Nestin overexpression reduces the sensitivity of gastric cancer cells to trastuzumab
Highlight box
Key findings
• Nestin overexpression reduced the sensitivity of GC cells to trastuzumab.
What is known and what is new?
• Trastuzumab can help treat HER2-positive breast cancer, trastuzumab resistance is a key clinical challenge. Nestin reportedly regulates the cellular redox homeostasis in lung cancer.
• The inhibitory effect of Nestin on the trastuzumab sensitivity of cells to TRA was confirmed in this study.
What is the implication, and what should change now?
• Antioxidative Nestin-Nrf2 axis may play a role in the mechanism underlying the resistance of GC cells to trastuzumab.
Introduction
Gastric cancer (GC) is the 4th most frequently occurring cancer, is also the 2nd leading attributor for cancer-related mortality worldwide (1). Despite considerable recent progress in cancer therapy owing to advances in molecularly targeted drugs, patients who experience GC recurrence or metastasis have poor prognosis. As a member of the EGFC family, human epidermal growth factor receptor 2 (HER2) (also known as ERBB2) is related to the multiplication, apoptosis, conglutination, migration, and differentiation of tumor cells (2). According to previous studies, HER2 acts as a major biomarker and key factor driving tumorigenesis in GC (3,4), and HER2 overexpression has been detected in 7–34% of gastric tumors (5-7). Recently, the third stage of a large scale clinical study, the ToGA trial, showed a significant survival advantage for HER2-positive GC patients following the administration of a combined therapy consisting of trastuzumab (TRA) and conventional chemotherapy compared with chemotherapy alone. This demonstrates that HER2 is a key target in GC treatment. Although TRA was used in the ToGA trial, 12% of the patients experienced disease progression (8). Additionally, after the administration of TRA in clinical settings in adjuvant and metastatic conditions, HER2-positive breast cancer patients have been reported to survive for a prolonged period (9). However, in the majority of patients who responded to the treatment initially, TRA resistance developed within a year after treatment (10). Zhang et al. investigated the effectiveness of conversion therapy in initially unresectable metastatic cancer with positive HER2 status that responded to chemotherapy plus TRA. They noticed that HER2-positive patients who received conversion gastrectomy after TRA treatment had a good prognosis (11). Even so, some researchers have begun to explore the molecular mechanisms by which breast cancer patients can resist TRA (12,13). As the involvement of similar mechanisms in GC remains unclear, it is very important to explain the mechanisms responsible for sensitivity to TRA, thus helping to develop new therapeutic strategies for GC.
Recently, Nrf2 has been expressed as a key mediator of stress responses in solid tumors (14), and its activation induces resistance to oxidative and xenobiotic stresses (15). Increased levels of Nrf2 have recently been linked to poor prognosis for lung cancer and pancreatic cancer (16,17) owing to chemo/radioresistance or aggressive proliferative phenotypes probably induced by Nrf2 (18,19). In a retrospective study, Nrf2 level was identified as a potential factor for 5-fluorouracil resistance in GC (20). Nrf2 binds to the antioxidant response element (ARE) of glutathione S-transferase (GST), NAD(P)H: quinone oxidoreductase-1 (NQO1), hemeoxygenase-1 (HO-1), and other antioxidant enzymes in the nucleus to upregulate the target gene expression (21). Nrf2 can enhance metabolic gene levels that are conducive to proliferative programs by inducing proliferation via the phosphatidylinositol 3-kinase (PI3K) signaling pathway (22). In solid tumors, PI3K/protein kinase B (AKT) pathway regulation is usually inhibited by activating the nuclear translocation of Nrf2 (23). Gambardella et al. studied the causes of primary and acquired resistance to the combination of TRA and platinum-based chemotherapy (24,25). They discovered that Nrf2 amplified the resistance to anti-HER2 drugs via the PI3K/AKT/mammalian target of rapamycin (mTOR)/Phosphorylation of Ribosomal Protein S6 (RPS6) pathway. RPS6 inhibition reduced Nrf2 expression and restored sensitivity in HER2-amplified GC (24). Brusatol, a Nrf2 suppressor, decreases cancer cell growth by suppressing the Nrf2/HO-1 and HER2-AKT/extracellular regulated kinase 1/2 (ERK1/2) signaling in HER2-positive cancer cells (SK-OV-3 and BT-474 cells) (26). However, its mechanism in HER2-positive cancer cells requires further study.
As reported in a previous study, Nestin, which acts as an intermediate filament protein, is a more specific marker for angiogenesis; additionally, it serves as a therapeutic target by inhibiting the formation of blood vessels (27). Nestin is a marker specific to angiogenesis in malignant tumors, especially in colorectal cancer (28) and prostate cancer (29). The association between HER2-postive GC and Nestin is not reported. However, Nestin showed high expression in basal breast cancer subtype (HER2 negative) but not in the HER2 subtype (HER2 positive) (30). Another study displayed that Nestin showed higher expression rates of triple-negative breast cancers than other breast cancers (31). In GC, Wang et al. (32) uncovered that Nestin protects Nrf2 from Keap1-mediated degradation by competitively binding to the Kelch domain of Keap1 and subsequently upregulating the expression of antioxidant enzymes. Although the effect of chemotherapeutic drugs can be strengthened or cancer cell apoptosis can be induced via the inhibition of Nrf2 expression, the role of Nrf2 in the resistance of GC cells to targeted drugs and its relationship with Nestin remain unclear. These findings linked the Nestin and HER2 positive GC via regulation on Nrf2, and let us hypothesize that Nestin may play a role in TRA sensitivity of HER2-positive GC cells.
The current study investigated possible in vitro and in vivo mechanisms through which anti-HER2 drugs TRA sensitivity in HER2-positive GC models. The findings demonstrate a novel role of Nestin as an upstream mediator of Nrf2 in resisting anti-HER2 TRA in GC. We present this article in accordance with the ARRIVE reporting checklist (available at https://jgo.amegroups.com/article/view/10.21037/jgo-22-1048/rc).
Methods
Cell culture
Using quantitative real-time polymerase chain reaction (qRT-PCR), Nestin-coding gene expression levels were detected in MKN45 and NCI-N87 cells, two HER2-positive GC cell lines provided by American Type Culture Collection (ATCC). Those cells were cultured in BioWhittaker® RPMI-1640 (Gibco, Waltham, USA) cell culture medium supplemented with Sigma-Aldrich’s 10% fetal bovine serum (FBS) (Gibco), stored in a humidity chamber (37 °C, 5% CO2), and then inoculated into 12-well plates for drug treatment. The cells were then grown in TRA-free or 10 µg/mL TRA-containing medium for 96 h.
Animals
Experiments were conducted on female BALB/c nude mice aged 6 weeks and purchased from the Beijing Vital River Laboratory Animal Technology (Beijing, China). All protocols were approved by the Animal Experimentation Ethics Committee of Zhongshan Hospital, Fudan University, in compliance with the 1985 edition (NIH publication No. 86-23) of the Care and Use of Laboratory Animals. During the study period, the investigator minimized the suffering of the mice, reduced the number of mice used, and treated the mice under the guidelines of the Animal Care and Use Committee of Zhongshan Hospital, Fudan University.
Female BALB/c nude mice (4–5 weeks old, 18–20 g) (33,34) were treated with NCI-N87 cells (5×107 per mouse) that had or had not been processed by Nestin overexpression on the right flank via subcutaneous inoculation. On day 10 post inoculation, the average tumor volume was approximately 120 mm3, and the mice were equally randomized into four groups: non-treated control (NTC) group, TRA group, TRA + negative control (NC), and TRA + Nestin (n=6 per group). The peritoneum of mice was injected with TRA for 1 week. After the mice were euthanized via asphyxiation, digital calipers were used to determine the tumor dimensions, and the tumor volumes were calculated as follows: volume = (length × width2)/2.
Terminal deoxynucleotidyl transferase dUTP nick-end labeling (TUNEL) assay
For the assessment of cell apoptosis, TUNEL (Green) assay (Promega, Madison, USA) was employed to stain the sections of tumor tissues that were 5-micrometer thick and freshly frozen. These sections were counterstained with Hoechst diluted at ratio of 1:10,000 (10). Three random fields were counted for TUNEL-positive cells in each slice, and five slices in each group were analyzed under ×200 magnification using a fluorescence microscope.
Lentivirus constructs and infection
pLenti-Nestin and pLenti-empty vectors were derived from the stock form of Escherichia coli (E. coli) and inoculated with LB-carbenicillin at 50 µg/mL (System Biosciences, Palo Alto, USA). Using the 293T cell line and pPACKH1 Lentivector Packaging Kit (System Biosciences), the viral particles were acquired according per manufacturer’s protocol. The viral particles were re-suspended in Dulbecco’s modified Eagle’s medium (DMEM) and stored in cryogenic vials at −80 °C for subsequent use.
Viral particles were used to infect the NCI-N87 cells. According to the green fluorescent protein analysis using flow cytometry, the infection efficiency was approximately 50%. Empty lentivirus (containing empty vector) was used as a NC.
qRT-PCR
RNA was extracted using TRIzol reagent (Invitrogen, Waltham, USA). A PrimeScript RT Reagent kit (Takara, Kusatsu, Japan) was applied to synthesize complementary DNA (cDNA). To identify the amount of mRNA expressed, a SYBR Premix Ex Taq RT-PCR kit (Takara) was used to amplify the cDNA with real-time PCR. Glyceraldehyde-3-phosphate dehydrogenase (GAPDH) expression was used as an internal control. The sequences of primers used in this experiment includes: Nestin F, 5'-AGG CTG AGA ACT CTC GCT TG-3'; Nestin R, 5'-TGA GAA GGA TGT TGG GCT GA-3'; GAPDH F, 5'-GTC TCC TCT GAC TTC AAC AGC G-3'; GAPDH R, 5'-ACC ACC CTG TTG CTG TAG CCA A-3'.
Western blotting (WB)
Cells were lysed in radioimmunoprecipitation assay (RIPA) lysis buffer containing 150 mmol/L NaCl, 0.5% deoxycholic acid sodium salt (w/v), 50 mmol/L Tris-HCl (pH 7.4), 1% NP-40, and 0.1% SDS. These components were then mixed with a phosphatase inhibitor cocktail (10 µL/mL, Sigma) and protease inhibitor cocktail (2 µL/mL, Sigma). Cell lysates underwent the assay with a bicinchoninic acid (BCA) protein kit to determine the protein concentration. After denaturing the total cell lysates, SDS-polyacrylamide gel electrophoresis was performed, followed by immunoblotting of the proteins using horseradish peroxidase (HRP)-conjugated primary and secondary antibodies. After the membrane was washed, the sensitive enhanced chemiluminescence (ECL) reagent (Millipore, Burlington, USA) was used for band detection, and the ChemiDoc imaging system (Bio-Rad Laboratories, Inc., Hercules, USA) was used for visualization.
Cell viability assay
A concentration of 5×103 cells/well was inoculated ino 96-well plates and treated with TRA for a specified period. The cell survival rate was measured using the Cell Counting Kit-8 (CCK-8) kit (Dojindo) and calculated using the formula: [(A450 of experimental group − A450 of background group)/(A450 of control group − A450 of background group)] × 100. Chou and Talalay analysis using Compusyn software was employed to acquire the combination index (CI). Defined by CI values, drug synergism, drug addiction, and antagonistic action were <1.0, =1.0, and >1.0, respectively.
ARE luciferase assay
The experimental procedure involved cloning ARE into a pGL3-basic luciferase reporter plasmid and plating the cells in 12-well plates at a concentration of 1×105 cells/well in triplicate. After a day of culture, the cells were treated with 200 ng of ARE luciferase reporter plasmids using OriGene transfection reagent (Company, City, Country). Following a recovery period of one day in a medium containing 10% FBS, the cells were transfected for 2 days, and the resulting firefly and Renilla luciferase signals were measured and expressed as an increase in activation relative to the single reporter.
Colony formation assay
To assay colony formation, 200 GC cells underwent a 14-day culture in 6-well plates. After the cells were fixed using 4% PFA solution and dyed using 1% crystal violet, the number of colonies composed of more than 50 cells was counted.
Reactive oxygen species (ROS) detection
According to the manufacturer’s instructions, dichloro-dihydro-fluorescein diacetate (DCFH-DA) (Sigma Aldrich) was utilized to routinely detect ROS in solution. Briefly, after treatment with TRA for 48 h, the cells (1×105/well) were supplemented with 1 mM DCFH-DA until DCFH-DA reached a final concentration of 10 µ. Subsequently, the cells were incubated for 30 min and washed with PBS before collection. A flow cytometer (BD Biosciences, San Jose, USA) and 488/525 nm excitation and emission spectra were used to record the fluorescence intensities revealing ROS expression.
Apoptosis detection
Cells were incubated in 6-well plates at a density of 3×105 cells per well with different agents. After 48 h of incubation, the cells were rinsed in ice-cold PBS and incubated for 15 min at room temperature shielded from light with Annexin V/propidium iodide (PI) (Sigma Aldrich). Later, flow cytometer (BD Biosciences) was used to measure the apoptosis rate, and FlowJo software was used for analysis. Annexin V (+)/PI (−) mounted the early stage of apoptotic cells in percentage, and annexin V (+)/PI (+) mounted the late stage.
Statistical analysis
All data are represented as mean ± standard deviation (SD) and compared using analysis of variance (ANOVA) among different groups, while a t-test was performed on data comparison between two groups; P<0.05 was considered statistically significant.
Results
Expression of Nestin was altered in GC cells in response to TRA
To probe the role of Nestin in the anti-tumor activity of TRA in GC cells, we first examined its expression in cells in response to TRA treatment. First, in vitro cytotoxicity assays were performed in MKN45 and NCI-N87 cells that had been treated with or without TRA. The CCK-8 assay showed that TRA administration inhibited the growth of both HER2-positive cell lines in a time-dependent manner (Figure 1A,1B). Furthermore, we observed that Nestin expression in cells treated with TRA gradually decreased with time at both the mRNA and protein levels (Figure 1C-1F). These results imply the potential effect of Nestin on the antitumor activity of TRA.
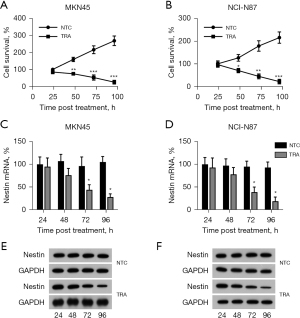
Positive correlation between Nestin expression and activation of HER2/AKT/ERK1/2 and Nrf2/HO-1 signaling
A previous study indicated that TRA treatment inhibited the activation of the HER2/AKT/ERK1/2 and Nrf2/HO-1 pathways (35), and Nestin displayed the capacity to stabilize the expression of Nrf2 protein in GC (32). Therefore, we sought to examine whether Nestin overexpression was also involved in the TRA-repressed activation of these two pathways in HER2-positive GC cells. First, Nestin was overexpressed in the TRA-treated MKN45 and NCI-N87 cell lines, which was verified by qRT-PCR and WB analyses (Figure 2A-2D). Furthermore, WB data showed that the levels of Nrf2; expression of downstream target proteins NQO1, HO-1, and GST; and phosphorylation of ERK1/2, AKT, and HER2 were reduced with TRA treatment, while Nestin overexpression partially reversed the effect of TRA treatment on these indicators (Figure 2C,2D). We also measured ARE luciferase activity to determine Nrf2 activity. The findings revealed that ARE luciferase activity was markedly reduced with TRA treatment, and this reduction could be abolished by Nestin upregulation (Figure 2E,2F). A combination of the data showed a positive relationship between Nestin expression and the activity of Nrf2 and activation of the HER2/AKT/ERK1/2 pathway.
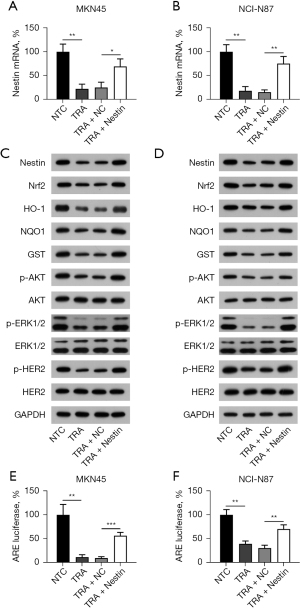
Overexpression of Nestin reduced sensitivity to TRA
To evaluate the Nestin-mediated resistance to TRA, the function of Nestin overexpression in MKN45 and NCI-N87 cells was explored. MKN45 and NCI-N87 cells with or without Nestin overexpression were subjected to cytotoxicity assays. TRA administration exhibited antitumor activities in both cell lines, while the overexpression of Nestin restored cell viability, which was impaired by TRA (Figure 3A,3B). To verify this finding, the colony formation assay showed that TRA nearly eliminated the number of colonies generated by MKN45 and NCI-N87 cells, whereas Nestin overexpression was able to partially recover the number of colonies (Figure 3C,3D), suggesting that Nestin reduced the sensitivity of GC cells to TRA.
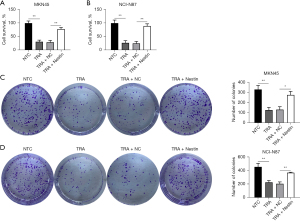
Nestin counteracts TRA-increased ROS production and apoptosis in GC cells
Nrf2 inhibition leads to an increase in ROS, further causing apoptosis (36-38). Accordingly, the level of ROS accumulation was explored after the two cell lines with or without Nestin overexpression were exposed to TRA. Our findings indicated an increase in ROS levels in the two cell lines treated with TRA (Figure 4A,4B). Interestingly, the overexpression of Nestin resulted in an amelioration of ROS accumulation compared to the TRA treatment group (Figure 5A,5B).
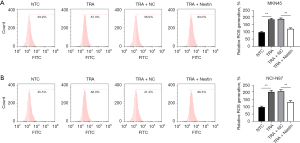
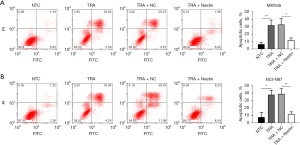
ROS exert antitumor effects by inducing apoptosis (39). Thus, we examined whether Nestin potently prevented TRA-induced apoptosis, as assessed by flow cytometry. As with the findings from ROS generation, the results demonstrated that TRA therapy induced apoptosis in both MKN45 and NCI-N87 cells, compared to the NTC group, while Nestin overexpression alleviated the percentage of apoptotic cells (Figure 5A,5B). A combination of these findings implied that TRA administration enhanced ROS accumulation and accelerated apoptosis in HER2-positive cells by regulating Nestin expression.
Nestin increased chemoresistance of GC tumorigenesis to TRA
According to the results above, it is necessary to study the effect of Nestin on tumor development. NCI-N87 cells were infected with a lentivirus expressing Nestin or with an empty vector and tested to determine whether they were able to develop tumors in vivo. All activities were based on subcutaneous injections to only a few mice (n=6). In mice injected with NCI-N87 cells expressing Nestin, the TRA-reduced average tumor volume was restored significantly (Figure 6A). Furthermore, TRA-reduced tumor weight was markedly increased in the Nestin overexpression group (Figure 6B,6C). Expression levels of Nestin were monitored and found to be downregulated in the TRA treatment group and recovered in the TRA + Nestin group (Figure 6D,6E). In addition, WB analyses also revealed that TRA-deactivated Nrf2, AKT, and ERK1/2 expression was abolished by Nestin overexpression (Figure 6E). Histopathological analysis demonstrated that increased cell apoptosis was induced by TRA and reduced after Nestin upregulation (Figure 6F).
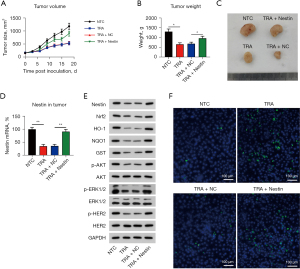
Discussion
This study highlights the role of the Nestin in modulating the sensitivity of GC cells to TRA. Overexpression of Nestin led to resistance to TRA in HER2-positive GC cells by mediating Nrf2-associated ROS production and apoptosis. As far as we know, the ability of Nestin/Nrf2 pathways to modulate the sensitive degree of HER2-positive GC cell lines to TRA was first demonstrated herein.
An increasing number of researchers have proposed molecular mechanisms to clarify the contribution of Nestin to tumor progression in vivo and in vitro. For instance, Li et al. demonstrated a higher transformation of tumor cells and severe genomic instability of Nestin-positive progenitor cells in the cerebellum (40). Tschaharganeh et al. showed that Nestin-expressing progenitor-like cells, derived from hepatocytes, have the potential to evolve into hepatocellular carcinomas (HCCs) or cholangiocarcinomas (41). Several studies also linked the Nestin with chemosensitivity of carcinomas. Zhang et al. investigated the correlation between Nestin expression and chemoresistance of HCC. High Nestin expression was observed in HCC tissues and drug-resistant cell lines, which correlated with poor prognosis. In vivo tumor growth study displayed that Nestin knockdown enhanced chemotherapeutic efficacy in nude mice (42). Similarly, in small cell lung cancer, Nestin expression exhibited a positive correlation with cell proliferation, while conversely displaying a negative correlation with chemosensitivity. Nestin expression in drug-resistant cell lines was upregulated compared to their parental cells (43). These studies underlined an importance of Nestin upregulation in chemoresistance of carcinomas. The present study highlights the critical role of Nestin in the TRA resistance of HER2-positive cells. Nestin expression in HER2-positive GC cells were reduced after TRA administration, accompanying with impairing tumor cell transformation and genomic instability. Furthermore, the phosphorylation of AKT and ERK1/2, which are essential HER2 downstream signals, was found to be positively correlated with the cellular level of Nestin, indicating that at least one of the molecular mechanisms of TRA in HER2-positive cells was challenged by Nestin overexpression. In addition, Nrf2 and its downstream antioxidant effectors were promoted by Nestin upregulation.
Activation of Nrf2 facilitates progressive (44) and metastatic processes in cancer cells (45), and also causes chemoradiotherapy resistance in patients (46). Lapatinib has recently been proven to induce oxidative stress in breast cancer cells and the role of Nrf2 in decreasing the levels of ROS in treated cells (47). In lung and pancreatic cancers, it has been shown that Nrf2 promotes cell metabolism, thereby stimulating cancer progression. The combination of TRA and nimotuzumab can repress the Nrf2 signaling pathway and induce ROS generation, generating a synergistic effect on HER2-positive breast cancers (16,17). In general, these findings indicate the role of Nrf2 as a key target in HER2-positive cancer therapies. According to the latest research, HER2/HER3, Nrf2, and ROS may synergistically regulate breast cancer, ovarian cancer, etc. (48,49). A recent study demonstrated a positive regulatory circle of Nestin and Nrf2 expression in regulating cellular redox homeostasis in lung cancer (32). Here, we observed that Nrf2 expression and activation were clearly promoted by Nestin overexpression in the HER2-positive MKN45 and NCI-N87 cells, as evidenced by increased NQO1, HO1, GST, and ARE luciferase reporter activity. Furthermore, ROS generation in both cell lines in response to TRA incubation was alleviated via Nestin-Nrf2 overexpression, thereby influencing cell apoptosis in vitro and in vivo. However, one of limitations in our study is lacking of validation from patients samples. In further investigation, more detailed data from patients specimen will be utilized to confirm the role of Nestin-Nrf2 in GC.
Conclusions
In summary, the present results demonstrated that Nestin-Nrf2 pathway acts as a possible mechanism inhibiting resistance to TRA in HER2-amplified GC models in vitro and in vivo. Nestin overexpression rendered less benefit from TRA treatment in GC-bearing mice, which suggested the great potential of the combination of adjuvant drugs, such as inhibitor of Nestin or Nrf2, with TRA in treating HER2-positive cancers in the future.
Acknowledgments
Funding: This study was funded by
Footnote
Reporting Checklist: The authors have completed the ARRIVE reporting checklist. Available at https://jgo.amegroups.com/article/view/10.21037/jgo-22-1048/rc
Data Sharing Statement: Available at https://jgo.amegroups.com/article/view/10.21037/jgo-22-1048/dss
Conflicts of Interest: All authors have completed the ICMJE uniform disclosure form (available at https://jgo.amegroups.com/article/view/10.21037/jgo-22-1048/coif). The authors have no conflicts of interest to declare.
Ethical Statement: The authors are accountable for all aspects of the work in ensuring that questions related to the accuracy or integrity of any part of the work are appropriately investigated and resolved. All protocols were approved by the Animal Experimentation Ethics Committee of Zhongshan Hospital, Fudan University, in compliance with the 1985 edition (NIH publication No. 86-23) of the Care and Use of Laboratory Animals. During the study period, the investigator minimized the suffering of the mice, reduced the number of mice used, and treated the mice under the guidelines of the Animal Care and Use Committee of Zhongshan Hospital, Fudan University.
Open Access Statement: This is an Open Access article distributed in accordance with the Creative Commons Attribution-NonCommercial-NoDerivs 4.0 International License (CC BY-NC-ND 4.0), which permits the non-commercial replication and distribution of the article with the strict proviso that no changes or edits are made and the original work is properly cited (including links to both the formal publication through the relevant DOI and the license). See: https://creativecommons.org/licenses/by-nc-nd/4.0/.
References
- Kamangar F, Dores GM, Anderson WF. Patterns of cancer incidence, mortality, and prevalence across five continents: defining priorities to reduce cancer disparities in different geographic regions of the world. J Clin Oncol 2006;24:2137-50. [Crossref] [PubMed]
- Gravalos C, Jimeno A. HER2 in gastric cancer: a new prognostic factor and a novel therapeutic target. Ann Oncol 2008;19:1523-9. [Crossref] [PubMed]
- García I, Vizoso F, Martín A, et al. Clinical significance of the epidermal growth factor receptor and HER2 receptor in resectable gastric cancer. Ann Surg Oncol 2003;10:234-41. [Crossref] [PubMed]
- Begnami MD, Fukuda E, Fregnani JH, et al. Prognostic implications of altered human epidermal growth factor receptors (HERs) in gastric carcinomas: HER2 and HER3 are predictors of poor outcome. J Clin Oncol 2011;29:3030-6. [Crossref] [PubMed]
- Hofmann M, Stoss O, Shi D, et al. Assessment of a HER2 scoring system for gastric cancer: results from a validation study. Histopathology 2008;52:797-805. [Crossref] [PubMed]
- Tanner M, Hollmén M, Junttila TT, et al. Amplification of HER-2 in gastric carcinoma: association with Topoisomerase IIα gene amplification, intestinal type, poor prognosis and sensitivity to trastuzumab. Ann Oncol 2005;16:273-8. [Crossref] [PubMed]
- Janjigian YY, Werner D, Pauligk C, et al. Prognosis of metastatic gastric and gastroesophageal junction cancer by HER2 status: a European and USA International collaborative analysis. Ann Oncol 2012;23:2656-62. [Crossref] [PubMed]
- Bang YJ, Van Cutsem E, Feyereislova A, et al. Trastuzumab in combination with chemotherapy versus chemotherapy alone for treatment of HER2-positive advanced gastric or gastro-oesophageal junction cancer (ToGA): a phase 3, open-label, randomised controlled trial. Lancet 2010;376:687-97. [Crossref] [PubMed]
- Mariani G, Fasolo A, De Benedictis E, et al. Trastuzumab as adjuvant systemic therapy for HER2-positive breast cancer. Nat Clin Pract Oncol 2009;6:93-104. [Crossref] [PubMed]
- Esteva FJ, Valero V, Booser D, et al. Phase II study of weekly docetaxel and trastuzumab for patients with HER-2-overexpressing metastatic breast cancer. J Clin Oncol 2002;20:1800-8. [Crossref] [PubMed]
- Zhang Y, Xu X, Hu C, et al. Trastuzumab in combination with chemotherapy for HER2-positive metastatic gastric cancer patients underwent conversion therapy. Transl Cancer Res 2022;11:2145-56. [Crossref] [PubMed]
- Lu Y, Zi X, Zhao Y, et al. Insulin-like growth factor-I receptor signaling and resistance to trastuzumab (Herceptin). J Natl Cancer Inst 2001;93:1852-7. [Crossref] [PubMed]
- Nagy P, Friedländer E, Tanner M, et al. Decreased accessibility and lack of activation of ErbB2 in JIMT-1, a herceptin-resistant, MUC4-expressing breast cancer cell line. Cancer Res 2005;65:473-82. [Crossref] [PubMed]
- DeNicola GM, Karreth FA, Humpton TJ, et al. Oncogene-induced Nrf2 transcription promotes ROS detoxification and tumorigenesis. Nature 2011;475:106-9. [Crossref] [PubMed]
- Rojo de la Vega M, Chapman E, Zhang DD. NRF2 and the Hallmarks of Cancer. Cancer Cell 2018;34:21-43. [Crossref] [PubMed]
- Best SA, De Souza DP, Kersbergen A, et al. Synergy between the KEAP1/NRF2 and PI3K Pathways Drives Non-Small-Cell Lung Cancer with an Altered Immune Microenvironment. Cell Metab 2018;27:935-43.e4. [Crossref] [PubMed]
- Chio IIC, Jafarnejad SM, Ponz-Sarvise M, et al. NRF2 Promotes Tumor Maintenance by Modulating mRNA Translation in Pancreatic Cancer. Cell 2016;166:963-76. [Crossref] [PubMed]
- Jeong Y, Hoang NT, Lovejoy A, et al. Role of KEAP1/NRF2 and TP53 Mutations in Lung Squamous Cell Carcinoma Development and Radiation Resistance. Cancer Discov 2017;7:86-101. [Crossref] [PubMed]
- Satoh H, Moriguchi T, Takai J, et al. Nrf2 prevents initiation but accelerates progression through the Kras signaling pathway during lung carcinogenesis. Cancer Res 2013;73:4158-68. [Crossref] [PubMed]
- Kang KA, Piao MJ, Ryu YS, et al. Interaction of DNA demethylase and histone methyltransferase upregulates Nrf2 in 5-fluorouracil-resistant colon cancer cells. Oncotarget 2016;7:40594-620. [Crossref] [PubMed]
- Min KJ, Lee JT, Joe EH, et al. An IκBα phosphorylation inhibitor induces heme oxygenase-1(HO-1) expression through the activation of reactive oxygen species (ROS)-Nrf2-ARE signaling and ROS-PI3K/Akt signaling in an NF-κB-independent mechanism. Cell Signal 2011;23:1505-13. [Crossref] [PubMed]
- Dai B, Yoo SY, Bartholomeusz G, et al. KEAP1-dependent synthetic lethality induced by AKT and TXNRD1 inhibitors in lung cancer. Cancer Res 2013;73:5532-43. [Crossref] [PubMed]
- Pavlova NN, Thompson CB. The Emerging Hallmarks of Cancer Metabolism. Cell Metab 2016;23:27-47. [Crossref] [PubMed]
- McCubrey JA, Steelman LS, Chappell WH, et al. Roles of the Raf/MEK/ERK pathway in cell growth, malignant transformation and drug resistance. Biochim Biophys Acta 2007;1773:1263-84. [Crossref] [PubMed]
- Gambardella V, Gimeno-Valiente F, Tarazona N, et al. NRF2 through RPS6 Activation Is Related to Anti-HER2 Drug Resistance in HER2-Amplified Gastric Cancer. Clin Cancer Res 2019;25:1639-49. [Crossref] [PubMed]
- Yang Y, Tian Z, Guo R, et al. Nrf2 Inhibitor, Brusatol in Combination with Trastuzumab Exerts Synergistic Antitumor Activity in HER2-Positive Cancers by Inhibiting Nrf2/HO-1 and HER2-AKT/ERK1/2 Pathways. Oxid Med Cell Longev 2020;2020:9867595. [Crossref] [PubMed]
- Lendahl U, Zimmerman LB, McKay RD. CNS stem cells express a new class of intermediate filament protein. Cell 1990;60:585-95. [Crossref] [PubMed]
- Teranishi N, Naito Z, Ishiwata T, et al. Identification of neovasculature using nestin in colorectal cancer. Int J Oncol 2007;30:593-603. [Crossref] [PubMed]
- Gravdal K, Halvorsen OJ, Haukaas SA, et al. Proliferation of immature tumor vessels is a novel marker of clinical progression in prostate cancer. Cancer Res 2009;69:4708-15. [Crossref] [PubMed]
- Li H, Cherukuri P, Li N, et al. Nestin is expressed in the basal/myoepithelial layer of the mammary gland and is a selective marker of basal epithelial breast tumors. Cancer Res 2007;67:501-10. [Crossref] [PubMed]
- Liu C, Chen B, Zhu J, et al. Clinical implications for nestin protein expression in breast cancer. Cancer Sci 2010;101:815-9. [Crossref] [PubMed]
- Wang J, Lu Q, Cai J, et al. Nestin regulates cellular redox homeostasis in lung cancer through the Keap1-Nrf2 feedback loop. Nat Commun 2019;10:5043. [Crossref] [PubMed]
- Feng HY, Zhang Y, Liu HJ, et al. Characterization of an orthotopic gastric cancer mouse model with lymph node and organ metastases using bioluminescence imaging. Oncol Lett 2018;16:5179-85. [Crossref] [PubMed]
- Matsui Y, Inomata M, Tojigamori M, et al. Suppression of tumor growth in human gastric cancer with HER2 overexpression by an anti-HER2 antibody in a murine model. Int J Oncol 2005;27:681-5. [PubMed]
- Zhou D, Yang Q, Tian T, et al. Gastroprotective effect of gallic acid against ethanol-induced gastric ulcer in rats: Involvement of the Nrf2/HO-1 signaling and anti-apoptosis role. Biomed Pharmacother 2020;126:110075. [Crossref] [PubMed]
- Xiang Y, Ye W, Huang C, et al. Brusatol Enhances the Chemotherapy Efficacy of Gemcitabine in Pancreatic Cancer via the Nrf2 Signalling Pathway. Oxid Med Cell Longev 2018;2018:2360427. [Crossref] [PubMed]
- Sun X, Wang Q, Wang Y, et al. Brusatol Enhances the Radiosensitivity of A549 Cells by Promoting ROS Production and Enhancing DNA Damage. Int J Mol Sci 2016;17:997. [Crossref] [PubMed]
- Kovac S, Angelova PR, Holmström KM, et al. Nrf2 regulates ROS production by mitochondria and NADPH oxidase. Biochim Biophys Acta 2015;1850:794-801. [Crossref] [PubMed]
- Chen P, Luo X, Nie P, et al. CQ synergistically sensitizes human colorectal cancer cells to SN-38/CPT-11 through lysosomal and mitochondrial apoptotic pathway via p53-ROS cross-talk. Free Radic Biol Med 2017;104:280-97. [Crossref] [PubMed]
- Li P, Du F, Yuelling LW, et al. A population of Nestin-expressing progenitors in the cerebellum exhibits increased tumorigenicity. Nat Neurosci 2013;16:1737-44. [Crossref] [PubMed]
- Tschaharganeh DF, Xue W, Calvisi DF, et al. p53-Dependent Nestin Regulation Links Tumor Suppression to Cellular Plasticity in Liver Cancer. Cell 2016;165:1546-7. [Crossref] [PubMed]
- Zhang Y, Zeng S, Ma J, et al. Nestin overexpression in hepatocellular carcinoma associates with epithelial-mesenchymal transition and chemoresistance. J Exp Clin Cancer Res 2016;35:111. [Crossref] [PubMed]
- Sone K, Maeno K, Masaki A, et al. Nestin Expression Affects Resistance to Chemotherapy and Clinical Outcome in Small Cell Lung Cancer. Front Oncol 2020;10:1367. [Crossref] [PubMed]
- Tao S, Rojo de la Vega M, Chapman E, et al. The effects of NRF2 modulation on the initiation and progression of chemically and genetically induced lung cancer. Mol Carcinog 2018;57:182-92. [Crossref] [PubMed]
- Chang CW, Chen YS, Tsay YG, et al. ROS-independent ER stress-mediated NRF2 activation promotes warburg effect to maintain stemness-associated properties of cancer-initiating cells. Cell Death Dis 2018;9:194. [Crossref] [PubMed]
- Wang H, Liu X, Long M, et al. NRF2 activation by antioxidant antidiabetic agents accelerates tumor metastasis. Sci Transl Med 2016;8:334ra51. [PubMed]
- Zhang R, Qiao H, Chen S, et al. Berberine reverses lapatinib resistance of HER2-positive breast cancer cells by increasing the level of ROS. Cancer Biol Ther 2016;17:925-34. [Crossref] [PubMed]
- Kang HJ, Yi YW, Hong YB, et al. HER2 confers drug resistance of human breast cancer cells through activation of NRF2 by direct interaction. Sci Rep 2014;4:7201. [Crossref] [PubMed]
- Khalil HS, Langdon SP, Goltsov A, et al. A novel mechanism of action of HER2 targeted immunotherapy is explained by inhibition of NRF2 function in ovarian cancer cells. Oncotarget 2016;7:75874-901. [Crossref] [PubMed]