Anti-ferroptotic PRKAA2 serves as a potential diagnostic and prognostic marker for hepatoblastoma
Highlight box
Key findings
• PRKAA2 functions as a tumor-promoting factor in hepatoblastoma and is a potential diagnostic and prognostic marker for hepatoblastoma.
What is known and what is new?
• Ferroptosis is known to play a key role in the development of tumor.
• The clinical value of PRKAA2 for hepatoblastoma was analyzed for the first time. We investigated further the mechanism by which PRKAA2 regulates ferroptosis.
What is the implication, and what should change now?
• An adequate understanding of the relationship between PRKAA2 and ferroptosis can contribute to diagnosis and therapeutic strategy of hepatoblastoma.
Introduction
Hepatoblastoma (HB), an embryonic tumor caused by the abnormal development of liver blastocytes, accounts for ~80% of primary liver malignant tumors in children (1). The annual incidence of HB is about 0.5–1.5/1 million (2), and the incidence is increasing in recent years (3). HB has an insidious onset with rapid progression; most patients are asymptomatic in the early stage. Most patients with HB are diagnosed with abdominal mass accompanied by elevated alpha-fetoprotein (AFP) levels. Effective biomarkers for the diagnosis of HB are lacking, and serum AFP level remains the most important diagnostic and monitoring marker of HB in the clinic (4). However, elevated AFP level is common in benign liver lesions and healthy infants and reduced AFP level occurs in 5–10% of patients with HB (5). This suggests that AFP has a limited role as a biomarker of HB. Complete tumor resection is the main method for treating HB and reducing recurrence (6), but most children cannot undergo radical resection because of the huge liver mass at diagnosis (7). Therefore, understanding the molecular mechanism of HB pathogenesis and identifying effective biomarkers will help in the early diagnosis of HB, development of treatment methods for HB, and improving the prognosis in children with HB.
Ferroptosis, characterized by changes in cellular metabolism in response to the environment, is a type of programmed cell death. Reactive oxygen species (ROS) and polyunsaturated fatty acids are created in high quantities during ferroptosis, leading to phospholipid peroxidation (8,9). It has been claimed that ferroptosis has a role in the occurrence and progression of plenty of diseases in human. Additionally, it has been shown that ferroptosis functions as a tumour suppression mechanism and is connected to the pathophysiology of a number of tumors (10). Iron, amino acids, glutathione (GSH), ROS and lipid peroxidation (LPO) metabolism are all involved in ferroptosis. In 2015, Gao et al. demonstrated that glutamate, glutamine, and transferrin are key regulators of ferroptosis (11). Since then, many studies have shown that several genes and proteins play a role in ferroptosis. Some molecules have a direct or indirect impact on ferroptosis, such as glutathione peroxidase 4 (GPX4) (12), nuclear factor E2-related factor 2 (NRF2) (13), tumor suppressor P53 (TP53) (14), heat shock protein B1 (15), NADPH oxidase (16) and solute carrier family 7 member 1 (SLC7A11) (17). However, studies on ferroptosis in HB are limited, and the role of ferroptosis in HB pathogenesis is unclear. Studies on ferroptosis-related markers in HB may help with HB diagnosis and therapy.
In this work, key ferroptosis-related genes (FRGs) in HB were identified to tackle the issue of lacking of biomarkers. Protein kinase AMP-activated catalytic subunit alpha 2 (PRKAA2), tribbles homolog 2 (TRIB2), and liver-type glutaminase (GLS2) were identified from differentially expressed FRGs (DE-FRGs) in patients with HB. PRKAA2 was selected for functional verification in HB cell lines and in vivo, and the role of PRKAA2 in ferroptosis was preliminarily evaluated. We present this article in accordance with the ARRIVE reporting checklist (available at https://jgo.amegroups.com/article/view/10.21037/jgo-23-110/rc).
Methods
The HB dataset
The Gene Expression Omnibus database portal is where the GSE131329 and GSE75271 files were downloaded. GSE131329, which contains 53 HB samples and fourteen noncancerous liver samples, underwent analysis with the GPL6244 platform, whereas GSE75271, which includes 50 HB samples and five noncancerous liver samples, underwent analysis with the GPL570 platform.
DE-FRGs determination and integrated microarray dataset analyses
Using “limma” in R, differentially expressed genes (DEGs) between tumor and non-tumor liver samples were identified. |Log2FC|>1, P<0.05, and false discovery rate (FDR) <0.05 were thresholds for DEGs (18). GSE131329 and GSE75271 were analyzed separately and the intersection was taken. 29 DE-FRGs were identified by searching the ferroptosis-related database FerrDb (19). Visualisation was conducted using a heatmap by R package “pheatmap”.
Functional annotation and enrichment analyses
To evaluate the functional annotation of candidate genes, Gene Ontology (GO) and Kyoto Encyclopedia of Genes and Genomes (KEGG) pathway analyses were completed using “clusterProfiler” in R (20). All genes were encoded by GO terms and KEGG pathways and analyzed by computational methods. GO terms included biological process, cellular component and molecular function. The P value was corrected using the Benjamini method for multiple testing calibrations. Adjusted P<0.05 were considered significantly enriched. The results were visualised by the R package “ggplot2”.
Selection of potential diagnostic markers
Two machine learning methods for predicting HB status were employed to find potential diagnostic factors. LASSO (least absolute shrinkage and selection operator) is a regression analytical arithmetic that performs regularization to increase prediction accuracy. The “glmnet” package in R was used to conduct LASSO regression analysis to identify the genes substantially associated with the ability of HB and noncancerous liver samples to distinguish one another. Then binomial distribution variables were used and a minimum criterion of standard error value is added to establish the model. Support vector machine (SVM) is a supervised machine learning method, to avoid overfit, recursive feature elimination (RFE) was used to identify optimum genes from the metadata cohort. Hence, SVM-RFE was used to identify the gene set with the greatest discrimination ability. Receiver operating characteristic (ROC) curves and area under the ROC curve (AUC) were generated using the R package “pROC” to investigate the categorizing impacts of the major genes on HB and noncancerous liver specimens.
CIBERSORT analysis
A deconvolution technique based on gene expression profiles is utilized in the computational approach of CIBERSORT (http://cibersort.stanford.edu/), which is used to evaluate changes in a gene group in relation to the other genes in a sample. In noncancerous liver samples and HB samples, CIBERSORT was utilized to determine the immunological responses of 22 immune cells and assess the relationship between the immune cells and the level of important genes. A “signature matrix” of barcode genes that are enriched in each target cell type is needed as input for CIBERSORT. There should be no missing data and all gene expression levels should be in non-log space with positive numbers (21). Upload the correctly formatted mixture file to the website. Then run CIBERSORT and select “LM22 (22 immune cell types)”.
Patients and paired tissue collections
30 pairs of HB samples and adjacent noncancerous samples were collected from patients who underwent HB surgery at Shanghai Children’s Medical Center from September 2019 to June 2022. This study was approved by the Institutional Research Ethical Committee of Shanghai Children’s Medical Centre (No. SCMCIRB-K2021076-1, 2021.10.10). All patients or their guardians provided their verbal and written consent. The study was conducted in accordance with the Declaration of Helsinki (as revised in 2013).
RNA extraction and quantitative reverse transcription polymerase chain reaction (qRT-PCR) analysis
TRIzol reagent (15596018, Invitrogen, Thermo Scientific, Shanghai, China) was used to separate the total RNA from the HB tissue and HB cell lines. Total mRNA was reverse-transcribed into cDNA with the prime script Reverse Transcription reagent kit (RR047, TaKaRa Bio, Shiga, Japan). SYBR Green reagent kit (RR820, TaKaRa Bio, Shiga, Japan) was used to determine the relative expression of mRNA in an ABI 7500 PCR system (Applied Biosystems, Forster City, California, USA). The 2-ΔΔCT method was applied for relative quantitation. As an internal control, 18s rRNA was employed. In the Appendix 1, the primers are provided. Correlation analysis was performed using the ΔΔCT method (22).
Immunohistochemistry
Sections that were embedded in paraffin were deparaffinized with xylene and hydrated using an ethanol gradient. The slices were then transferred to a 10 mmol/L sodium citrate solution for antigen recovery after being treated in 3% methanol-H2O2. The sections were incubated with anti-PRKAA2 antibodies (1/100; Proteintech, 18167-1-AP, RRID:AB_10695046), followed by secondary antibodies (1/1,000; Abcam, ab7090, RRID:AB_955417), after being blocked with 5% skimmed milk powder solution at 25 °C. Color was developed using DAB (3,3N-Diaminobenzidine Tertrahydrochloride), and the film was mounted with neutral gum.
Cell culture, transient transfection and lentivirus transduction
The Cell bank of Chinese Academy of Science (Shanghai, China) provided the HepG2 (RRID: CVCL_1×10), HUH6 (RRID: CVCL_4381) and HEK293T (RRID: CVCL_0063) cells. THLE-2 (RRID: CVCL_3803) was obtained from Ningbo Mingzhou Biotechnology Co., Ltd. (Ningbo, China). HepG2 cells were cultured in minimum Eagle’s medium (MEM) (SH30265.01, HyClone, Logan, UT, USA), HUH6 and HEK293T cells were cultured in Dulbecco’s modified Eagle’s medium (DMEM) (C11995500BT, Gibco Laboratories), and BEBM medium (CC3170, Lonza/Clonetics Corporation, Walkersville, MD, USA) was employed to culture THLE-2 cells. All cells were cultured with 10% fetal bovine serum (FBS) and 1% antibiotic (Gibco, Carlsbad, CA, USA). Specifically, BEBM medium was supplemented with 5 µg/L EGF (SRP3027, Sigma-Aldrich) and 70 µg/L phosphorylethanolamine (HY-N5034, MedChemExpress, Monmouth Junction, NJ, USA). All cultures were kept alive at 37 °C in an incubator with 5% CO2 humidity (Thermo Fisher Scientific, Waltham, MA, USA). Prior to the transfection day, 2.5×105/mL of HepG2 and HUH6 cells were planted in six-well plates and cultured for an overnight period at 40–60% confluence. Lipofectamine 2000 (11668019, Invitrogen, Carlsbad, CA, USA) has been employed to transfect these cells with siRNA targeted against PRKAA2 (GenePharma, Shanghai, China). The cells were then incubated for 48 hours. Target sequences are listed in Appendix 1. For lentivirus transduction, the plasmids and a packaging plasmid were transfected into HEK293T cells; 48 h later, supernatants were obtained and utilized to infect HB cells for 48 h. Finally, HB cells were cultured in the company of 2 mg/L puromycin to obtain stable cell lines.
Western blotting assay
RIPA lysis buffer (P0013B, Beyotime, Jiangsu, China) mixed with phosphatase and protease inhibitors (P1046, Beyotime, Jiangsu, China) was used to lyse the cells on ice. Afterwards, sodium dodecyl sulfate (SDS) was added to the total protein after it had been measured. Then, 40 µg of protein was transferred to nitrocellulose membranes (HATF85R, Millipore, Bedford, MA, USA) after being separated on SDS polyacrylamide gel electrophoresis. The membranes were then blocked and treated with primary antibodies at 4 °C for an overnight period. The membranes were then cleaned and treated with secondary antibodies that had been colored fluorescently (Licor, USA). Finally, an Odyssey equipment (Licor, USA) was used to see the bands on the membranes. The Appendix 1 contains a collection of information about primary antibodies.
Cell proliferation and colony formation assay
Cell counting kit-8 (CCK8) reagent (C0043, Beyotime, Jiangsu, China) was used to measure cell viability. Briefly, the specified treatments were applied to the cells after they were seeded into 96-well plates. Following this, 100 µL of fresh medium containing 10 µL of CCK8 solutions were added to cells. Then the cells were cultured for 2 hours (37 °C, 5% CO2). Afterwards, the absorbance was spectrophotometrically measured at 450 nm. Both the relative cell viability and the collected data were normalized to the corresponding dimethyl sulphoxide-treated wells. Cells (500 cells/well) were sown in six-well plates for colony formation assay and cultured for 1–2 weeks until the emergence of cell colonies. These colonies were then preserved with methanol and stained with Giemsa.
Cell apoptosis analysis
After transfection, 5×105PRKAA2 knockdown cells were collected and washed. After that, cells were stained for 10 minutes with Annexin V that had been fluorescein isothiocyanate-conjugated (C1062M-1, Beyotime, Jiangsu, China), and then for 10 minutes with Propidium Iodide (PI) (C1062M-3, Beyotime, Jiangsu, China). A FACSCanto II flow cytometer (BD, New Jersey, USA) was then used to calculated the proportion of apototic cells.
Xenograft tumor assay
Experiments were performed under a project license (No. SYXK2019-0025, 2022.4.22) granted by the Shanghai Children’s Medical Center, in compliance with institutional guidelines for the care and use of animals. The nude mice were provided by Shanghai Laboratory Animal Center and kept in SPF animal feeding room of Shanghai Children’s Medical Center. The physiological habits of nude mice were fully taken into account in the building of the feeding room, with humidity of 50–60% and temperature of 24 °C. The daily diet of nude mice was uniformly fed and managed by professionals. A xenograft tumor model was established by subcutaneous injection of 4-week-old male nude mice with 1×107 cells. The same batch of mice were purchased and randomly selected for vaccination. Each group contained five mice and the mice were marked with scissor toe. After 25 days, the mice were sacrificed by spinal dislocation and the tumors were weighed. If the nude mice died or became seriously ill during the period, they were not included in the statistics.
Lipid ROS assay
The cells were plated in a six-well plate and incubated with 5 µM BODIPY-C11 (D3861, Thermo Fisher Scientific) for 30 minutes (37 °C, 5% CO2), which was added to the culture media. Using trypsin without ethylene diamine tetraacetie acid, the cells were collected, followed by two phosphate buffered saline (PBS) washes and resuspension in 500 µL PBS. The CytoFLEX cytometer (Beckman Coulter, California, USA) was used to measured ROS levels, and the CytExpert software (Beckman Coulter, California, USA) was used to interpret the results.
Malondialdehyde (MDA), iron and GSH/glutathione oxidized (GSSG) assay
Assessment of the MDA concentration was completed using lipid peroxidation assay kit (ab118970, Abcam, Shanghai, China). GSH and GSSG assay kits (RK05819, ABclonal, Wuhan, China) were used to measure the GSH/GSSG ratio. The determination of intracellular iron level used the iron assay kit (ab83366, Abcam).
Reagents and the concentrations used
From Selleck Chemicals (Houston, Texas, USA), ferrostatin-1 (S7243), Z-VAD-FMK (S7023), and necrosulfonamide (S8251) were bought. HIF-1α-IN-2 (HY-115903) was purchased from MedChemExpress (New Jersey, USA). The concentrations of the stimuli were ferrostatin-1 (2 µM), Z-VAD-FMK (10 µM), necrosulfonamide (0.5 µM), HIF-1α-IN-2 (1 µM).
Statistical analysis
In this study, R software (version 4.0.1), SPSS software (version 24.0), or GraphPad Prism 8 were all used for all statistical analyses. Continuous variables were compared using the Pearson test. The means of two groups were compared using Student’s t-tests. Comparative data between the three groups were compared using one-way analysis of variance. The correlation of PRKAA2 expression and the clinicopathological characteristics of HB patients was examined using Fisher’s exact test. The mean ± standard deviation (mean ± SD) from three independent experiments are used to represent all quantitative data. Unless otherwise stated, a significant difference was considered statistically when P<0.05.
Results
DE-FRGs in HB and functional enrichment analysis
Data from 103 patients with HB and nineteen controls from the GSE131329 (23) and GSE75271 (24) datasets were evaluated retrospectively. As described in the two articles (23,24), tumor samples and noncancerous liver samples were obtained before treatment. DEGs from source data were evaluated using “limma” in R. Then a total of 29 DE-FRGs were identified by searching FerrDb database, of which thirteen were up- and sixteen were downregulated significantly. The expression of 29 DE-FRGs in 103 HB samples compared with that in nineteen controls were shown by the heatmap (Figure 1A). We also performed correlation analysis between these 29 genes. P<0.05 were considered significantly correlate, r value >0 denotes a positive correlation while a r value <0 denotes a negative correlation (Figure 1B). The findings demonstrated that there is dysregulation of ferroptosis-related genes in HB, which may be a key factor in the development of HB.
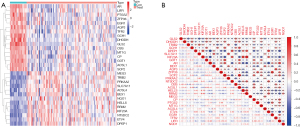
Discovering the connections between fundamental functions or pathways with genes of interest will enhance our understanding of their roles. To study the biological function of the screened FRGs in HB, GO analysis and KEGG analysis were carried out via “clusterProfiler” in R. The results revealed that DE-FRGs mainly participated in biological process, especially response to metal ion, response to nutrient levels and cellular response to metal ion, and were less involved in molecular function and cellular component. KEGG analysis revealed that arginine biosynthesis, ferroptosis, and fatty acid biosynthesis were significantly enriched. Thus, these genes may influence disease progression through lipid metabolism and ferroptosis. Details of the results of GO analysis and KEGG analysis are in Tables 1,2.
Table 1
Module | GO_ID | GO_Term | Count | P value | Genes |
---|---|---|---|---|---|
BP | GO:0010038 | Response to metal ion | 8 | 5.59×10−8 | DPEP1/GOT1/AQP3/PRKAA2/PTGS2/MT1G/TFR2/NQO1 |
GO:0031667 | Response to nutrient levels | 8 | 3.97×10−7 | DHODH/AQP3/ZFP36/PRKAA2/ACSL1/PTGS2/ACSL4/NQO1 | |
GO:0071248 | Cellular response to metal ion | 6 | 4.14×10−7 | DPEP1/PRKAA2/PTGS2/MT1G/TFR2/NQO1 | |
GO:0071241 | Cellular response to inorganic substance | 6 | 9.16×10−7 | DPEP1/PRKAA2/PTGS2/MT1G/TFR2/NQO1 | |
GO:0046394 | Carboxylic acid biosynthetic process | 7 | 1.07×10−6 | GLS2/GCH1/GOT1/SCP2/PRKAA2/CBS/PTGS2 | |
GO:0016053 | Organic acid biosynthetic process | 7 | 1.09×10−6 | GLS2/GCH1/GOT1/SCP2/PRKAA2/CBS/PTGS2 | |
CC | GO:0005777 | Peroxisome | 3 | 0.00087 | SCP2/ACSL1/ACSL4 |
GO:0042579 | Microbody | 3 | 0.00087 | SCP2/ACSL1/ACSL4 | |
GO:0005778 | Peroxisomal membrane | 2 | 0.0031 | ACSL1/ACSL4 | |
GO:0031903 | Microbody membrane | 2 | 0.0031 | ACSL1/ACSL4 | |
GO:0031968 | Organelle outer membrane | 3 | 0.0032 | ACSL1/PTGS2/ACSL4 | |
GO:0019867 | Outer membrane | 3 | 0.0033 | ACSL1/PTGS2/ACSL4 | |
MF | GO:0102391 | Decanoate-CoA ligase activity | 2 | 0.0070 | ACSL1/ACSL4 |
GO:0031956 | MMedium-chain fatty acid-CoA ligase activity | 2 | 0.0070 | ACSL1/ACSL4 | |
GO:0047676 | Arachidonate-CoA ligase activity | 2 | 0.0070 | ACSL1/ACSL4 | |
GO:0004467 | Long-chain fatty acid-CoA ligase activity | 2 | 0.0074 | ACSL1/ACSL4 | |
GO:0003996 | Acyl-CoA ligase activity | 2 | 0.0091 | ACSL1/ACSL4 | |
GO:0015645 | Fatty acid ligase activity | 2 | 0.015 | ACSL1/ACSL4 |
GO, Gene Ontology; BP, biological process; CC, cellular component; MF, molecular function.
Table 2
Pathway ID | Pathway term | Count | P value | Genes |
---|---|---|---|---|
hsa04216 | Ferroptosis | 4 | 3.08×10−6 | SLC7A11/ACSL1/CP/ACSL4 |
hsa01212 | Fatty acid metabolism | 3 | 0.00039 | SCP2/ACSL1/ACSL4 |
hsa04920 | Adipocytokine signaling pathway | 3 | 0.00069 | PRKAA2/ACSL1/ACSL4 |
hsa03320 | PPAR signaling pathway | 3 | 0.00088 | SCP2/ACSL1/ACSL4 |
hsa00061 | Fatty acid biosynthesis | 2 | 0.00094 | ACSL1/ACSL4 |
hsa04146 | Peroxisome | 3 | 0.0011 | SCP2/ACSL1/ACSL4 |
hsa00220 | Arginine biosynthesis | 2 | 0.0014 | GLS2/GOT1 |
hsa00250 | Alanine, aspartate and glutamate metabolism | 2 | 0.0040 | GLS2/GOT1 |
hsa00071 | Fatty acid degradation | 2 | 0.005 | ACSL1/ACSL4 |
hsa01240 | Biosynthesis of cofactors | 3 | 0.0067 | DHODH/GCH1/NQO1 |
hsa00270 | Cysteine and methionine metabolism | 2 | 0.0074 | GOT1/CBS |
KEGG, Kyoto Encyclopedia of Genes and Genomes.
Expression and diagnosis significance of PRKAA2, TRIB2, and GLS2 in HB
Two algorithms were used to select the biomarkers of HB. DE-FRGs were identified using LASSO regression (25), The logistic LASSO regression includes HB as the dependent variable Y, coded 0 for samples without HB, 1 represents samples with HB. The punishment term lambda (λ) was chosen using ten-fold cross-validation. To gauge how well the fitted model performed predictions, binomial deviation was utilized. Eleven variables were identified as diagnostic markers of HB, including GLS2, dihydroorotate dehydrogenase (DHODH), TRIB2, GTP cyclohydrolase 1 (GCH1), aquaporin 3 (AQP3), PRKAA2, 5’-nucleotidase domain containing 2 (NT5DC2), prostaglandin-endoperoxide synthase 2 (PTGS2), metallothionein 1G (MT1G), LIF receptor subunit alpha (LIFR) and NAD(P)H quinone dehydrogenase 1 (NQO1) (Figure S1A,S1B). SVM-RFE is a process that involves iteratively removing features in a backward manner. The process continues until there is only one feature left, providing a list of features in order of importance. During the algorithm, features with the lowest ranking weight are removed, while retaining significant feature variables. Analysis using the SVM-RFE algorithm (26) revealed four subsets of features, including TRIB2, GLS2, PRKAA2 and dipeptidase 1 (DPEP1) (Figure S1C,S1D). Finally, to identify the most valuable DE-FRGs three overlapping features (PRKAA2, TRIB2, and GLS2) were selected between the two algorithms, indicating that these three genes play important roles in HB development (Figure 2A).
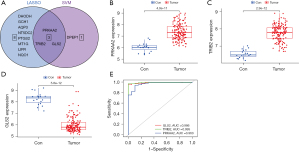
The expression of GLS2 was significantly lower (Figure 2B) and that of PRKAA2 and TRIB2 was significantly higher (Figure 2C,2D) in HB samples than in noncancerous liver samples. To further assess the diagnostic utility of PRKAA2, TRIB2, and GLS2, ROC assays were carried out. ROC curves and AUC were computed using “pROC” in R. When screening HB samples from noncancerous liver samples, the three genes shown a good competence. AUC values for PRKAA2, TRIB2, and GLS2 were 0.963 (95% CI: 0.942–0.985), 0.995 (95% CI: 0.971–1.000), and 0.986 (95% CI: 0.951–1.000), respectively (Figure 2E).
PRKAA2 is a potential prognostic marker for HB
The infiltration of immune cells in the tumor microenvironment is an independent predictor of prognosis. In recent years, ferroptosis and immunity have been revealed to interact extremely closely by a number of studies, with this relationship being critical to the development, progression, and treatment of many malignancies (27-32). Therefore, the status of immune cell infiltration in HB and noncancerous liver samples was evaluated. The CIBERSORT method was employed to calculate the proportion of 22 types of infiltrating immune cells in tissues to evaluate the characteristics of immune cells. Six types of immune cells showed an imbalance between noncancerous liver samples and HB samples. Compared with controls, tumor tissues generally contained a lower fraction of T cells regulatory, NK cells resting, dendritic cells activated and neutrophils and a higher fraction of macrophages M0, and mast cells resting (Figure S2A).
The degree of immune infiltration was examined with the expression of PRKAA2, TRIB2, and GLS2. PRKAA2 mRNA expression is positively correlated with macrophages M1 and mast cell resting and negatively correlated with neutrophils, macrophages M2 and mast cells activated. TRIB2 mRNA expression is positively correlated with NK cells activated and negatively correlated with cells activated and neutrophils. GLS2 mRNA expression is positively correlated with B cells memory and neutrophils and negatively correlated with mast cells resting (Figure S2B). It can be concluded from the results that PRKAA2, TRIB2 and GLS2 were all correlated with immune cells.
PRKAA2 expression was evaluated in tumor tissues and paired adjacent noncancerous liver tissues from 30 patients with HB. Analysis using qRT-PCR and histochemistry revealed that PRKAA2 expression was upregulated in HB samples (Figure 3A,3B). We also evaluated the expression of TRIB2 and GLS2 (Figure S3A-S3D), but there was no difference in the expression of TRIB2 protein between tumor tissue and noncancerous liver tissue. Moreover, we would like to choose genes that promote tumor and can be used as biomarkers and therapeutic targets for research, so PRKAA2 was selected. As shown in Table 3, we collated the clinical information and pathological features of 30 patients with HB, including sex, age at diagnosis, AFP at diagnosis, AFP at final detection, tumor size, histology, PRETEXT (PRE-Treatment EXTent of tumor) stage and PRETEXT annotation factors such as metastasis, vascular involvement, tumor rupture, multifocality, extrahepatic spread and caudate involvement (33). According to the median value of ∆∆Ct [∆∆Ct=∆Ct (normalized noncancerous) − ∆Ct (normalized tumor)], the results of tissue samples determined using qRT-PCR are separated into PRKAA2 low expression group and PRKAA2 high expression group. The RNA level of PRKAA2 was significantly correlated with PRETEXT stage of HB patients (P=0.021) (Table 4). According to the staining intensity of PRKAA2, tissue samples were separated into two groups: PRKAA2 low expression group (−, +) and PRKAA2 high expression group (++, +++). The protein level of PRKAA2 is significantly correlated with serum AFP level at the time of diagnosis (P=0.042), PRETEXT stage (P=0.007), distant metastasis (P=0.014) and multifocality (P=0.017) in HB patients (Table 5). These results suggest that PRKAA2 expression can reflect the progress of HB and are significantly correlated with prognostic factors. Therefore, PRKAA2 expression level may have the potential to be used for prognostic assessment of HB patients.
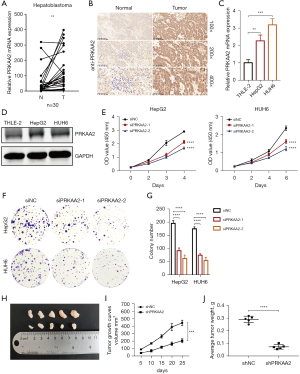
Table 3
Variable | n |
---|---|
Sex | |
Male/female | 15/15 |
Age at diagnosis (months) | |
≥24/<24 | 10/20 |
AFP at diagnosis (ng/mL) | |
≥1,200/<1,200 | 25/5 |
AFP at final detection (ng/mL) | |
≥5/<5/NA | 15/7/8 |
PRETEXT | |
I-II/III-IV/NAv | 16/9/5 |
Histology | |
MIX/E/NA | 14/9/7 |
Tumor size (cm3) | |
≥500/<500 | 16/14 |
Distant metastasis | |
Yes/no | 9/21 |
Vascular involvement | |
Yes/no | 17/13 |
Tumor rupture | |
Yes/no | 4/26 |
Multifocality | |
Yes/no | 6/24 |
Extrahepatic spread | |
Yes/no | 3/27 |
Caudate involvement | |
Yes/no | 13/17 |
AFP, alpha-fetoprotein; NA, unknown; MIX, mixed epithelial and mesenchymal; E, epithelial.
Table 4
Hepatoblastoma (n=30) | PRKAA2 expression | P value | |
---|---|---|---|
Low | High | ||
Sex | 0.715 | ||
Male | 9 | 6 | |
Female | 7 | 8 | |
Age at diagnosis (months) | >0.999 | ||
≥24 | 5 | 5 | |
<24 | 10 | 10 | |
AFP at diagnosis | 0.330 | ||
≥1,200 ng/mL | 11 | 14 | |
<1,200 ng/mL | 4 | 1 | |
AFP at final detection | 0.634 | ||
≥5 ng/mL | 6 | 9 | |
<5 ng/mL | 4 | 3 | |
NA | 5 | 3 | |
PRETEXT | 0.021 | ||
I−II | 11 | 5 | |
III−IV | 1 | 8 | |
NA | 3 | 2 | |
Histology | >0.999 | ||
MIX | 7 | 7 | |
E | 4 | 5 | |
NA | 4 | 3 | |
Tumor size (cm3) | 0.715 | ||
≥500 | 7 | 9 | |
<500 | 8 | 6 | |
Distant metastasis | 0.427 | ||
Yes | 3 | 6 | |
No | 12 | 9 | |
Vascular involvement | 0.462 | ||
Yes | 7 | 10 | |
No | 8 | 5 | |
Tumor rupture | 0.598 | ||
Yes | 1 | 3 | |
No | 14 | 12 | |
Multifocality | 0.169 | ||
Yes | 1 | 5 | |
No | 14 | 10 | |
Extrahepatic spread | >0.999 | ||
Yes | 2 | 1 | |
No | 13 | 14 | |
Caudate involvement | 0.139 | ||
Yes | 4 | 9 | |
No | 11 | 6 |
PRKAA2, protein kinase AMP-activated catalytic subunit alpha 2; AFP, alpha-fetoprotein; NA, unknown; MIX, mixed epithelial and mesenchymal; E, epithelial.
Table 5
Hepatoblastoma (n=30) | PRKAA2 expression | P value | |
---|---|---|---|
Low | High | ||
Sex | 0.466 | ||
Male | 9 | 6 | |
Female | 6 | 9 | |
Age at diagnosis (months) | 0.700 | ||
≥24 | 4 | 6 | |
<24 | 11 | 9 | |
AFP at diagnosis | 0.042 | ||
≥1,200 ng/mL | 10 | 15 | |
<1,200 ng/mL | 5 | 0 | |
AFP at final detection | >0.999 | ||
≥5 ng/mL | 7 | 8 | |
<5 ng/mL | 4 | 3 | |
NA | 4 | 4 | |
PRETEXT | 0.007 | ||
I−II | 10 | 6 | |
III−IV | 1 | 8 | |
NA | 4 | 1 | |
Histology | 0.805 | ||
MIX | 6 | 8 | |
E | 5 | 4 | |
NA | 4 | 3 | |
Tumor size (cm3) | 0.272 | ||
≥500 | 6 | 10 | |
<500 | 9 | 5 | |
Distant metastasis | 0.014 | ||
Yes | 1 | 8 | |
No | 14 | 7 | |
Vascular involvement | |||
Yes | 6 | 11 | 0.139 |
No | 9 | 4 | |
Tumor rupture | |||
Yes | 1 | 3 | 0.598 |
No | 14 | 12 | |
Multifocality | |||
Yes | 0 | 6 | 0.017 |
No | 15 | 9 | |
Extrahepatic spread | >0.999 | ||
Yes | 1 | 2 | |
No | 14 | 13 | |
Caudate involvement | 0.139 | ||
Yes | 4 | 9 | |
No | 11 | 6 |
PRKAA2, protein kinase AMP-activated catalytic subunit alpha 2; AFP, alpha-fetoprotein; NA, unknown; MIX, mixed epithelial and mesenchymal; E, epithelial.
PRKAA2 plays a carcinogenic role in HB through ferroptosis
Comparing HB cells to normal cells, PRKAA2 expression was likewise elevated (Figure 3C,3D). Then, PRKAA2 was knocked down with small interfering RNAs (siRNA) and downregulation of PRKAA2 was verified via western blot and qRT-PCR analysis (Figure S4A,S4B). Results from the CCK-8 assays showed that PRKAA2 silencing significantly reduced the proliferation of both HepG2 and HUH6 cells (Figure 3E). Furthermore, interfering with PRKAA2 expression inhibited colony formation (Figure 3F,3G). Results from flow cytometry analysis revealed that PRKAA2 knockdown increased apoptosis in HepG2 and HUH6 cells (Figure S4C,S4D).
In order to explore whether PRKAA2 can also promote HB cell proliferation in vivo, we conducted tumor formation experiments in nude mice. We selected siPRKAA2-2 because the efficiency of siPRKAA2-2 was better than siPRKAA2-1, and designed and synthesized PRKAA2-knockdown lentiviral plasmids with this sequence. Male nude mice at the age of 4 weeks were injected with 1×107 shNC and shPRKAA2 cells into the left and right upper groins, respectively. The tumor volume was measured for the first time 7 and 10 days after injection, and then was measured every 5 days thereafter. After 25 days, the tumor was removed, weighed and photographed, and the tumor growth curve was drawn. The weight and volume of transplanted tumors were significantly reduced after PRKAA2 knockdown, indicating that PRKAA2 knockdown can significantly inhibit the growth of HB tumors in vivo (Figure 3H-3J).
To further explore the role of PRKAA2 in HB procession, BODIPY-C11 probe, which can precisely detect ferroptosis by counting the quantity of lipid peroxides in cellular membranes, was employed to detect ferroptosis. PRKAA2 silencing increased lipid peroxide levels, indicating an increase in ferroptosis (Figure 4A,4B). The analysis of MDA (Figure 4C), GSH/GSSG ratio (Figure 4D), and iron concentration (Figure 4E) also proved that PRKAA2 knockdown increased ferroptosis. We further confirmed that the cell viability after knockdown of PRKAA2 in HB cells could be partially rescued by Z-VAD-FMK as well as ferrostatin-1 (Figure 4F), which again demonstrated that knockdown of PRKAA2 caused apoptosis as well as ferroptosis in HB cells.
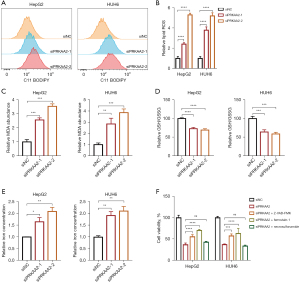
PRKAA2 regulates transferrin receptor 1 (TFR1) through hypoxia-inducible factor 1α (HIF-1α)
Ferroptosis is associated with several metabolism pathways including iron metabolism, lipid metabolism and amino acid metabolism, so we examined some important genes involved in these metabolic pathways after PRKAA2 knockdown. The results showed that the expression of TFR1 increased, while ferritin heavy chain 1 (FTH1), divalent metal transporter 1 (DMT1), ferroportin (FPN), recombinant solute carrier family 7, member 11 (SLC7A11) were no significant change (Figure S5A). TFR1 can transport Fe2+ into cells by binding transferrin (TF), which is the main way for Fe2+ to enter cells (34). The increase of TFR1 may be the reason for the increase of intracellular iron concentration. These results suggest that PRKAA2 knockdown’s ferroptosis induction may be achieved by increasing TFR1 expression to increase intracellular iron concentration in some way. Of course, there are many genes involved that we have not tested, and it is possible that PRKAA2 acts in other ways, too.
Since PRKAA2 knockdown significantly increased the RNA expression of TFR1, we examined whether protein expression was also increased. Consistently, PRKAA2 knockdown enhances the protein expression of TFR1 (Figure 5A). TFR1 expressions are delicately regulated at many levels. IRP1 and IRP2 control the post-transcriptional regulation of the TFR gene (35), while the transcription of TFR1 gene can be induced by hypoxia-inducible factors (HIFs) (36). Therefore, we considered that PRKAA2 may regulate TFR1 expression through IRPs or HIFs. The RNA expression of HIF-1α was up-regulated by PRKAA2 knockdown, and the RNA expression of HIF-2α, IRP1 and IRP2 were no change (Figure 5B). And the results of western blotting were consistent with those of qRT-PCR, PRKAA2 knock down increased HIF-1α expression (Figure 5C). So, we next tried to find out whether the changes in TFR1 expression were related to HIF-1α by inhibiting the up-regulation of HIF-1α. The treatment of the PRKAA2 knockdown cells with HIF-1α inhibitor HIF-1α IN 2 reduced the elevation of TFR1 (Figure 5D). This suggests that PRKAA2 regulates TFR1 indirectly through HIF-1α. Moreover, HIF-1α IN 2 reduced ferroptosis caused by PRKAA2 knockdown (Figure 5E,5F, and Figure S5B,S5C). We also examined the intracellular iron concentration and found that the use of HIF-1α IN 2 also inhibited the increase of iron concentration (Figure S5D). These confirmed the effect of PRKAA2 to negatively modulates ferroptosis by targeting TFR1 through HIF-1α.
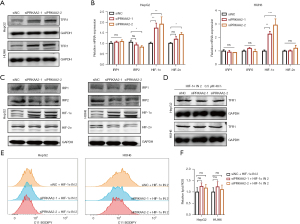
Discussion
In recent years, ferroptosis has attracted a great deal of interest in cancer research. Ferroptosis holds great potential for cancer therapy, for example, LY294002, sirolimus, and wortmannin, among other possible anti-ovarian cancer small molecule medicines, induce ferroptosis, which may lead to the development of new therapeutic alternatives for the treatment of ovarian cancer (37). Cytoglobin (CYGB) is a regulator of ROS, which can promote lipid peroxidation on the cell membrane and is a therapeutic target for colorectal cancer (38). Few researches have looked at ferroptosis in HB cells, despite the fact that it is intimately associated to carcinogenesis and development. Liu et al. demonstrated that m6A modification in HB enhanced HB ferroptosis resistance of SLC7A11, suggesting the immense potential of ferroptosis in HB treatment (39). Studies on HB pathogenesis have mainly focused on the WNT signaling pathway (40), but lack of further research. Investigating the ferroptosis-related markers of HB is of great significance. Mechanistic studies can provide clues for HB novel therapeutic target in the future.
Based on transcription data, bioinformatics-based analysis, expression analysis, and functional verification of DE-FRGs was conducted. Three candidate genes, including PRKAA2, TRIB2, and GLS2, were selected using LASSO regression and SVM-RFE, and they had strong diagnostic efficiency. PRKAA2 significantly affected the proliferation of HB cells, according to functional verification. PRKAA2 is the catalytic component of AMP-activated protein kinase (AMPK), which has complex functions and is an important regulator of energy homeostasis. AMPK maintains the operation of cell physiological activities and participates in a variety of signal transduction pathways (41).
Whether AMPK acts as a tumor suppressor or promoter may depend on the context. AMPK was identified as a tumor suppressor in several studies. For example, dysregulation of AMPK leads to dysphosphorylation of acetyl-CoA carboxylase, which accelerates hepatocellular carcinoma (42). However, there are many other studies suggested that AMPK promotes tumor development. Loss of either PRKAA1 or PRKAA2, or both, in non-small cell lung cancer increased the tumour burden and reduced tumour-free survival (43). According to the literature review on AMPK and tumor by Vara-Ciruelos et al., AMPK acts as a tumor suppressor before tumorigenesis; however, after tumor formation, AMPK transforms into a tumor promoting factor and plays a protective role on tumor cells (44).
In our study, PRKAA2 appears to be a tumor-promoting factor in HB. The results of this research indicate that PRKAA2 is a tumor-promoting factor in HB. In addition to inhibiting proliferation, PRKAA2 inhibits ferroptosis, and knockdown of PRKAA2 increases ferroptosis in HB cells. Therefore, abnormally elevated PRKAA2 may inhibit the ferroptosis in HB cells, thereby promoting progression of HB. AMPK dysregulation may be a potential barrier to cancer treatment. Mechanistically, our results indicated that the regulation of intracellular iron concentration may be the cause of abnormal ferroptosis in HB cells. Although this has not been reported in tumors, studies have shown that iron deposition in vascular dementia is related to the upregulation of TFR1 and AMPK (45). TFR1 expressions are delicately regulated at many levels. TFR gene post-transcriptional regulation is regulated by IRP1 and IRP2 (35). Besides cellular iron, IRP activities can be regulated by some iron-independent effectors such as inflammation (46), oxidative stress (47), and hypoxia (48). The transcription of TFR1 gene can be induced by HIFs (36). In this study, we found PRKAA2 targets TFR1 through HIF-1α, but how PRKAA2 regulates HIF-1α needs further study.
Our results indicate that PRKAA2 expression can reflect the progress of HB and are significantly correlated with prognostic factors. The protein level of PRKAA2 is significantly correlated with serum AFP level at the time of diagnosis (P=0.042), PRETEXT stage (P=0.007), distant metastasis (P=0.014) and multifocality (P=0.017) in HB patients (Table 5). It is very interesting that all 5 cases whose AFP levels were less than 1,200 ng/mL showed low PRKAA2 level. We provide detail about the five patients as Appendix 1. It has been reported that HB patients with an AFP <100 ng/mL had poor prognoses (4). The AFP values of these five patients were all higher than 100 ng/mL, and these 5 patients had no poor prognostic indicator such as PRETEXT stage IV, metastasis and multiple foci of the primary tumor. We hypothesized that low PRKAA2 expression accompanied by low AFP level (1,200 ng/mL > AFP >100 ng/mL) might be a good prognostic factor. However, this needs to be verified by further expanding the sample size. Overall, PRKAA2 expression may be an important prognostic factor for HB.
Ferroptosis is reported to have a strong connection to the immune system (49). Cancer immunotherapy offers cancer patients fresh hope since it works via a different mechanism than conventional radiotherapy, chemotherapy or targeted therapy. In this study, we also discovered some hints that FRGs may influence the degree of HB immune infiltration. This work may serve to provide a fresh light on the molecular processes behind the origin and progression of HB as well as the investigation of prospective targeted treatments for HB, even if the hypothesis needs further clarity. Neutrophils was found to have a lower proportion in tumor samples than controls and negatively correlated with the level of PRKAA2 (Figure S2A). Neutrophils make up a major proportion of leukocytes in the circulation and are key effector cells against cancer, may participate in cellular networks that promote antitumor resistance and mediate antitumor responses by directly destroying tumor cells (50). The role of neutrophils in HB progression and their relationship with PRKAA2 warrant further investigation.
Our research is not exhaustive and has some limitations, for example, the sample size included in this study was not large. What’s more, the mechanism of PRKAA2 regulating ferroptosis deserves further study. In conclusion, we provide the first proof that PRKAA2 regulates ferroptosis in HB and is a potential diagnostic and prognostic marker for HB. Our study provides some clues for the study of ferroptosis in HB, and further research may lead to novel and less aggressive therapeutic modalities for HB.
Conclusions
FRGs might play vital roles in HB development. In HB tissues, the expression of PRKAA2 is significantly up-regulated, and PRKAA2 greater expression is correlated with PRETEXT stage. PRKAA2 functions as a tumor-promoting factor in HB by promoting cell proliferation and prohibiting ferroptosis. Ferroptosis-related genes PRKAA2 is a potential diagnostic and prognostic markers for HB and could serve as a novel therapeutic target in the future.
Acknowledgments
We would like to thank all editors and reviewers for their useful feedback that improved this paper.
Funding: This work was supported by
Footnote
Reporting Checklist: The authors have completed the ARRIVE reporting checklist. Available at https://jgo.amegroups.com/article/view/10.21037/jgo-23-110/rc
Data Sharing Statement: Available at https://jgo.amegroups.com/article/view/10.21037/jgo-23-110/dss
Peer Review File: Available at https://jgo.amegroups.com/article/view/10.21037/jgo-23-110/prf
Conflicts of Interest: All authors have completed the ICMJE uniform disclosure form (available at https://jgo.amegroups.com/article/view/10.21037/jgo-23-110/coif). The authors have no conflicts of interest to declare.
Ethical Statement: The authors are accountable for all aspects of the work in ensuring that questions related to the accuracy or integrity of any part of the work are appropriately investigated and resolved. The study was conducted in accordance with the Declaration of Helsinki (as revised in 2013). The study was approved by the Institutional Research Ethical Committee of Shanghai Children’s Medical Centre (No. SCMCIRB-K2021076-1, 2021.10.10). All patients or their guardians provided their verbal and written consent. Experiments were performed under a project license (No. SYXK2019-0025, 2022.4.22) granted by the Shanghai Children’s Medical Center, in compliance with institutional guidelines for the care and use of animals.
Open Access Statement: This is an Open Access article distributed in accordance with the Creative Commons Attribution-NonCommercial-NoDerivs 4.0 International License (CC BY-NC-ND 4.0), which permits the non-commercial replication and distribution of the article with the strict proviso that no changes or edits are made and the original work is properly cited (including links to both the formal publication through the relevant DOI and the license). See: https://creativecommons.org/licenses/by-nc-nd/4.0/.
References
- Czauderna P, Haeberle B, Hiyama E, et al. The Children's Hepatic tumors International Collaboration (CHIC): Novel global rare tumor database yields new prognostic factors in hepatoblastoma and becomes a research model. Eur J Cancer 2016;52:92-101. [Crossref] [PubMed]
- Lucas B, Ravishankar S, Pateva I. Pediatric Primary Hepatic Tumors: Diagnostic Considerations. Diagnostics (Basel) 2021;11:333. [Crossref] [PubMed]
- Feng J, Polychronidis G, Heger U, et al. Incidence trends and survival prediction of hepatoblastoma in children: a population-based study. Cancer Commun (Lond) 2019;39:62. [Crossref] [PubMed]
- Zhi T, Zhang W, Zhang Y, et al. Clinical Characteristics and Prognosis Analysis of Infantile Hepatoblastoma-A 15-Year Retrospective Single-Center Study. Cancer Manag Res 2021;13:3201-8. [Crossref] [PubMed]
- Fazlollahi L, Hsiao SJ, Kochhar M, et al. Malignant Rhabdoid Tumor, an Aggressive Tumor Often Misclassified as Small Cell Variant of Hepatoblastoma. Cancers (Basel) 2019;11:1992. [Crossref] [PubMed]
- Murawski M, Weeda VB, Czauderna P. Surgical management in hepatoblastoma: points to take. Pediatr Surg Int 2023;39:81. [Crossref] [PubMed]
- Musick SR, Smith M, Rouster AS, et al. Hepatoblastoma. 2023.
- Stockwell BR, Friedmann Angeli JP, Bayir H, et al. Ferroptosis: A Regulated Cell Death Nexus Linking Metabolism, Redox Biology, and Disease. Cell 2017;171:273-85. [Crossref] [PubMed]
- Dixon SJ, Lemberg KM, Lamprecht MR, et al. Ferroptosis: an iron-dependent form of nonapoptotic cell death. Cell 2012;149:1060-72. [Crossref] [PubMed]
- Lei G, Zhuang L, Gan B. Targeting ferroptosis as a vulnerability in cancer. Nat Rev Cancer 2022;22:381-96. [Crossref] [PubMed]
- Gao M, Monian P, Quadri N, et al. Glutaminolysis and Transferrin Regulate Ferroptosis. Mol Cell 2015;59:298-308. [Crossref] [PubMed]
- Zhang Y, Swanda RV, Nie L, et al. mTORC1 couples cyst(e)ine availability with GPX4 protein synthesis and ferroptosis regulation. Nat Commun 2021;12:1589. [Crossref] [PubMed]
- Li W, Fu H, Fang L, et al. Andrographolide induced ferroptosis in multiple myeloma cells by regulating the P38/Nrf2/HO-1 pathway. Arch Biochem Biophys 2023;742:109622. [Crossref] [PubMed]
- Jennis M, Kung CP, Basu S, et al. An African-specific polymorphism in the TP53 gene impairs p53 tumor suppressor function in a mouse model. Genes Dev 2016;30:918-30. [Crossref] [PubMed]
- Wang L, Wu S, He H, et al. CircRNA-ST6GALNAC6 increases the sensitivity of bladder cancer cells to erastin-induced ferroptosis by regulating the HSPB1/P38 axis. Lab Invest 2022;102:1323-34. [Crossref] [PubMed]
- Ding CC, Rose J, Sun T, et al. MESH1 is a cytosolic NADPH phosphatase that regulates ferroptosis. Nat Metab 2020;2:270-7. [Crossref] [PubMed]
- Wang W, Green M, Choi JE, et al. CD8(+) T cells regulate tumour ferroptosis during cancer immunotherapy. Nature 2019;569:270-4. [Crossref] [PubMed]
- Ritchie ME, Phipson B, Wu D, et al. limma powers differential expression analyses for RNA-sequencing and microarray studies. Nucleic Acids Res 2015;43:e47. [Crossref] [PubMed]
- Zhou N, Bao J. FerrDb: a manually curated resource for regulators and markers of ferroptosis and ferroptosis-disease associations. Database (Oxford) 2020;2020:baaa021. [Crossref] [PubMed]
- Wu T, Hu E, Xu S, et al. clusterProfiler 4.0: A universal enrichment tool for interpreting omics data. Innovation (Camb) 2021;2:100141. [Crossref] [PubMed]
- Chen B, Khodadoust MS, Liu CL, et al. Profiling Tumor Infiltrating Immune Cells with CIBERSORT. Methods Mol Biol 2018;1711:243-59. [Crossref] [PubMed]
- Yuan JS, Reed A, Chen F, et al. Statistical analysis of real-time PCR data. BMC Bioinformatics 2006;7:85. [Crossref] [PubMed]
- Hiyama E, Ueda Y, Kurihara S, et al. Gene expression profiling in hepatoblastoma cases of the Japanese study group for pediatric liver tumors-2 (JPLT-2) trial. Eur J Mol Cancer 2019:12-8.
- Sumazin P, Chen Y, Treviño LR, et al. Genomic analysis of hepatoblastoma identifies distinct molecular and prognostic subgroups. Hepatology 2017;65:104-21. [Crossref] [PubMed]
- Lin Q, Zhao Z, Liu JS. Sparse Sliced Inverse Regression Via Lasso. J Am Stat Assoc 2019;114:1726-39. [Crossref] [PubMed]
- Sanz H, Valim C, Vegas E, et al. SVM-RFE: selection and visualization of the most relevant features through non-linear kernels. BMC Bioinformatics 2018;19:432. [Crossref] [PubMed]
- Liao P, Wang W, Wang W, et al. CD8(+) T cells and fatty acids orchestrate tumor ferroptosis and immunity via ACSL4. Cancer Cell 2022;40:365-378.e6. [Crossref] [PubMed]
- Lei G, Horbath A, Li Z, et al. PKCβII-ACSL4 pathway mediating ferroptosis execution and anti-tumor immunity. Cancer Commun (Lond) 2022;42:583-6. [Crossref] [PubMed]
- Jiang Z, Lim SO, Yan M, et al. TYRO3 induces anti-PD-1/PD-L1 therapy resistance by limiting innate immunity and tumoral ferroptosis. J Clin Invest 2021;131:e139434. [Crossref] [PubMed]
- Hsieh CH, Hsieh HC, Shih FS, et al. An innovative NRF2 nano-modulator induces lung cancer ferroptosis and elicits an immunostimulatory tumor microenvironment. Theranostics 2021;11:7072-91. [Crossref] [PubMed]
- Dai E, Han L, Liu J, et al. Autophagy-dependent ferroptosis drives tumor-associated macrophage polarization via release and uptake of oncogenic KRAS protein. Autophagy 2020;16:2069-83. [Crossref] [PubMed]
- Wiernicki B, Maschalidi S, Pinney J, et al. Cancer cells dying from ferroptosis impede dendritic cell-mediated anti-tumor immunity. Nat Commun 2022;13:3676. [Crossref] [PubMed]
- Towbin AJ, Meyers RL, Woodley H, et al. 2017 PRETEXT: radiologic staging system for primary hepatic malignancies of childhood revised for the Paediatric Hepatic International Tumour Trial (PHITT). Pediatr Radiol 2018;48:536-54. [Crossref] [PubMed]
- Sze SCW, Zhang L, Zhang S, et al. Aberrant Transferrin and Ferritin Upregulation Elicits Iron Accumulation and Oxidative Inflammaging Causing Ferroptosis and Undermines Estradiol Biosynthesis in Aging Rat Ovaries by Upregulating NF-Kb-Activated Inducible Nitric Oxide Synthase: First Demonstration of an Intricate Mechanism. Int J Mol Sci 2022;23:12689. [Crossref] [PubMed]
- Gammella E, Buratti P, Cairo G, et al. The transferrin receptor: the cellular iron gate. Metallomics 2017;9:1367-75. [Crossref] [PubMed]
- Udensi UK, Tackett AJ, Byrum S, et al. Proteomics-Based Identification of Differentially Abundant Proteins from Human Keratinocytes Exposed to Arsenic Trioxide. J Proteomics Bioinform 2014;7:166-78. [Crossref] [PubMed]
- Zhang J, Xi J, Huang P, et al. Comprehensive Analysis Identifies Potential Ferroptosis-Associated mRNA Therapeutic Targets in Ovarian Cancer. Front Med (Lausanne) 2021;8:644053. [Crossref] [PubMed]
- Ye S, Xu M, Zhu T, et al. Cytoglobin promotes sensitivity to ferroptosis by regulating p53-YAP1 axis in colon cancer cells. J Cell Mol Med 2021;25:3300-11. [Crossref] [PubMed]
- Liu L, He J, Sun G, et al. The N6-methyladenosine modification enhances ferroptosis resistance through inhibiting SLC7A11 mRNA deadenylation in hepatoblastoma. Clin Transl Med 2022;12:e778. [Crossref] [PubMed]
- Zhang Y, Solinas A, Cairo S, et al. Molecular Mechanisms of Hepatoblastoma. Semin Liver Dis 2021;41:28-41. [Crossref] [PubMed]
- Steinberg GR, Hardie DG. New insights into activation and function of the AMPK. Nat Rev Mol Cell Biol 2023;24:255-72. [Crossref] [PubMed]
- Lally JSV, Ghoshal S, DePeralta DK, et al. Inhibition of Acetyl-CoA Carboxylase by Phosphorylation or the Inhibitor ND-654 Suppresses Lipogenesis and Hepatocellular Carcinoma. Cell Metab 2019;29:174-182.e5. [Crossref] [PubMed]
- La Montagna M, Shi L, Magee P, et al. AMPKalpha loss promotes KRAS-mediated lung tumorigenesis. Cell Death Differ 2021;28:2673-89. [Crossref] [PubMed]
- Vara-Ciruelos D, Russell FM, Hardie DG. The strange case of AMPK and cancer: Dr Jekyll or Mr Hyde? †. Open Biol 2019;9:190099. [Crossref] [PubMed]
- Huo T, Jia Y, Yin C, et al. Iron dysregulation in vascular dementia: Focused on the AMPK/autophagy pathway. Brain Res Bull 2019;153:305-13. [Crossref] [PubMed]
- Charlebois E, Fillebeen C, Katsarou A, et al. A crosstalk between hepcidin and IRE/IRP pathways controls ferroportin expression and determines serum iron levels in mice. Elife 2022;11:e81332. [Crossref] [PubMed]
- Muto Y, Nishiyama M, Nita A, et al. Essential role of FBXL5-mediated cellular iron homeostasis in maintenance of hematopoietic stem cells. Nat Commun 2017;8:16114. [Crossref] [PubMed]
- Anderson SA, Nizzi CP, Chang YI, et al. The IRP1-HIF-2alpha axis coordinates iron and oxygen sensing with erythropoiesis and iron absorption. Cell Metab 2013;17:282-90. [Crossref] [PubMed]
- Wang P, Lu YQ. Ferroptosis: A Critical Moderator in the Life Cycle of Immune Cells. Front Immunol 2022;13:877634. [Crossref] [PubMed]
- Que H, Fu Q, Lan T, et al. Tumor-associated neutrophils and neutrophil-targeted cancer therapies. Biochim Biophys Acta Rev Cancer 2022;1877:188762. [Crossref] [PubMed]