | - Primary endpoint: safety and tolerability - ORR secondary endpoint - PD-L1 (+ vs. −): ORR 68.8×37.5%; OS 19.8×11.1 m - PD-L1 scoring: CPS ≥1% |
KEYNOTE-059, Phase II, cohort 3 (84), CPS | Gastric or GEJ CPS ≥1%, 1st line; Japan, South Korea, USA, Israel, Canada and Chile (n=31) | Pembrolizumab monotherapy | NA | ORR 25.8% (11.9–44.6%) | - Primary endpoints: ORR, safety and tolerability - OS 20.7 m |
KEYNOTE-062, Phase 3 (38), CPS | Gastric or GEJ CPS ≥1%, 1st line; global (n=763) | Pembrolizumab monotherapy, or Pembrolizumab plus chemotherapy | Chemotherapy (cisplatin + 5-FU or capecitabine doublet) | - (A): Pembrolizumab vs. chemotherapy: OS 10.6×11.1 m, HR 0.91 (0.69–1.18), non-inferiority met (A) - (B): Pembro+chemo vs. chemo: OS 12.5×11.1 m, HR 0.85 (0.70–1.03; P=0.05), not superior |
- Partially blinded - Primary endpoint: A: OS pembro vs. chemo (non-inferiority); B: pembro+chemo vs. chemo (superiority) - CPS ≥10%; A: OS 17.4×10.8 m; HR, 0.69 (0.49-0.97; P not tested); B: OS 12.3×10.8 m, HR, 0.85; (0.62–1.17; P=0.16) |
JAVELIN, Gastric 100, Phase III (85), CPS | Gastric or GEJ, who achieved ORR after 12 weeks of 1st line (FOLFOX or XELOX); global (n=499) | Avelumab switch maintenance as monotherapy | Continuation of first-line chemotherapy or BSC | - ITT population: OS 10.4×10.9, HR 0.91 (0.74–1.11; P=0.177) - PD-L1+ population (n=54), HR 1.13 (0.57–2.23; P=0.63) |
- Open label - Primary endpoint: OS after induction chemotherapy in all patients or PD-L1+ (here, ≥1% by 73-10 IHC assays) - Exploratory analysis CPS ≥1% (n=137): OS 14.9×11.6 m, HR 0.72 (0.49 to 1.05) |
CheckMate 649, Phase III (39,87), CPS | Gastric or GEJ, or esophageal adenocarcinoma, 1st line; global (n=1,581) | Nivolumab plus chemotherapy or nivolumabe plus ipilimumab | Chemotherapy (CAPOX or FOLFOX) | OS: 14.4×11.1 m, HR 0.70 (0.60–0.81; P<0.0001); PFS: 8.1×6.1 m, HR 0.68 (0.56–0.81; P<0.0001) |
- Open label - Primary outcome: OS or PFS, in patients CPS ≥5% nivo+chemo vs. chemo - All randomly assigned patients: OS 13.8×11.6, HR 0.79 (0.71–0.88; P=0.0002) - Additional results also showed better OS and PFS in CPS ≥1% |
ATTRACTION-4, Phase II/III (76), CPS | Gastric or GEJ, 1st line; Asia (n=724) | Chemotherapy (SOX or CAPOX) plus Nivolumab | Chemotherapy plus placebo | - PFS: 10.45×8.34 m, HR 0.68 (0.51–0.90; P=0.0007) - OS: 17.45×17.15 m, HR 0.90 (0.75–1.08; P=0.26) |
- Double-blind - Primary outcome: PFS and OS in the ITT population (all randomly assigned patients) - Stratified by intensity of PD-L1 expression, by IHC 28-8 pharmDx - TPS ≥1% (n=114): PFS 8.34×4.37 HR 0.80 (0.48–1.33); OS 16.56×16.62 HR 1.06 (0.67–1.68) |
m, months; GEJ, gastroesophageal junction; HR, hazard ratio; ORR, overall response rate; OS, overall survival; PFS, progression-free survival; 5-FU, 5-Fluorouracil; SOX, Tegafur–gimeracil–oteracil potassium [S-1] plus oxaliplatin; CAPOX, capecitabine plus oxaliplatin; FOLFOX, 5-FU, leucovorin plus oxaliplatin; NIVO, nivolumab; IPI, ipilimumab; BSC, best supportive care; CPS, combine positive score; TPS, tumor proportion score; TC, tumor cells; ITT, intention to treat; IHC, immunohistochemistry.
Beyond second-line
The Asian phase III study ATTRACTION-2, which included chemo refractory GC patients, showed an increase of the median OS from 4.14 months in the placebo arm to 5.32 months in the nivolumab arm (91). In the exploratory analysis, the PD-L1-positive subgroup, defined as TPS ≥1%, did not show an improvement in OS (Table 1). However, since availability of tumor tissue was not mandatory for patient enrollment, this analysis was only possible in a small number of patients. Despite these limitations, this modest but statistically significant benefit led to the approval of nivolumab in Japan (98).
As in other primary malignancies, such as metastatic melanoma and MSI-H colorectal cancer, combination immunotherapy with nivolumab and ipilimumab was performed for advanced gastric, esophageal, and GEJ adenocarcinoma, aiming to enhance response rates (98,99). In the phase I/II Checkmate 032 trial, patients were randomized to either nivolumab monotherapy arm or nivolumab and ipilimumab in different doses. The study primary outcome, objective response rate (ORR), tended to be higher in the high dose ipilimumab arm, but there were overlapping 95% CI among the three arms (92). Interestingly, a comparison according to the PD-L1 expression, also exploratory, revealed an ORR of 13% in PD-L1 positive (TPS ≥1%) versus 4% in PD-L1 negative subgroup (92).
The first trial assessing the effect of pembrolizumab in advanced GC was part of phase 1b KEYNOTE-012 study (100). Its favorable benefit in ORR warranted further study in phase II and III trials. In the first of its three cohorts, phase II KEYNOTE 059 included 259 patients after at least 2 lines of chemotherapy from 17 countries for anti-PD-1 monotherapy. The ORR was 11.6% in the overall population. Regarding PD-L1 status, the ORR was 15.5% in PD-L1 positive (CPS ≥1) and 6.4% in PD-L1 negative patients. Considering only the third-line setting, the ORR was 22.7% versus 8.6% for PD-L1 positive and negative patients, respectively (7). Based on these data, single agent pembrolizumab was granted accelerated approval by FDA in September 2017, for patients with PD-L1 CPS ≥1 (2). More recently, after trials in first-line setting became available, this indication was voluntarily withdrawn by the pharmaceutical company.
Another immunotherapy studied beyond the second-line regimen for advanced GC was avelumab. The JAVELIN Gastric 300 was a phase III, open-label trial, comparing the anti-PD-L1 monotherapy with physician’s choice chemotherapy (paclitaxel, irinotecan, or best supportive care). However, it failed to demonstrate a significant difference in OS [4.6 versus 5.0 months, HR 1.1 (95% CI: 0.9–1.4; P=0.81)], even when analyzed according to PD-L1 expression [OS 4.0 versus 4.6 months, respectively for PD-L1 positive (TPS ≥1%) and negative] (93).
Second-line setting
Among advanced GC patients in the second-line setting, immunotherapy failed to reach its primary endpoint. Despite the amendment that restricted the enrollment to CPS ≥1, after the first interim analysis, the open-label phase III KEYNOTE-061 comparing pembrolizumab with paclitaxel, both in monotherapy, did not demonstrate difference in OS and PFS between arms (6). In an updated publication, OS was 9.1 versus 8.3 months (HR 0.82, 0.66–1.00) and PFS 1.5 versus 4.1 months (HR 1.27, 1.03–1.57) for paclitaxel versus pembrolizumab, respectively (94). In an exploratory analyses stratifying by PD-L1 expression, there was a benefit in OS for those with CPS ≥5, but not for those with CPS ≥10 (Table 1).
First-line setting
Recently, studies addressing the role of ICIs for advanced HER2-negative GC in first-line setting have been published, leading to a paradigm shift in the immunotherapy indication in this subset of GC patients. Taking advantage of the knowledge added by older studies, many of these trials included only patients whose tumors expressed PD-L1 or had pre-planned analysis stratifying patients according to the biomarker expression.
In cohort 2 of the phase II KEYNOTE-059 (n=25), which evaluated pembrolizumab in combination with chemotherapy (consisting of a cisplatin plus fluoropyrimidine doublet), an ORR as high as 60% was reported. Among PD-L1 positive (CPS ≥1) patients, ORR and OS were even higher compared to PD-L1 negative (ORR 68.8% versus 37.5%, OS 19.8 versus 11.1 months) (95). In cohort 3, which included only CPS ≥1 (n=31), pembrolizumab monotherapy reached out an ORR of 25.8% and a median OS of 20.7 months, as it has never been shown before (95).
More robust evidence comes from data of the phase III, partially blinded, KEYNOTE 062 trial, which evaluated efficacy and safety of pembrolizumab or pembrolizumab plus chemotherapy versus chemotherapy alone in the CPS ≥1 population. The chemotherapy backbone consisted of cisplatin plus fluoropyrimidine. After a median follow-up of 29.4 months, pembrolizumab monotherapy was noninferior to chemotherapy in median OS (10.6 versus 11.1 months, HR 0.91, 99.2% CI: 0.69–1.18). However, the combination of chemotherapy plus anti-PD-1, did not show superiority in terms of OS when compared to chemotherapy alone. In a subgroup analysis, patients with CPS ≥10 achieved numerically higher OS when immunotherapy was used, but no statistically significant difference was demonstrated (Table 1) (50).
In a different study design, JAVELIN Gastric 100 was an open-label phase III trial which evaluated immunotherapy in the maintenance setting, i.e., in advanced GC patient who achieved response after 12 weeks of first line chemotherapy. Comparing to best supportive care or the continuation of first-line chemotherapy, the study did not reach its primary outcome of OS, even among the PD-L1 positive (CPS ≥1) population (96).
The first immunotherapy approved by the FDA and also by regulatory agencies in other countries for the first line setting was nivolumab in combination with oxaliplatin-based chemotherapy (101). It was supported by the phase III CheckMate-649 trial (n=789), a randomized, multicenter, open-label and global study, in which GC patients, regardless of PD-L1 status, were included. Considering all randomly assigned patients, those treated in the nivolumab plus chemotherapy arm had median OS of 13.8 months, compared with 11.6 months in patients who received chemotherapy alone (8). In the CPS ≥5 population, both PFS (HR: 0.68, 0.56–0.81; P<0.0001) and OS (HR: 0.71, 0.59–0.86, P<0.0001), which were the dual primary endpoints, favored the nivolumab arm. In an exploratory analyses, unstratified HR for OS with nivolumab plus chemotherapy versus chemotherapy alone for patients with CPS <1 and <5 was 0.92 (95% CI: 0.70–1.23) and 0·94 (0.78–1.13), respectively. In addition, interaction test comparing the subgroups by CPS ≥5 was statistically significant (P=0.0107) (8). These results reflect the minimal (at most) benefit of adding nivolumab to chemotherapy in the subgroup of patients with CPS <5.
Recently, the FDA and other regulatory agencies have approved nivolumab plus chemotherapy for first-line treatment, regardless of PD-L1 expression (3,101). The higher rates of grade ≥3 adverse events (59% versus 44%) and the financial burden associated to the indiscriminate prescription of nivolumab should not be disregarded (8).
Another important trial of nivolumab for frontline advanced GC was the double-blind phase II/III ATTRACTION-4, conducted in Asia. Regarding one of its coprimary endpoint, the PFS in the ITT population (among all randomly assigned patients) were higher in the chemotherapy plus anti-PD-1 arm than in chemotherapy alone (10.5 versus 8.3 months, HR 0.68, P=0.0007). However, at the final analysis, OS was similar for both groups (HR: 0.90, 0.75–1.08, P=2.57). Among TPS ≥1% patients (n=114), OS was 16.56 months versus 16.62 months (HR 1.06, 0.67–1.68) for chemotherapy with nivolumab versus chemotherapy only, respectively (91).
Conclusions
GC is a markedly heterogenous disease whose systemic therapy is an unmet clinical need. The cornerstone of the systemic therapy is the combination of fluoropyrimidines plus platins, which yields modest clinical outcomes. Apart from trastuzumab for HER2-positive disease, there are currently no genome-guided personalized therapies in GC, despite promising novel therapies in the next few years. ICI has demonstrated modest activity in an unselected population of advanced GC. From the emerging predictive biomarkers for ICI that have been identified in the past decade (PD-L1 expression, tumor mutational burden, microsatellite instability, TILs, immune gene signatures, among others), microsatellite instability likely has the strongest value, but PD-L1 has demonstrated a meaningful association between level of expression in the tumor microenvironment and the magnitude of benefit derived from ICI in GC. Despite the limitations in the interpretation of PD-L1 expression (spatial and temporal heterogeneity, interobserver variability, IHC assay, and influence by chemotherapy or radiation therapy), the randomized clinical trials have been consistent to suggest that the subgroup of GC patients that do not express PD-L1 in the tumor microenvironment derive little, if any, benefit from ICI. Novel immunotherapeutic approaches and targeted therapies, as well as novel predictive biomarkers, are urgently needed to be incorporated in the management of GC, in order to bring hope to the patients and their families affected by this challenging disease.
Acknowledgments
Funding: None.
Footnote
Reporting Checklist: The authors have completed the Narrative Review reporting checklist. Available at https://jgo.amegroups.com/article/view/10.21037/jgo-22-1133/rc
Peer Review File: Available at https://jgo.amegroups.com/article/view/10.21037/jgo-22-1133/prf
Conflicts of Interest: All authors have completed the ICMJE uniform disclosure form (available at https://jgo.amegroups.com/article/view/10.21037/jgo-22-1133/coif). RDP reports honoraria for lectures from Servier, BMS, MSD and Lilly. She also reports consulting fees from BMS and Lilly as well as support for attending meetings from Astrazeneca and Daiichi and Advisory Boards from AztraZeneca, Daiichi, BMS, Lilly and Servier. MCMM reports honoraria for lectures from Lilly and support for attending meetins from Pfizer. AJ reports consulting fees from Bayer, Lilly, Servier, BMS and Merck. He also reports honoraria for lectures from BMS and Astellas. MG reports honoraria for lectures from Servier, BMS, MSD, Amgen and Lilly. She also reports support for attending meetings from Pfizer, AstraZeneca and Daiichi. The author receives consulting fees from Servier and BMS and also has participated on advisory boards from AstraZeneca, Daiichi, BMS, Lilly, Servier. JF reports honoraria for lectures from Bayer and Servier, support for attending meetings from Bayer and Servier and participation in Advisory Boards for Servier. BS reports honoraria for lectures from Janssen and Servier. TP has received support for attending meetings and travel from IPSEN. ACA reports honoraria for lectures from MSD, AstraZeneca, IPSEN, Servier, Norvartis and Daiichi. PHD reports honoraria for lectures from AstraZeneca and Sanofi. AP reports honoraria for lectures from Servier, MSD, Zodiac, Amgen and AstraZeneca. FL reports that honoraria for lectures from Servier, BMS, MSD, Lilly, and AstraZeneca. She also reports support for attending meeting from AstraZeneca, BMS and Lilly and participation in advisory boards with Servier. MS reports honoraria for lectures from Servier and has received support for attending meetings and/or travel from IPSEN. The other authors have no conflicts of interest to declare.
Ethical Statement: The authors are accountable for all aspects of the work in ensuring that questions related to the accuracy or integrity of any part of the work are appropriately investigated and resolved.
Open Access Statement: This is an Open Access article distributed in accordance with the Creative Commons Attribution-NonCommercial-NoDerivs 4.0 International License (CC BY-NC-ND 4.0), which permits the non-commercial replication and distribution of the article with the strict proviso that no changes or edits are made and the original work is properly cited (including links to both the formal publication through the relevant DOI and the license). See: https://creativecommons.org/licenses/by-nc-nd/4.0/.
References
- Sung H, Ferlay J, Siegel RL, et al. Global Cancer Statistics 2020: GLOBOCAN Estimates of Incidence and Mortality Worldwide for 36 Cancers in 185 Countries. CA Cancer J Clin 2021;71:209-49. [Crossref] [PubMed]
- Fashoyin-Aje L, Donoghue M, Chen H, et al. FDA Approval Summary: Pembrolizumab for Recurrent Locally Advanced or Metastatic Gastric or Gastroesophageal Junction Adenocarcinoma Expressing PD-L1. Oncologist 2019;24:103-9. [Crossref] [PubMed]
- EMA. Opdivo [Internet]. European Medicines Agency. 2018 [cited 2023 Jan 17]. Available online: https://www.ema.europa.eu/en/medicines/human/EPAR/opdivo
- Liu X, Hogg GD, DeNardo DG. Rethinking immune checkpoint blockade: “Beyond the T cell.” J Immunother Cancer 2021;9:e001460. [Crossref] [PubMed]
- Waldman AD, Fritz JM, Lenardo MJ. A guide to cancer immunotherapy: from T cell basic science to clinical practice. Nat Rev Immunol 2020;20:651-68. [Crossref] [PubMed]
- Shitara K, Özgüroğlu M, Bang YJ, et al. Pembrolizumab versus paclitaxel for previously treated, advanced gastric or gastro-oesophageal junction cancer (KEYNOTE-061): a randomised, open-label, controlled, phase 3 trial. Lancet 2018;392:123-33. [Crossref] [PubMed]
- Fuchs CS, Doi T, Jang RW, et al. Safety and Efficacy of Pembrolizumab Monotherapy in Patients With Previously Treated Advanced Gastric and Gastroesophageal Junction Cancer: Phase 2 Clinical KEYNOTE-059 Trial. JAMA Oncol 2018;4:e180013. [Crossref] [PubMed]
- Janjigian YY, Shitara K, Moehler M, et al. First-line nivolumab plus chemotherapy versus chemotherapy alone for advanced gastric, gastro-oesophageal junction, and oesophageal adenocarcinoma (CheckMate 649): a randomised, open-label, phase 3 trial. Lancet 2021;398:27-40. [Crossref] [PubMed]
- Park Y, Koh J, Na HY, et al. PD-L1 Testing in Gastric Cancer by the Combined Positive Score of the 22C3 PharmDx and SP263 Assay with Clinically Relevant Cut-offs. Cancer Res Treat 2020;52:661-70. [Crossref] [PubMed]
- Sharpe AH, Pauken KE. The diverse functions of the PD1 inhibitory pathway. Nat Rev Immunol 2018;18:153-67. [Crossref] [PubMed]
- Ohaegbulam KC, Assal A, Lazar-Molnar E, et al. Human cancer immunotherapy with antibodies to the PD-1 and PD-L1 pathway. Trends Mol Med 2015;21:24-33. [Crossref] [PubMed]
- Parry RV, Chemnitz JM, Frauwirth KA, et al. CTLA-4 and PD-1 receptors inhibit T-cell activation by distinct mechanisms. Mol Cell Biol 2005;25:9543-53. [Crossref] [PubMed]
- Disis ML. Mechanism of action of immunotherapy. Semin Oncol 2014;41:S3-13. [Crossref] [PubMed]
- Ribas A. Tumor Immunotherapy Directed at PD-1 [Internet].
10.1056/NEJMe1205943 . Massachusetts Medical Society; 2012 [cited 2022 Apr 19]. Available online: https://www.nejm.org/doi/pdf/10.1056/NEJMe120594310.1056/NEJMe1205943 - Kalluri R. The biology and function of fibroblasts in cancer. Nat Rev Cancer 2016;16:582-98. [Crossref] [PubMed]
- Elyada E, Bolisetty M, Laise P, et al. Cross-Species Single-Cell Analysis of Pancreatic Ductal Adenocarcinoma Reveals Antigen-Presenting Cancer-Associated Fibroblasts. Cancer Discov 2019;9:1102-23. [Crossref] [PubMed]
- Kobayashi H, Enomoto A, Woods SL, et al. Cancer-associated fibroblasts in gastrointestinal cancer. Nat Rev Gastroenterol Hepatol 2019;16:282-95. [Crossref] [PubMed]
- Sigal M, Reinés MDM, Müllerke S, et al. R-spondin-3 induces secretory, antimicrobial Lgr5(+) cells in the stomach. Nat Cell Biol 2019;21:812-23. [Crossref] [PubMed]
- Sigal M, Logan CY, Kapalczynska M, et al. Stromal R-spondin orchestrates gastric epithelial stem cells and gland homeostasis. Nature 2017;548:451-5. [Crossref] [PubMed]
- Berdiel-Acer M, Sanz-Pamplona R, Calon A, et al. Differences between CAFs and their paired NCF from adjacent colonic mucosa reveal functional heterogeneity of CAFs, providing prognostic information. Mol Oncol 2014;8:1290-305. [Crossref] [PubMed]
- Costea DE, Hills A, Osman AH, et al. Identification of two distinct carcinoma-associated fibroblast subtypes with differential tumor-promoting abilities in oral squamous cell carcinoma. Cancer Res 2013;73:3888-901. [Crossref] [PubMed]
- Mu L, Yu W, Su H, et al. Relationship between the expressions of PD-L1 and tumour-associated fibroblasts in gastric cancer. Artif Cells Nanomed Biotechnol 2019;47:1036-42. [Crossref] [PubMed]
- Butler JM, Kobayashi H, Rafii S. Instructive role of the vascular niche in promoting tumour growth and tissue repair by angiocrine factors. Nat Rev Cancer 2010;10:138-46. [Crossref] [PubMed]
- Wang Z, Wang Z, Li G, et al. CXCL1 from tumor-associated lymphatic endothelial cells drives gastric cancer cell into lymphatic system via activating integrin β1/FAK/AKT signaling. Cancer Lett 2017;385:28-38. [Crossref] [PubMed]
- Yang P, Hu Y, Zhou Q. The CXCL12-CXCR4 Signaling Axis Plays a Key Role in Cancer Metastasis and is a Potential Target for Developing Novel Therapeutics against Metastatic Cancer. Curr Med Chem 2020;27:5543-61. [Crossref] [PubMed]
- Oya Y, Hayakawa Y, Koike K. Tumor microenvironment in gastric cancers. Cancer Sci 2020;111:2696-707. [Crossref] [PubMed]
- Javle M, Smyth EC, Chau I. Ramucirumab: successfully targeting angiogenesis in gastric cancer. Clin Cancer Res 2014;20:5875-81. [Crossref] [PubMed]
- Biswas SK, Mantovani A. Macrophage plasticity and interaction with lymphocyte subsets: cancer as a paradigm. Nat Immunol 2010;11:889-96. [Crossref] [PubMed]
- Sade-Feldman M, Kanterman J, Ish-Shalom E, et al. Tumor necrosis factor-α blocks differentiation and enhances suppressive activity of immature myeloid cells during chronic inflammation. Immunity 2013;38:541-54. [Crossref] [PubMed]
- Tu S, Bhagat G, Cui G, et al. Overexpression of interleukin-1beta induces gastric inflammation and cancer and mobilizes myeloid-derived suppressor cells in mice. Cancer Cell 2008;14:408-19. [Crossref] [PubMed]
- Lee K, Hwang H, Nam KT. Immune response and the tumor microenvironment: how they communicate to regulate gastric cancer. Gut Liver 2014;8:131-9. [Crossref] [PubMed]
- Zhang F, Parayath NN, Ene CI, et al. Genetic programming of macrophages to perform anti-tumor functions using targeted mRNA nanocarriers. Nat Commun 2019;10:3974. [Crossref] [PubMed]
- Oishi S, Takano R, Tamura S, et al. M2 polarization of murine peritoneal macrophages induces regulatory cytokine production and suppresses T-cell proliferation. Immunology 2016;149:320-8. [Crossref] [PubMed]
- Veremeyko T, Yung AWY, Anthony DC, et al. Early Growth Response Gene-2 Is Essential for M1 and M2 Macrophage Activation and Plasticity by Modulation of the Transcription Factor CEBPβ. Front Immunol 2018;9:2515. [Crossref] [PubMed]
- Lin C, He H, Liu H, et al. Tumour-associated macrophages-derived CXCL8 determines immune evasion through autonomous PD-L1 expression in gastric cancer. Gut 2019;68:1764-73. [Crossref] [PubMed]
- Tang Y, Zhou C, Li Q, et al. Targeting depletion of myeloid-derived suppressor cells potentiates PD-L1 blockade efficacy in gastric and colon cancers. Oncoimmunology 2022;11:2131084. [Crossref] [PubMed]
- Stromnes IM, Brockenbrough JS, Izeradjene K, et al. Targeted depletion of an MDSC subset unmasks pancreatic ductal adenocarcinoma to adaptive immunity. Gut 2014;63:1769-81. [Crossref] [PubMed]
- Katoh H, Wang D, Daikoku T, et al. CXCR2-expressing myeloid-derived suppressor cells are essential to promote colitis-associated tumorigenesis. Cancer Cell 2013;24:631-44. [Crossref] [PubMed]
- Seif F, Khoshmirsafa M, Aazami H, et al. The role of JAK-STAT signaling pathway and its regulators in the fate of T helper cells. Cell Commun Signal 2017;15:23. [Crossref] [PubMed]
- O'Reilly LA, Putoczki TL, Mielke LA, et al. Loss of NF-κB1 Causes Gastric Cancer with Aberrant Inflammation and Expression of Immune Checkpoint Regulators in a STAT-1-Dependent Manner. Immunity 2018;48:570-583.e8. [Crossref] [PubMed]
- Ju X, Shen R, Huang P, et al. Predictive relevance of PD-L1 expression with pre-existing TILs in gastric cancer. Oncotarget 2017;8:99372-81. [Crossref] [PubMed]
- Amedei A, Della Bella C, Silvestri E, et al. T cells in gastric cancer: friends or foes. Clin Dev Immunol 2012;2012:690571. [Crossref] [PubMed]
- Lantuejoul S, Sound-Tsao M, Cooper WA, et al. PD-L1 Testing for Lung Cancer in 2019: Perspective From the IASLC Pathology Committee. J Thorac Oncol 2020;15:499-519. [Crossref] [PubMed]
- Burtness B, Harrington KJ, Greil R, et al. Pembrolizumab alone or with chemotherapy versus cetuximab with chemotherapy for recurrent or metastatic squamous cell carcinoma of the head and neck (KEYNOTE-048): a randomised, open-label, phase 3 study. Lancet 2019;394:1915-28. [Crossref] [PubMed]
- Sundar R, Smyth EC, Peng S, et al. Predictive Biomarkers of Immune Checkpoint Inhibition in Gastroesophageal Cancers. Front Oncol 2020;10:763. [Crossref] [PubMed]
- de Ruiter EJ, Mulder FJ, Koomen BM, et al. Comparison of three PD-L1 immunohistochemical assays in head and neck squamous cell carcinoma (HNSCC). Mod Pathol 2021;34:1125-32. [Crossref] [PubMed]
- Necchi A, Raggi D, Gallina A, et al. Updated Results of PURE-01 with Preliminary Activity of Neoadjuvant Pembrolizumab in Patients with Muscle-invasive Bladder Carcinoma with Variant Histologies. Eur Urol 2020;77:439-46. [Crossref] [PubMed]
- Yamashita K, Iwatsuki M, Harada K, et al. Prognostic impacts of the combined positive score and the tumor proportion score for programmed death ligand-1 expression by double immunohistochemical staining in patients with advanced gastric cancer. Gastric Cancer 2020;23:95-104. [Crossref] [PubMed]
- Kulangara K, Zhang N, Corigliano E, et al. Clinical Utility of the Combined Positive Score for Programmed Death Ligand-1 Expression and the Approval of Pembrolizumab for Treatment of Gastric Cancer. Arch Pathol Lab Med 2019;143:330-7. [Crossref] [PubMed]
- Shitara K, Van Cutsem E, Bang YJ, et al. Efficacy and Safety of Pembrolizumab or Pembrolizumab Plus Chemotherapy vs Chemotherapy Alone for Patients With First-line, Advanced Gastric Cancer: The KEYNOTE-062 Phase 3 Randomized Clinical Trial. JAMA Oncol 2020;6:1571-80. [Crossref] [PubMed]
- Narita Y, Sasaki E, Masuishi T, et al. PD-L1 immunohistochemistry comparison of 22C3 and 28-8 assays for gastric cancer. J Gastrointest Oncol 2021;12:2696-705. [Crossref] [PubMed]
- Ahn S, Kim KM. PD-L1 expression in gastric cancer: interchangeability of 22C3 and 28-8 pharmDx assays for responses to immunotherapy. Mod Pathol 2021;34:1719-27. [Crossref] [PubMed]
- Tay RYK, Yeong JPS, Lum J, et al. Choice of PD-L1 immunohistochemistry assay influences clinical eligibility for gastric cancer immunotherapy. JCO 2022;40:4026. [Crossref]
- Yeong J, Lum HYJ, Teo CB, et al. Choice of PD-L1 immunohistochemistry assay influences clinical eligibility for gastric cancer immunotherapy. Gastric Cancer 2022;25:741-50. [Crossref] [PubMed]
- Krohn D. PD-L1 IHC 22C3 pharmDx, Interpretation Manual, NSCLC 1% 50%.:76.
- Son SM, Woo CG, Kim DH, et al. Distinct tumor immune microenvironments in primary and metastatic lesions in gastric cancer patients. Sci Rep 2020;10:14293. [Crossref] [PubMed]
- Zhou J, Gong Z, Jia Q, et al. Programmed death ligand 1 expression and CD8(+) tumor-infiltrating lymphocyte density differences between paired primary and brain metastatic lesions in non-small cell lung cancer. Biochem Biophys Res Commun 2018;498:751-7. [Crossref] [PubMed]
- Haffner MC, Guner G, Taheri D, et al. Comprehensive Evaluation of Programmed Death-Ligand 1 Expression in Primary and Metastatic Prostate Cancer. Am J Pathol 2018;188:1478-85. [Crossref] [PubMed]
- Tawfik O, Kimler BF, Karnik T, et al. Clinicopathological correlation of PD-L1 expression in primary and metastatic breast cancer and infiltrating immune cells. Hum Pathol 2018;80:170-8. [Crossref] [PubMed]
- Eckstein M, Sikic D, Strissel PL, et al. Evolution of PD-1 and PD-L1 Gene and Protein Expression in Primary Tumors and Corresponding Liver Metastases of Metastatic Bladder Cancer. Eur Urol 2018;74:527-9. [Crossref] [PubMed]
- Colarossi L, Aiello E, Cumberbatch M, et al. Abstract 1536: Tumor heterogeneity and primary versus metastatic evaluation of PD-L1. Cancer Res 2019;79:1536. [Crossref]
- Zhou KI, Peterson B, Serritella A, et al. Spatial and Temporal Heterogeneity of PD-L1 Expression and Tumor Mutational Burden in Gastroesophageal Adenocarcinoma at Baseline Diagnosis and after Chemotherapy. Clin Cancer Res 2020;26:6453-63. [Crossref] [PubMed]
- Kim R, An M, Lee H, et al. Early Tumor-Immune Microenvironmental Remodeling and Response to First-Line Fluoropyrimidine and Platinum Chemotherapy in Advanced Gastric Cancer. Cancer Discov 2022;12:984-1001. [Crossref] [PubMed]
- Ye M, Huang D, Zhang Q, et al. Heterogeneous programmed death-ligand 1 expression in gastric cancer: comparison of tissue microarrays and whole sections. Cancer Cell Int 2020;20:186. [Crossref] [PubMed]
- Schoemig-Markiefka B, Eschbach J, Scheel AH, et al. Optimized PD-L1 scoring of gastric cancer. Gastric Cancer 2021;24:1115-22. [Crossref] [PubMed]
- Svensson MC, Borg D, Zhang C, et al. Expression of PD-L1 and PD-1 in Chemoradiotherapy-Naïve Esophageal and Gastric Adenocarcinoma: Relationship With Mismatch Repair Status and Survival. Front Oncol 2019;9:136. [Crossref] [PubMed]
- Erdogdu IH. MHC Class 1 and PDL-1 Status of Primary Tumor and Lymph Node Metastatic Tumor Tissue in Gastric Cancers. Gastroenterol Res Pract 2019;2019:4785098. [Crossref] [PubMed]
- Kim ST, Cristescu R, Bass AJ, et al. Comprehensive molecular characterization of clinical responses to PD-1 inhibition in metastatic gastric cancer. Nat Med 2018;24:1449-58. [Crossref] [PubMed]
- Ma C, Patel K, Singhi AD, et al. Programmed Death-Ligand 1 Expression Is Common in Gastric Cancer Associated With Epstein-Barr Virus or Microsatellite Instability. Am J Surg Pathol 2016;40:1496-506. [Crossref] [PubMed]
- Derks S, Liao X, Chiaravalli AM, et al. Abundant PD-L1 expression in Epstein-Barr Virus-infected gastric cancers. Oncotarget 2016;7:32925-32. [Crossref] [PubMed]
- Gu L, Chen M, Guo D, et al. PD-L1 and gastric cancer prognosis: A systematic review and meta-analysis. PLoS One 2017;12:e0182692. [Crossref] [PubMed]
- Nakano H, Saito M, Nakajima S, et al. PD-L1 overexpression in EBV-positive gastric cancer is caused by unique genomic or epigenomic mechanisms. Sci Rep 2021;11:1982. [Crossref] [PubMed]
- Roudko V, Bozkus CC, Orfanelli T, et al. Shared Immunogenic Poly-Epitope Frameshift Mutations in Microsatellite Unstable Tumors. Cell 2020;183:1634-1649.e17. [Crossref] [PubMed]
- Zhao Z, Li W, Zhang X, Ge M, Song C. Correlation between TMB and MSI in patients with solid tumors. JCO 2020;38:e15169. [Crossref]
- Pietrantonio F, Randon G, Di Bartolomeo M, et al. Predictive role of microsatellite instability for PD-1 blockade in patients with advanced gastric cancer: a meta-analysis of randomized clinical trials. ESMO Open 2021;6:100036. [Crossref] [PubMed]
- Kwon M, An M, Klempner SJ, et al. Determinants of Response and Intrinsic Resistance to PD-1 Blockade in Microsatellite Instability-High Gastric Cancer. Cancer Discov 2021;11:2168-85. [Crossref] [PubMed]
- Goodman AM, Kato S, Bazhenova L, et al. Tumor Mutational Burden as an Independent Predictor of Response to Immunotherapy in Diverse Cancers. Mol Cancer Ther 2017;16:2598-608. [Crossref] [PubMed]
- Legrand FA, Gandara DR, Mariathasan S, et al. Association of high tissue TMB and atezolizumab efficacy across multiple tumor types. JCO 2018;36:12000. [Crossref]
- Prasad V, Addeo A. The FDA approval of pembrolizumab for patients with TMB >10 mut/Mb: was it a wise decision? No. Ann Oncol 2020;31:1112-4. [Crossref] [PubMed]
- Strickler JH, Hanks BA, Khasraw M. Tumor Mutational Burden as a Predictor of Immunotherapy Response: Is More Always Better? Clin Cancer Res 2021;27:1236-41. [Crossref] [PubMed]
- Kim J, Kim B, Kang SY, et al. Tumor Mutational Burden Determined by Panel Sequencing Predicts Survival After Immunotherapy in Patients With Advanced Gastric Cancer. Front Oncol 2020;10:314. [Crossref] [PubMed]
- Shitara K, Özgüroğlu M, Bang YJ, et al. Molecular determinants of clinical outcomes with pembrolizumab versus paclitaxel in a randomized, open-label, phase III trial in patients with gastroesophageal adenocarcinoma. Ann Oncol 2021;32:1127-36. [Crossref] [PubMed]
- Folprecht G. Tumor mutational burden as a new biomarker for PD-1 antibody treatment in gastric cancer. Cancer Commun (Lond) 2019;39:74. [Crossref] [PubMed]
- Mishima S, Kawazoe A, Nakamura Y, et al. Clinicopathological and molecular features of responders to nivolumab for patients with advanced gastric cancer. J Immunother Cancer 2019;7:24. [Crossref] [PubMed]
- Hatakeyama K, Nagashima T, Urakami K, et al. Tumor mutational burden analysis of 2,000 Japanese cancer genomes using whole exome and targeted gene panel sequencing. Biomed Res 2018;39:159-67. [Crossref] [PubMed]
- Chalmers ZR, Connelly CF, Fabrizio D, et al. Analysis of 100,000 human cancer genomes reveals the landscape of tumor mutational burden. Genome Med 2017;9:34. [Crossref] [PubMed]
- Valero C, Lee M, Hoen D, et al. Response Rates to Anti-PD-1 Immunotherapy in Microsatellite-Stable Solid Tumors With 10 or More Mutations per Megabase. JAMA Oncol 2021;7:739-43. [Crossref] [PubMed]
- Huang RSP, Haberberger J, Severson E, et al. A pan-cancer analysis of PD-L1 immunohistochemistry and gene amplification, tumor mutation burden and microsatellite instability in 48,782 cases. Mod Pathol 2021;34:252-63. [Crossref] [PubMed]
- Chen Y, Wang Y, Luo H, et al. The frequency and inter-relationship of PD-L1 expression and tumour mutational burden across multiple types of advanced solid tumours in China. Exp Hematol Oncol 2020;9:17. [Crossref] [PubMed]
- Yarchoan M, Albacker LA, Hopkins AC, et al. PD-L1 expression and tumor mutational burden are independent biomarkers in most cancers. JCI Insight 2019;4:e126908. [Crossref] [PubMed]
- Kang YK, Chen LT, Ryu MH, et al. Nivolumab plus chemotherapy versus placebo plus chemotherapy in patients with HER2-negative, untreated, unresectable advanced or recurrent gastric or gastro-oesophageal junction cancer (ATTRACTION-4): a randomised, multicentre, double-blind, placebo-controlled, phase 3 trial. Lancet Oncol 2022;23:234-47. [Crossref] [PubMed]
- Janjigian YY, Bendell J, Calvo E, et al. CheckMate-032 Study: Efficacy and Safety of Nivolumab and Nivolumab Plus Ipilimumab in Patients With Metastatic Esophagogastric Cancer. J Clin Oncol 2018;36:2836-44. [Crossref] [PubMed]
- Bang YJ, Ruiz EY, Van Cutsem E, et al. Phase III, randomised trial of avelumab versus physician’s choice of chemotherapy as third-line treatment of patients with advanced gastric or gastro-oesophageal junction cancer: primary analysis of JAVELIN Gastric 300. Ann Oncol 2018;29:2052-60. [Crossref] [PubMed]
- Fuchs CS, Özgüroğlu M, Bang YJ, et al. Pembrolizumab versus paclitaxel for previously treated PD-L1-positive advanced gastric or gastroesophageal junction cancer: 2-year update of the randomized phase 3 KEYNOTE-061 trial. Gastric Cancer 2022;25:197-206. [Crossref] [PubMed]
- Bang YJ, Kang YK, Catenacci DV, et al. Pembrolizumab alone or in combination with chemotherapy as first-line therapy for patients with advanced gastric or gastroesophageal junction adenocarcinoma: results from the phase II nonrandomized KEYNOTE-059 study. Gastric Cancer 2019;22:828-37. [Crossref] [PubMed]
- Moehler M, Dvorkin M, Boku N, et al. Phase III Trial of Avelumab Maintenance After First-Line Induction Chemotherapy Versus Continuation of Chemotherapy in Patients With Gastric Cancers: Results From JAVELIN Gastric 100. J Clin Oncol 2021;39:966-77. [Crossref] [PubMed]
- Moehler M, Shitara K, Garrido M, et al. LBA6_PR Nivolumab (nivo) plus chemotherapy (chemo) versus chemo as first-line (1L) treatment for advanced gastric cancer/gastroesophageal junction cancer (GC/GEJC)/esophageal adenocarcinoma (EAC): First results of the CheckMate 649 study. Ann Oncol 2020;31:S1191. [Crossref]
- Larkin J, Chiarion-Sileni V, Gonzalez R, et al. Combined Nivolumab and Ipilimumab or Monotherapy in Untreated Melanoma. N Engl J Med 2015;373:23-34. [Crossref] [PubMed]
- Overman MJ, Lonardi S, Wong KYM, et al. Durable Clinical Benefit With Nivolumab Plus Ipilimumab in DNA Mismatch Repair-Deficient/Microsatellite Instability-High Metastatic Colorectal Cancer. J Clin Oncol 2018;36:773-9. [Crossref] [PubMed]
- Muro K, Chung HC, Shankaran V, et al. Pembrolizumab for patients with PD-L1-positive advanced gastric cancer (KEYNOTE-012): a multicentre, open-label, phase 1b trial. Lancet Oncol 2016;17:717-26. [Crossref] [PubMed]
- Commissioner O of the. FDA Approves First Immunotherapy for Initial Treatment of Gastric Cancer [Internet]. FDA. FDA; 2021 [cited 2022 Apr 14]. Available online: https://www.fda.gov/news-events/press-announcements/fda-approves-first-immunotherapy-initial-treatment-gastric-cancer