Long-term outcome analysis of Y90 radioembolization in hepatocellular carcinoma
Highlight box
Key findings
• In real-world experience, patients treated with Y90 had shorter PFS than those in clinical trials.
What is known and what is new?
• Careful patient selection is required for Y90 treatment with advanced-staged HCC with or without vascular invasion who have limited extrahepatic disease and preserved liver function.
• Our study emphasizes the importance of analyzing outcomes from RCTs in complementary fashion with real-world studies. Efficacy in RCTs vs. effectiveness in real-world practice may have significant differences due to differences in practice (e.g., use of RECIST vs. LiRADS).
What is the implication, and what should change now?
• Real-world studies play an essential role for comparison of OS and PFS with next-generation HCC therapeutic trials. Results from a RCT may not be applicable in real-world clinical practice given the strict eligibility criteria and controlled setting required in a RCT. Therefore, real-world outcomes studies such as ours emphasize the importance of not only analyzing outcomes from RCTs alone but in complimentary fashion with real-world studies as there may be significant differences.
Introduction
Hepatocellular carcinoma (HCC) is primary liver cancer and the third leading cause of cancer-related death globally, reaching over 900,000 new cases in 2020 (1,2) with significant mortality rates in the United States (US) (3-5). Since 1980, the incidence of liver cancer in the US had more than tripled with risk factors for developing HCC including hepatitis B virus (HBV), hepatitis C virus (HCV), alcohol-associated liver disease (ALD) and nonalcoholic fatty liver disease (NAFLD) (5). Worldwide, the highest incidence of HCC is found in areas endemic for chronic HBV (2,6) while the rising burden of liver cancer now in the US is predominantly caused by ALD and NAFLD (7,8).
Yttrium-90 (Y90) radioembolization, a form of locoregional therapy (LRT), has been one treatment modality of particular interest in HCC (9,10). It involves the passage of a catheter through the hepatic artery, localized to the area of the tumor, where Y90 microspheres are released. These microspheres embed themselves into the vascular bed of the tumor and release localized radiation over several weeks. The ultimate goal is to provide localized radiation to the tumor (11). The role of Y90 in HCC management has changed over the past decade as it was initially used only in advanced, non-curable HCC, or with portal vein thrombosis (PVT). It is now applied in both early to advanced HCC, including treatment as a bridge to transplant.
Y90 has been shown to be efficacious in the treatment of primary HCC (12-20) in multiple studies, and its safety profile appears to be acceptable in the majority of patients with primary HCC and intact hepatic function. However, transient toxicities such as abdominal pain, fatigue, nausea/vomiting, weight loss, diarrhea, and low-grade fever are common (21). Other observed liver-related toxicities, including hyperbilirubinemia (22), liver decompensation (e.g., ascites/fluid retention, encephalopathy) and radiation embolization induced liver disease (REILD), may contribute to severe acute toxicity (23) or contribute to chronic hepatic functional decline over time. This is especially relevant in the HCC population, where a majority of patients have pre-existing cirrhosis.
Previous studies have generally followed patients for short periods of time (range, 7.8–32 months) and late effects from treatment and effects on liver function are not well characterized (17,18). Further study is needed to understand the long-term outcomes post-Y90. In this retrospective study, we sought to further evaluate the long-term outcomes of Y90 treatment in real-world practice in patients with advanced HCC. We evaluated the median overall survival (OS), progression-free survival (PFS), and overall response rate (ORR) using longitudinal radiology reports. We also examined both acute and late Y90-induced hepatotoxicity by measuring the model for end-stage liver disease (MELD) and Child-Pugh (CP) scores at intervals from initial time of Y90 treatment to either the time of death or lost to follow-up. Finally, we evaluated any other complications that occurred secondary to Y90 to further assess treatment safety. We present this article in accordance with the STROBE reporting checklist (available at https://jgo.amegroups.com/article/view/10.21037/jgo-22-882/rc).
Methods
Patients
We retrospectively identified eligible patients with HCC who received Y90 at Virginia Commonwealth University (VCU) Health in Richmond, Virginia between 2008 and 2015. Patients were identified through a database maintained through the VCU Division of Vascular Interventional Radiology and further screened for eligibility if there was at least 6 months follow-up after initial treatment. Patients were included in the study if they were at least 18 years old and had a diagnosis of primary HCC that was treated with Y90 at VCU Health between 2008 and 2015. Patients included in the study must have had clinical follow up for at least 30 days and one additional follow-up visit for response evaluation with an imaging modality after initial treatment. The study was conducted in accordance with the Declaration of Helsinki (as revised in 2013). The study was approved by the Virginia Commonwealth University Institutional Review Board (No. HM20007405) and individual consent for this retrospective analysis was waived.
Imaging protocols
Diagnosis of HCC was made using standardized protocols based on imaging criteria following United Network for Organ Sharing guidelines or with biopsy. For the purpose of this study, diagnosis of staging utilized American Joint Committee on Cancer TNM Staging (5th or 7th editions) for liver tumors (AJCC TNM). MRI was performed with gadolinium in majority of patients with most examinations using a 1.5T Siemens Avanto MRI scanner. Some patients were scanned in a 1.5T GE Signa device. Four post-contrast phase imaging was obtained with arterial, portal venous, 3- and 5-minute delayed phases. Over the study period, gadolinium contrast agents used included ProHance® (gadoteridol), Magnevist® (gadopentetate dimeglumine), MultiHance® (gadobenate dimeglumine), and occasionally Eovist® (gadoxetate disodium). Between 2008 and 2014, unenhanced images with subsequent post-contrast images obtained in the arterial and portal venous phases were included as part of the CT protocol. An additional delayed venous phase was included in 2014 to meet American College of Radiology (ACR) Liver Reporting and Data system (Li-RADS) recommendations.
A RedCap database was used to enter patient information. The clinical information included a patient’s baseline characteristics, treatments received, and long-term outcomes. Baseline characteristics consisted of etiology of liver disease, fibrosis score, HCC stage (AJCC TNM) at diagnosis, and radiologic characteristics. Treatment information collected included any other prior LRT [transarterial chemoembolization (TACE), radiofrequency ablation, stereotactic body radiation therapy], and systemic therapy (sorafenib, chemotherapy, immunotherapy). All treatment information regarding Y90, including treatment type, dose, date of treatment, and any radiation exposure was collected. Baseline laboratory values prior to Y90 such as liver function tests, renal function, and coagulation tests as well as calculated CP and MELD-NA scores, were collected prior to Y90 therapy. Complications of Y90 treatments and adverse events such as radiation embolization-induced liver disease (REILD), radioembolization-induced chronic hepatotoxicity (RECHT), acute liver injury, liver decompensation, and infection were collected. Laboratory values, as well as any clinical markers of decompensation of liver disease such as ascites and encephalopathy at 30 and 90 days after treatment, were collected.
Endpoints and statistical analysis
Continuous data were summarized using means (std) or medians (min, max) or both while categorical data were summarized by reporting frequency (percent). To determine the efficacy of Y90 as a treatment for primary HCC, PFS and OS were analyzed using both univariate (Kaplan-Meier) and multivariable (Cox proportional hazards regression) to control for covariates that could potentially impact survival. For analysis, PFS time was defined as the duration of time (in months) from the start of Y90 treatment until the date of progression, or if progression had not occurred, the date of data extraction. If progression had not occurred, the patient was considered to be a censored observation for the purposes of PFS analysis. OS time was defined as the duration of time (in months) from either the date of diagnosis or the start of Y90 treatment until the date of death, or if death had not occurred, the date of data extraction. As was the case for PFS, if death had not occurred, the patient was considered to be a censored observation for the purposes of OS analysis. For OS and PFS, patients who had undergone transplant were censored at the date of transplant. SAS v9.4 was used for all analyses and unless otherwise specified and α=0.05 level of significance was utilized to determine statistical significance.
Results
Baseline clinical characteristics
Of the 134 patients enrolled, the mean age was 60 years old, 78% were male, and 59% were white, 32% were Black/African American/African and 9% other (Table 1). Underlying liver disease included HCV (66%), ALD (23%), NAFLD (19%), and HBV (5%). The majority of patients (85%) had CP A. While 39% patients were within Milan Criteria, overall, 34% were at AJCC TNM stage 1, 31% at stage 2 and 26% at stage 3. The median MELD-NA and CP scores at baseline was 10, and 6 respectively (Table 1).
Table 1
Characteristics | Value |
---|---|
Age (years) at first visit or diagnosis, median [range] | 60 [44–90] |
Gender (male), n [%] | 78 [105] |
Race/ethnicity, n [%] | |
White | 59 [79] |
Black/African-American/African | 32 [43] |
Hispanic/Latino | 2 [3] |
Asian | 2 [3] |
South Asian | 2 [3] |
Native Hawaiian/Pacific Islander | 2 [3] |
American Indian/Alaskan Native | 0 [0] |
Other | 0 [0] |
Primary liver disease, n [%] | |
HCV | 66 [89] |
HBV | 5 [4] |
HDV (coinfection with HBV) | 0 [0] |
NAFLD | 19 [25] |
Alcoholic liver disease | 23 [31] |
Hemochromatosis | 1 [1] |
Autoimmune hepatitis | 1 [1] |
Alpha-1 antitrypsin | 1 [1] |
Wilson’s | 1 [1] |
Primary biliary cirrhosis | 1 [1] |
Primary sclerosing cholangitis | 1 [1] |
Other | 0 [0] |
Cryptogenic | 1 [1] |
None | 4 [5] |
HCC staging 1–4 (AJCC TNM), n [%] | |
1 | 34 [45] |
2 | 31 [42] |
3 | 26 [35] |
4 | 9 [12] |
Tumor location, n [%] | |
Unilobar | 74 [99] |
Bilobar | 22 [29] |
Diffuse | 4 [5] |
Not reported | 1 [1] |
MELD-NA at baseline, median (range) | 10.0 (6.0–21.0) |
CP class at baseline, n [%] | |
A | 85 [114] |
B | 14 [19] |
C | 1 [1] |
Other HCC treatments received, n [%] | |
TACE | 43 [58] |
RFA | 19 [26] |
SBRT | 4 [5] |
Other treatments received (ever), n [%] | |
Sorafenib | 34 [45] |
Chemotherapy | 1 [2] |
Immunotherapy | 2 [3] |
Patients with PVT, n [%] | |
Main PVT | 7 [10] |
Branch PVT | 16 [21] |
Both main and branch PVT | 4 [6] |
PVT, n [%] | |
Bland thrombus | 3 [4] |
Malignant thrombus | 15 [20] |
Unclear/unable to determine | 1 [2] |
Not reported | 8 [11] |
Location of PVT, n [%] | |
Right branch | 12 [16] |
Left branch | 7 [10] |
Main | 7 [10] |
Patients who underwent liver transplantation after Y90, n [%] | 6 [8] |
Y90 treatment, n [%] | |
Single lobe | 89 [119] |
Bi-lobar | 11 [15] |
HCV, hepatitis C virus; HBV, hepatitis B virus; HDV, hepatitis D virus; NAFLD, non-alcoholic fatty liver disease; HCC, hepatocelllular carcinoma; AJCC, American Joint Committee of Cancer; MELD-NA, model for end-stage liver disease-sodium; CP, Child-Pugh; TACE, transarterial chemoembolization; RFA, radiofrequency ablation; SBRT, stereotactic body radiation therapy; PVT, portal vein thrombosis.
OS and PFS
Median OS for the study population from date of diagnosis was 28 months [95% confidence interval (CI): 22.21–38.05]. OS and PFS from date of Y90 treatment was 15 and 3 months, respectively (Table 2). When OS was analyzed based on CP class, CP A patients (85%) had a median OS of 17 months (95% CI: 9.59–23.10) from date of Y90 treatment compared to a median of 8 months (95% CI: 4.60–15.64) for CP B. For PFS, CP A patients had a median PFS of 3 months (95% CI: 2.99–5.55) from date of Y90 treatment compared to a median of 4 months (95% CI: 2.07–8.28) for CP B patients. No significant differences were seen between cancer stage and OS (Table 3, Figure 1A). However, analysis between PFS and cancer stage did show significant difference between cancer stage 1 and 3 with longer median PFS seen in stage 1 (Table 3, Figure 1B).
Table 2
OS/PFS | Total (n) | Failed† (n) | Censored* (n) | Median survival time (months) (95% CI) |
---|---|---|---|---|
OS from date of diagnosis | 134 | 94 | 40 | 28.22 (22.21–38.05) |
OS from date of Y90 session | 134 | 94 | 40 | 14.52 (8.64–19.78) |
PFS from date of Y90 session | 131 | 99 | 32 | 3.45 (3.06–4.63) |
†, death or progression; *, OS censored: patient is still alive; PFS censored: no progression of cancer. OS, overall survival; PFS, progression-free survival.
Table 3
Cancer stage† | OS, months, median (95% CI) | PFS, months, median (95% CI) |
---|---|---|
I | 19.78 (7.59–28.29) | 6.60 (3.19–9.13) |
II | 15.64 (8.28–43.20) | 4.30 (2.76–8.31) |
III | 8.35 (5.32–14.29) | 2.60 (2.14–3.06) |
IV | 12.50 (5.65, –) | 9.00 (2.46–9.89) |
†, I, stage 1A/T1a, stage 1B/T1b; II, stage 2/T2; III, stage 3A/T3, stage 3B/T4; IV, stage 4A/any T, N1, M0, stage 4B/any T, any N, M1. OS, overall survival; PFS, progression-free survival; AJCC, American Joint Committee of Cancer.
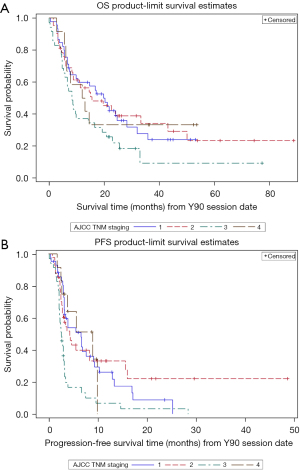
Patients with no PVT had a median OS of 17 months (95% CI: 11.37–24.12) from date of Y90 compared to a median of 7 months (95% CI: 4.50–9.89) for patients with presence of PVT. This difference was statistically significant by both the Log-Rank (χ2=7.68, P=0.0056) and the Wilcoxon (χ2=8.89, P=0.0029) tests (Figure 2). When examining PFS, patients with no PVT had a median PFS of 4 months [95% CI: 3.19–6.67; mean survival 8.75 (1.00) months] from date of Y90 treatment compared to a median of 3 months [95% CI: 2.14–3.29; mean survival 4.05 (0.74) months] for patients with a history of PVT. This difference was statistically significant by both the Log-Rank (χ2=8.33, P=0.0039) and the Wilcoxon (χ2=5.33, P=0.0210) tests.
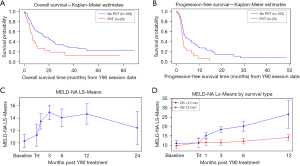
Both univariate and multivariate analysis for OS and PFS were performed controlling for various covariates (Table 5). Univariate analysis performed for MELD-NA score, CP score, and OS identified a trend that OS ≥12 months was associated with lower MELD-NA with a median MELD-NA of 10 while those patients with a median MELD-NA of 11 were likely to have OS <12 months (P=0.0937). OS ≥12 months was associated with lower CP score with a median 5.0 while those with a median 6.0 were likely to have OS <12 months (<0.0001) (Table 4). Median total bilirubin and INR of 1.0 and 1.2, respectively, were associated with OS <12 months, while median total bilirubin and INR of 0.80 and 1.10 were associated with OS ≥12 months (P=0.0037 and P=0.0500, respectively). When multivariate analysis was performed for age, MELD-NA score, AJCC TNM stage at diagnosis, and CP score, older age (HR, 1.03; 95% CI: 1.01–1.05; P=0.0076), higher MELD-NA (HR, 1.08; 95% CI: 1.00–1.15; P=0.0420), and higher CP (P=0.0022) scores were associated with significantly lower OS. PFS was significantly shorter in those patients with advanced stage disease at diagnosis (HR, 1.79; 95% CI: 1.19–2.69; P=0.0049) and higher CP score (HR, 1.27; 95% CI: 0.99–1.64; P=0.0564).
Table 5
MELD/CP/hepatic panel | PFS <6 months (n=92) | PFS ≥6 months (n=39) | P value | |||
---|---|---|---|---|---|---|
Mean (SD) | Median (range) | Mean (SD) | Median (range) | |||
MELD | 9.58 (3.19) | 9.0 (6.0–21.0) | 8.05 (1.87) | 7.0 (6.0–13.0) | 0.0152 | |
MELD-NA | 10.68 (3.59) | 10.0 (6.0–21.0) | 9.16 (2.53) | 9.0 (6.0–16.0) | 0.0344 | |
CP | 6.19 (1.34) | 6.0 (5.0–11.0) | 5.51 (0.90) | 5.0 (5.0–8.0) | 0.0053 | |
AST | 88.15 (53.91) | 72.5 (21.0–264.0) | 88.46 (75.16) | 72.0 (21.0–313.0) | 0.3790 | |
ALT | 72.40 (48.36) | 57.0 (10.0–251.0) | 79.16 (80.12) | 55.0 (20.0–442.0) | 0.9297 | |
Bilirubin | 1.08 (0.65) | 0.9 (0.2–3.7) | 0.92 (0.57) | 0.8 (0.3–2.6) | 0.1276 | |
INR | 1.21 (0.24) | 1.2 (0.9–2.4) | 1.11 (0.10) | 1.1 (1.0–1.4) | 0.0133 |
PFS, progression-free survival; MELD, model for end-stage liver disease; MELD-NA, model for end-stage liver disease-sodium; CP, Child-Pugh; AST, aspartate aminotransferase; ALT, alanine transaminase; INR, international normalized ratio.
Table 4
MELD/CP/hepatic panel | OS <12 months (n=64), median (range) | OS ≥12 months (n=70), median (range) | P value |
---|---|---|---|
MELD-NA | 10.5 (6.0–21.0) | 9.5 (6.0–19.0) | 0.0937 |
CP | 6.0 (5.0–11.0) | 5.0 (5.0–8.0) | <0.0001 |
AST | 80.0 (23.0–313.0) | 62.0 (21.0–298.0) | 0.0402 |
ALT | 63.0 (10.0–292.0) | 54.0 (20.0–442.0) | 0.5557 |
Bilirubin | 1.0 (0.2–3.7) | 0.8 (0.3–3.5) | 0.0037 |
INR | 1.2 (0.9–2.4) | 1.1 (1.0–2.4) | 0.0500 |
MELD-NA, model for end-stage liver disease-sodium; CP, Child-Pugh; AST, aspartate aminotransferase; ALT, alanine transaminase; INR, international normalized ratio.
MELD-NA and CP score after Y90
MELD-NA and CP scores increased after Y90, peaking at 3 months post-treatment. The scores were statistically significantly higher for MELD-NA at 1, 3, 6, and 12 months post-treatment than on Day 0 (P<0.0001), and for CP score at 3 months post-treatment (P=0.0010). The nadirs of MELD-NA and CP score were both noted at 24 months, although these were not statistically significant (Table 6 and Figure 2).
Table 6
Time | Estimate | SE | Difference from baseline | |||
---|---|---|---|---|---|---|
Estimate | SE | t value | Adjusted P value | |||
Baseline | 10.28 | 0.540 | – | – | – | – |
Treatment | 11.24 | 0.900 | 0.96 | 1.050 | 0.92 | 0.9067 |
30 days post-treatment | 13.46 | 0.629 | 3.18 | 0.829 | 3.84 | 0.0008 |
90 days post-treatment | 14.91 | 0.584 | 4.63 | 0.799 | 5.79 | <0.0001 |
180 days post-treatment | 14.03 | 0.726 | 3.75 | 0.905 | 4.14 | 0.0002 |
365 days post-treatment | 14.66 | 0.841 | 4.38 | 0.999 | 4.38 | <0.0001 |
730 days post-treatment | 12.39 | 1.359 | 2.11 | 1.462 | 1.44 | 0.5709 |
MELD-NA, model for end-stage liver disease-sodium; SE, standard error.
Analysis on secondary outcomes was performed comparing MELD-NA/CP scores between long-term survivors (OS >12 months) and short-term survivors (OS <12 months) at baseline and after Y90 treatment (Table 7). Baseline MELD-NA/CP scores for short-term survivors was higher at 11/6.5 compared to long-term survivors with 9.6/5.5. At 30- and 90-day post-treatment, a significantly higher increase in change in MELD-NA/CP scores was noted for short-term survivors compared to the long-term survivors. These differences between survivor groups were statistically significant (for MELD-NA, F5,433=6.44, P<0.001; and for CP score, F5,409=4.82, P=0.0003).
Table 7
Time | MELD-NA | Child-Pugh score | |||
---|---|---|---|---|---|
Short-term (n=67) | Long-term (n=67) | Short-term (n=67) | Long-term (n=67) | ||
Baseline | 11.02 (0.718) | 9.59 (0.693) | 6.45 (0.195) | 5.54 (0.188) | |
Treatment | 11.13 (1.110) | 11.39 (1.254) | 5.86 (0.308) | 5.47 (0.350) | |
30 days post-treatment | 15.13 (0.793) | 11.54 (0.852) | 6.83 (0.225) | 5.736 (0.237) | |
90 days post-treatment | 18.42 (0.768) | 11.396 (0.768) | 8.00 (0.217) | 5.76 (0.213) | |
180 days post-treatment | 20.13 (1.374) | 12.13 (0.768) | 8.00 (0.400) | 5.91 (0.213) | |
365 days post-treatment | 26.50 (3.762) | 14.13 (0.793) | 11.00 (1.442) | 6.20 (0.228) |
Data are shown as mean (standard error). For both MELD-NA and Child-Pugh score, the interaction term between Survival group and time was statistically significant (for MELD-NA, F5,433=6.44, P<0.001) and for Child-Pugh score, F5,409=4.82, P=0.0003) indicating that both survival group and time are statistically significant, but differences between groups (time) depends time (group). MELD-NA, model for end-stage liver disease-sodium.
Y90 toxicities
Overall, 51% patients reported some form of side effects. Nine patients (7%) experienced REILD, while more common side effects included fatigue (21%), abdominal pain (23%) and nausea/vomiting (14%) (Table 8). Other common grade 1 or 2 toxicities were elevated liver enzymes (4%) and total bilirubin levels (24–26%) (17-19,22,24,25). Lower baseline CP classes had less grade 3 or 4 toxicities, 5% for CP A and 11% for CP B (26). Other symptoms of liver decompensation reported included 9% ascites/fluid retention, 4% hepatic encephalopathy (Table 8). Only one death was noted.
Table 8
General side effects | N (%) |
---|---|
Fatigue | 28 (20.90) |
Abdominal pain | 31 (23.13) |
Nausea/vomiting | 19 (14.18) |
Anorexia | 9 (6.72) |
Diarrhea | 3 (2.24) |
Fever/chills | 1 (0.75) |
Infection | 1 (0.75) |
Sepsis | 1 (0.75) |
Renal failure | 0 (0.00) |
Weight loss | 1 (0.75) |
Abnormal liver test | 5 (3.73) |
Worsening liver failure | 3 (2.24) |
Intraperitoneal hemorrhage | 1 (0.75) |
Encephalopathy | 6 (4.48) |
Pleural effusion | 0 (0.00) |
Hypotension | 1 (0.75) |
Cancer seeding | 0 (0.00) |
Liver abscess | 0 (0.00) |
Liver infarction | 0 (0.00) |
Gastrointestinal bleed | 1 (0.75) |
Ascites/fluid retention | 12 (8.96) |
Hyponatremia | 1 (0.75) |
Death | 1 (0.75) |
Other | 11 (8.21) |
Cholangitis | 0 (0.00) |
Malaise | 0 (0.00) |
Skin irritation | 1 (0.75) |
Radiation embolization induced liver disease | 9 (6.72) |
Leukocytosis | 0 (0.00) |
Patients who reported general side effects | 69 (51.49) |
Discussion
Despite the common use of Y90 in the treatment of HCC, the long-term outcomes of patients with HCC treated with Y90 have not been fully studied. Our study sought to determine OS, PFS, and ORR based on patient characteristics at baseline (including CP class, MELD score, TNM staging, and the presence of PVT) and to assess the safety profile during treatment. In this retrospective, “real-world” study of 134 patients who received Y90, the OS from date of diagnosis was 28 months, OS from date of Y90 was 15 months, and PFS from date of Y90 was 3 months. CP A patients (79%) had an increased median OS of 17 months compared to 8 months in CP B patients. However, this was not statistically significant. For PFS, CP A had a median PFS of 3 months from date of Y90, while CP B had 4 months. Both association between OS and PFS and MELD scores and CP class were not statistically significant. Between OS and cancer stage, no differences were seen, while PFS and cancer stage showed significant differences between stage 1 and 3. The lack of statistical significance may be attributed to our small sample size. However, it should be noted that our multivariate analysis demonstrated correlation between older age, high cancer stage, higher MELD and CP class and presence of PVT with significantly lower OS. Those with advanced stage disease at diagnosis and higher CP score also had significantly shorter PFS.
Long-term outcomes
Multiple studies have found median OS times ranging from 17 to 18 months for CP A and 6 to 8 months for CP B, and PFS ranging from 8 to 18 months (17-19). One of the first outcome-focused studies led by Salem et al. examined response rate, time to progression (TTP), and OS across CP classes in 291 patients with HCC demonstrating objective response rates of 42% and 57% based on the World Health Organization and European Association for the Study of the Liver criteria, respectively (17). They also showed that TTP and OS varied with CP class. Median TTP was 7.9 months with median OS of 17.2 months in patients with CP class A compared to 7.7 months in patients with CP class B. Patients with CP B and PVT had the worst median OS of 5.6 months (17). A European investigation of Y90 in 108 patients with advanced HCC demonstrated a median TTP of 10 months and median OS of 16.4 months (18). Overall, these findings confirm data reported in other studies, which found significant correlation between CP score in relation to OS and PFS (17-19).
While our data for OS after Y90 treatment is consistent with these prior studies, we found a shorter PFS in our patient population. This may reflect the utilization of RECIST in clinical trials in contrast to clinical radiology practice, as was used in our cohort in determining disease progression. Standard clinical radiology practice for all liver transplant centers in the US utilize LiRADS for diagnosis and post treatment response of HCC instead of the RECIST criteria (27). The LiRADS algorithm was initially introduced by the ACR in 2011 to standardize the reporting of treated lesions and is applicable after any type of LRT. The Li-RADS treatment response (LR-TR) algorithm broadens the definition of viable tumor with the addition of novel imaging features including the appearance of washout and enhancement similar to pretreatment. In contrast, arterial phase hyperenhancement for the mRECIST criteria is considered the only characteristic of a viable tumor. In addition, under the LR-TR algorithm, there is an equivocal classification when there is difficulty distinguishing between viable tumor and expected post treatment enhancement in a treated lesion. The LR-TR algorithm uses a ternary system that categorizes the treated observations as: (I) LR-TR viable (probably or definitely viable), (II) equivocal (equivocally viable), or (III) nonviable category. By defining viable disease and providing non-categorizable, equivocal, and nonviable treatment response categories, this further expands on the mRECIST approach. Differing also from mRECIST, the Li-RADS treatment response categories are assigned on a lesion-by-lesion basis and are not assigned to the whole liver or patient.
Studies have compared the diagnostic performance between the LR-TR algorithm and mRECIST criteria with reported results varying considerably. One meta-analysis comparing mRECIST and LiRADS criteria have suggested that LiRADS has better specificity than mRECIST without significant difference in sensitivity for the diagnosis of viable HCC after LRT (28). Given the variable response criteria for HCC including RECIST, modified RECIST, and immune response RECIST, this underscores the utility of OS measurements in formal trials of HCC therapeutic interventions.
Our results also showed a significant effect of PVT on OS and PFS. Patients with no PVT had a median OS of 17 months from date of Y90 treatment compared to a median OS of 7 months for patients with PVT. When examining PFS, we found that patients with no PVT had a median PFS of 4 months from date of Y90 treatment compared to a median of 3 months for patients with PVT. While an estimated 1/3 patients with HCC will develop a PVT (29), Y90 can be safely used in the presence of PVT as an alternative to TACE given concerns for risk of acute liver failure caused by hepatic ischemia or infarction (17). A systematic review demonstrated that PVT has a significantly negative effect on prognosis, with the median OS reduced to 2–4 months in those patients who had unresectable HCC with PVT compared to 10–24 months in those without PVT (24). Our results are consistent with previous studies, suggesting more limited utility in this patient population.
Overall, our findings confirm data reported in other studies, which found significant correlation between CP score in relation to OS and PFS (17-19). Interestingly, this differed from the study by Mazzaferro et al., which found no significant difference in PFS in those patients with or without PVT (19). In one study, a significant correlation was found between OS and extension of PVT and tumor burden (30). Another study found that tumor burden and high bilirubin significantly affected OS in patients with PVT (31). Overall, a common theme found in these studies is that minimal functional liver capacity at time of diagnosis, as seen in patients with higher CP class and advanced tumor burden, significantly impacts OS and PFS. Decline in liver function is a contraindication for Y90 and precludes the ability to treat tumor burden safely as Y90 can cause worsening liver failure.
We also evaluated liver function tests and the MELD score after Y90 and its effect on OS and PFS (Table 5). We compared those who survived less than a year and those greater than a year, and found a significant difference in patient baseline CP class, bilirubin, and INR. Patients with lower CP class, bilirubin, and INR were more likely to have an OS greater than 6 months. Lower MELD-NA, CP class, and INR were more likely to be seen in those with PFS greater than 6 months. This again reinforces the importance of overall liver function at baseline, which is influenced by the status of the underlying liver disease (presence of cirrhosis or not, and well compensated vs. decompensated status) as well as the extent of tumor burden.
MELD-NA and CP class remain important markers to assess liver function during and after treatment. We followed MELD-NA and CP scores at baseline, 30 days, 3, 6, 12, and 24 months post-Y90. MELD scores were statistically significantly higher at 1, 3, 6, and 12 months post-treatment than on Day 0 versus an increase at 3 months for CP scores. Recovery was seen at 24 months reflected by a nadir in MELD and CP scores, although this was not statistically significant. The increasing MELD and CP scores over time likely reflects a combination of liver decompensation (not meeting REILD criteria but includes the development of worsening liver failure, encephalopathy, ascites/fluid retention) (Table 8), REILD, and progression of HCC, all which can worsen synthetic liver function. The observed recovery at 24 months is likely due to durable survivors who received reasonable tumor treatment effect from therapy and did not experience significant long-term complications from Y90.
In addition, our secondary outcomes analysis comparing differences in MELD-NA/CP between long-term survivors (OS >12 months) and short-term survivors (OS <12 months) at baseline and after 30- and 90-day post-Y90 treatment showed again a higher baseline MELD-NA/CP for short-term survivors than long-term survivors. However, a much higher increase in MELD-NA/CP at 30- and 90-day post-treatment was also observed in the short-term survivors compared to the long-term survivors. These differences between survivor groups over time post-treatment was statistically significant. The predictor of poorer outcomes could be guided by the degree of increase in MELD-NA/CP post-treatment when compared to baseline.
Long-term safety profile
The relative safety and efficacy of Y90 has been confirmed in a number of clinical trials. Current guidelines clearly advise against the use of Y90 in patients with clinical liver decompensation and significantly abnormal liver function tests, particularly with total bilirubin >2 mg/dL or albumin <3.0 g/dL to avoid the risk of REILD (32). Nine patients (7%) in our cohort experienced REILD, while others frequently had fatigue (21%), abdominal pain (23%) and nausea/vomiting (14%) (Table 7). These side effects are similar to those previously reported in prior studies. One death in our cohort occurred a day after Y90 treatment and was likely directly related to complications from the procedure. Other common grade 1 or 2 toxicities were elevated liver enzymes (2.5–34%) and bilirubin levels (24–26%) (17-19,24,26,27). Lower baseline CP scores had less grade 3 or 4 toxicities, 5% for CP A and 11% for CP B (26).
One small, single center, retrospective study of 106 patients evaluated a delayed hepatotoxicity from Y90 that was beyond the timeline of REILD based on the temporal relationship of adverse events to initial treatment. REILD occurs within 1 to 2 months after Y90 treatment and is characterized by jaundice and ascites in the absence of tumor progression or biliary obstruction and typically resolves within 6 months. A new classification system has been developed to capture the distinct clinical entity of chronic hepatotoxicity called RECHT, which occurs at least 6 months after initial therapy in the absence of tumor progression (33). Laboratory (INR, bilirubin, ALT, AST, alkaline phosphatase, albumin, platelets) and clinical toxicity (encephalopathy, ascites, varices, PVT) criteria were used for RECHT diagnosis. Risk factors for RECHT appeared to be associated with higher total tumor volume within the liver and possibly the cumulative dose of Y90. In this study, the median occurrence of RECHT was at 2 years (range of 6 months to 5 years) following the first Y90 treatment. It is unclear if any patients in our study had adverse events reflecting RECHT. Rising MELD scores in our study were significantly higher at 1, 3, 6, and 12 months post-treatment while only an increase at 3 months for CP scores. Decrease in MELD and CP scores were seen at 24 months likely due to our durable survivors receiving good treatment effect.
Real-world vs. clinical trial outcomes
Our study supports previous studies evaluating Y90 therapy, affirming the importance of carefully selecting patients. This would include those with advanced-staged HCC with or without vascular invasion and with limited extrahepatic disease but with preserved liver function. Our study also reinforces the potential discrepancies in outcomes that can be seen in a clinical trial setting versus the real-world setting of clinical practice. Results from a randomized controlled trials (RCTs) may not be easily applied in real-world clinical practice given the strict eligibility criteria and controlled setting required in an RCT. Therefore, efficacy in RCTs versus effectiveness in real-world practice may have significant differences including the use of RECIST criteria vs. LiRADS. This efficacy-effectiveness gap has been demonstrated in a recent study involving non-small cell lung cancer (34). Real-world outcomes studies such as ours emphasize the importance of not only analyzing outcomes from RCTs alone but in complementary fashion with real-world studies. They should play a critical role as a benchmark for comparison in assessing response to treatment, OS, and PFS as the next wave of new generation clinical therapeutic trials in HCC treatments are being evaluated.
Limitations
A limiting factor of this study is its retrospective nature with a small sample size of patients enrolled at a single center. With the retrospective nature of this study, this may have introduced lead-time bias in the interpretation of disease progression. Additional limitations include lack of granularity of the tumor size, how tumors were treated with specific Y90 parameters as well as the order and timing of Y90 when other LRT therapies were used. Another limitation is that the radiologists at our institution did not use RECIST criteria to assess radiologic disease progression. Rather, progression was based on standard readings in clinical radiology practice utilizing LiRADS in diagnosis and post treatment assessment of HCC which was introduced in 2011 by the American College of Radiology to standardize the reporting of treated lesions in the US. This may create challenges in comparing our study with prior Y90 studies that have utilized RECIST criteria. However, what could be considered a strength of our study is introducing the differences in practices between real world practice vs. strict parameters of clinical trials by describing the use of LiRADS vs. mRECIST and the potential advantage of using LiRADS over mcRECIST. Our institution at the time of the study did not use BCLC staging as standard practice. Its use is variable and heterogenous throughout the US. This highlights the differences that can be seen between clinical trial and real-world practice and adds further insight regarding our understanding in HCC management. We believe our small study represents a stepping stone in bridging the efficacy-effectiveness gap, and provides further insight between efficacy outcomes and meaningful change in the real-world clinical context. Additional strengths of this study include the setting of a tertiary care center with a robust multidisciplinary HCC program serving a diverse demographic of patients with varying stages of HCC reflecting a real-world clinic practicing setting.
Conclusions
In summary, while this study supports the literature for OS in Y90 patients, we found a shorter PFS in this population. This may reflect the utilization of RECIST in clinical trials in contrast to real-world clinical radiology practice in determining progression utilizing LiRADS criteria. Over time, the most significant factors in our study associated with OS were age, MELD and CP scores, and PVT. For PFS, CP score, and HCC stage at diagnosis were significant. The increasing MELD and CP scores over time likely reflect a combination of REILD, liver decompensation and progression of HCC. The downtrend at 24 months is likely due to long term survivors with significant benefit from therapy with no long-term complications from Y90.
Our single center, “real-world” retrospective study demonstrates that Y90 can be used safely with promising survival rates in properly selected patients. It supports the ongoing critical role of Y90 in the therapeutic armamentarium of HCC. The delicate balance between liver function and tumor burden remains a critical factor in the management of HCC. With the identification of carefully selected patients for such treatments, the priority remains focused on improving overall outcomes and survival. Lastly, outcomes in RCTs versus real-world practice may have significant differences. Real-world studies should play an essential role as a benchmark for comparison in assessing OS and PFS with the next wave of next-generation clinical therapeutic trials in HCC treatments.
Acknowledgments
Funding: None.
Footnote
Reporting Checklist: The authors have completed the STROBE reporting checklist. Available at https://jgo.amegroups.com/article/view/10.21037/jgo-22-882/rc
Data Sharing Statement: Available at https://jgo.amegroups.com/article/view/10.21037/jgo-22-882/dss
Peer Review File: Available at https://jgo.amegroups.com/article/view/10.21037/jgo-22-882/prf
Conflicts of Interest: All authors have completed the ICMJE uniform disclosure form (available at https://jgo.amegroups.com/article/view/10.21037/jgo-22-882/coif). The authors have no conflicts of interest to declare.
Ethical Statement: The authors are accountable for all aspects of the work in ensuring that questions related to the accuracy or integrity of any part of the work are appropriately investigated and resolved. The study was conducted in accordance with the Declaration of Helsinki (as revised in 2013). The study was approved by the Virginia Commonwealth University Institutional Review Board (No. HM20007405) and individual consent for this retrospective analysis was waived.
Open Access Statement: This is an Open Access article distributed in accordance with the Creative Commons Attribution-NonCommercial-NoDerivs 4.0 International License (CC BY-NC-ND 4.0), which permits the non-commercial replication and distribution of the article with the strict proviso that no changes or edits are made and the original work is properly cited (including links to both the formal publication through the relevant DOI and the license). See: https://creativecommons.org/licenses/by-nc-nd/4.0/.
References
- Vogel A, Meyer T, Sapisochin G, et al. Hepatocellular carcinoma. Lancet 2022;400:1345-62. [Crossref] [PubMed]
- McGlynn KA, Petrick JL, El-Serag HB. Epidemiology of Hepatocellular Carcinoma. Hepatology 2021;73:4-13. [Crossref] [PubMed]
- National Program of Cancer Registries. Cancer Surveillance System (NPCR-CSS), Centers for Disease Control and Prevention. Available online: https://www.cdc.gov/cancer/npcr/index.htm
- National Cancer Institute. Surveillance, Epidemiology, and End Results (SEER) Program. Available online: https://seer.cancer.gov
- Kim E, Viatour P. Hepatocellular carcinoma: old friends and new tricks. Exp Mol Med 2020;52:1898-907. [Crossref] [PubMed]
- Mittal S, El-Serag HB. Epidemiology of hepatocellular carcinoma: consider the population. J Clin Gastroenterol 2013;47:S2-6. [Crossref] [PubMed]
- Tapper EB, Parikh ND. Mortality due to cirrhosis and liver cancer in the United States, 1999-2016: observational study. BMJ 2018;362:k2817. [Crossref] [PubMed]
- Estes C, Razavi H, Loomba R, et al. Modeling the epidemic of nonalcoholic fatty liver disease demonstrates an exponential increase in burden of disease. Hepatology 2018;67:123-33. [Crossref] [PubMed]
- Ariel IM. Treatment of inoperable primary pancreatic and liver cancer by the intra-arterial administration of radioactive isotopes (Y90 radiating microspheres). Ann Surg 1965;162:267-78. [Crossref] [PubMed]
- Simon N, Warner RR, Baron MG, et al. Intra-arterial irradiation of carcinoid tumors of the liver. Am J Roentgenol Radium Ther Nucl Med 1968;102:552-61. [Crossref] [PubMed]
- Bierman HR, Byron RL Jr, Kelley KH, et al. Studies on the blood supply of tumors in man. III. Vascular patterns of the liver by hepatic arteriography in vivo. J Natl Cancer Inst 1951;12:107-31. [PubMed]
- Andrews JC, Walker SC, Ackermann RJ, et al. Hepatic radioembolization with yttrium-90 containing glass microspheres: preliminary results and clinical follow-up. J Nucl Med 1994;35:1637-44. [PubMed]
- Dancey JE, Shepherd FA, Paul K, et al. Treatment of nonresectable hepatocellular carcinoma with intrahepatic 90Y-microspheres. J Nucl Med 2000;41:1673-81. [PubMed]
- Houle S, Yip TK, Shepherd FA, et al. Hepatocellular carcinoma: pilot trial of treatment with Y-90 microspheres. Radiology 1989;172:857-60. [Crossref] [PubMed]
- Gray BN, Anderson JE, Burton MA, et al. Regression of liver metastases following treatment with yttrium-90 microspheres. Aust N Z J Surg 1992;62:105-10. [Crossref] [PubMed]
- Gray BN, Burton MA, Kelleher DK, et al. Selective internal radiation (SIR) therapy for treatment of liver metastases: measurement of response rate. J Surg Oncol 1989;42:192-6. [Crossref] [PubMed]
- Salem R, Lewandowski RJ, Mulcahy MF, et al. Radioembolization for hepatocellular carcinoma using Yttrium-90 microspheres: a comprehensive report of long-term outcomes. Gastroenterology 2010;138:52-64. [Crossref] [PubMed]
- Hilgard P, Hamami M, Fouly AE, et al. Radioembolization with yttrium-90 glass microspheres in hepatocellular carcinoma: European experience on safety and long-term survival. Hepatology 2010;52:1741-9. [Crossref] [PubMed]
- Mazzaferro V, Sposito C, Bhoori S, et al. Yttrium-90 radioembolization for intermediate-advanced hepatocellular carcinoma: a phase 2 study. Hepatology 2013;57:1826-37. [Crossref] [PubMed]
- Gordon AC, Gabr A, Riaz A, et al. Radioembolization Super Survivors: Extended Survival in Non-operative Hepatocellular Carcinoma. Cardiovasc Intervent Radiol 2018;41:1557-65. [Crossref] [PubMed]
- Golfieri R, Bilbao JI, Carpanese L, et al. Comparison of the survival and tolerability of radioembolization in elderly vs. younger patients with unresectable hepatocellular carcinoma. J Hepatol 2013;59:753-61. [Crossref] [PubMed]
- Kim DY, Park BJ, Kim YH, et al. Radioembolization With Yttrium-90 Resin Microspheres in Hepatocellular Carcinoma: A Multicenter Prospective Study. Am J Clin Oncol 2015;38:495-501. [Crossref] [PubMed]
- Hamoui N, Ryu RK. Hepatic radioembolization complicated by fulminant hepatic failure. Semin Intervent Radiol 2011;28:246-51. [Crossref] [PubMed]
- Jia Z, Jiang G, Tian F, et al. A systematic review on the safety and effectiveness of yttrium-90 radioembolization for hepatocellular carcinoma with portal vein tumor thrombosis. Saudi J Gastroenterol 2016;22:353-9. [Crossref] [PubMed]
- Mokdad AA, Hester CA, Singal AG, et al. Management of hepatocellular in the United States. Chin Clin Oncol 2017;6:21. [Crossref] [PubMed]
- Salem R, Gabr A, Riaz A, et al. Institutional decision to adopt Y90 as primary treatment for hepatocellular carcinoma informed by a 1,000-patient 15-year experience. Hepatology 2018;68:1429-40. [Crossref] [PubMed]
- Available online: https://www.acr.org/Clinical-Resources/Reporting-and-Data-Systems/LI-RADS/LI-RADS-CT-MRI-v2018 (last accessed Dec 1, 2022).
- Kim DH, Kim B, Choi JI, et al. LI-RADS Treatment Response versus Modified RECIST for Diagnosing Viable Hepatocellular Carcinoma after Locoregional Therapy: A Systematic Review and Meta-Analysis of Comparative Studies. Taehan Yongsang Uihakhoe Chi 2022;83:331-43. [Crossref] [PubMed]
- Saini A, Wallace A, Alzubaidi S, et al. History and Evolution of Yttrium-90 Radioembolization for Hepatocellular Carcinoma. J Clin Med 2019;8:55. [Crossref] [PubMed]
- Spreafico C, Sposito C, Vaiani M, et al. Development of a prognostic score to predict response to Yttrium-90 radioembolization for hepatocellular carcinoma with portal vein invasion. J Hepatol 2018;68:724-32. [Crossref] [PubMed]
- Lau WY, Sangro B, Chen PJ, et al. Treatment for hepatocellular carcinoma with portal vein tumor thrombosis: the emerging role for radioembolization using yttrium-90. Oncology 2013;84:311-8. [Crossref] [PubMed]
- Wehrenberg-Klee E, Gandhi RT, Ganguli S. Patient Selection and Clinical Outcomes of Y90 in Hepatocellular Carcinoma. Tech Vasc Interv Radiol 2019;22:70-3. [Crossref] [PubMed]
- Currie BM, Hoteit MA, Ben-Josef E, et al. Radioembolization-Induced Chronic Hepatotoxicity: A Single-Center Cohort Analysis. J Vasc Interv Radiol 2019;30:1915-23. [Crossref] [PubMed]
- Cramer-van der Welle CM, Verschueren MV, Tonn M, et al. Real-world outcomes versus clinical trial results of immunotherapy in stage IV non-small cell lung cancer (NSCLC) in the Netherlands. Sci Rep 2021;11:6306. [Crossref] [PubMed]