Clinical outcomes and toxicities of proton radiotherapy for gastrointestinal neoplasms: a systematic review
Introduction
The use of proton beam radiotherapy (PBT) to treat various cancers is increasing globally. Ten years ago in the US, there were only four proton therapy facilities; as of the writing of this manuscript, 17 centers have become operational in the US, with several more anticipated to come online in the next 2 years. Because of the near-total absence of exit dose, PBT affords excellent dose distributions, especially in the beam-exit path, which permits the use of a very limited number of treatment fields, which further reduces the total, whole-body integral dose. Consequently, in several clinical situations, the dose to organs-at-risk (OARs) is reduced with PBT, and almost uniformly the integral dose is more favorable for PBT, the consequence of which is a potential decline in both acute and long-term radiation-related toxicities (including second malignancies), as well as the toxicities of combined chemoradiotherapy (1,2).
Due to the anatomy of the abdomen, OARs generally lie in close proximity to many GI tumors. The excellent dosimetric profiles, conformality, and ability to spare critical organs and structures make PBT especially attractive as a treatment for gastrointestinal (GI) tumors (3). Due to the dearth of general reviews of this topic (4), we conducted a systematic literature review for published clinical outcomes after PBT for GI neoplasms.
Methods
This systematic review was conducted using the Preferred Reporting Items for Systematic Reviews and Meta-Analyses (PRISMA) guidelines (5). Eligibility criteria included published work in English evaluating clinical outcomes of proton radiation therapy for GI malignancies. Sources of information for this review included PubMed, EMBASE, abstracts from annual meetings of the American Society for Radiation Oncology (ASTRO), Particle Therapy Co-Operative Group (PTCOG), and the American Society of Clinical Oncology (ASCO), those found in references from the major articles identified, and articles known to the authors. The searches were conducted to identify any and all articles addressing clinical outcomes of proton radiotherapy (RT) for GI neoplasms in adults with the following headings: proton, proton radiation therapy, proton beam therapy, pencil beam, GI, anus, anal, anal cancer, biliary, bile duct cancer, cholangiocarcinoma, esophagus, esophageal, esophageal cancer, liver cancer, hepatocellular carcinoma (HCC), metastasis, pancreas, pancreatic, pancreatic cancer, rectum, rectal, rectal cancer, retroperitoneum, retroperitoneal, retroperitoneal sarcoma, stomach, gastric, gastric cancer. Due to the dearth of overall data, search terms were not restricted in terms of publication year or number of patients. Searches were complete by October 15, 2015.
Based on the initial searches, a total of 331 articles/abstracts were identified (Figure 1). Care was taken to ensure that the inclusion criteria were sufficiently broad in order to ensure that possibly pertinent publications were excluded by individual screening rather than the initial database search. In case of journal publications and meeting abstracts being from the same group, the abstract was excluded in favor of the journal article. If updates with larger sample sizes were available from the same group, those were chosen preferentially. Though subgroup analyses were often cited, they were not officially counted in the list of included articles. After duplicates were removed, each of the 283 remaining eligible items was independently screened for the described criteria, and a further 223 were excluded. Articles without specific assessments of clinically relevant outcomes (e.g., survival, toxicity) of proton RT for GI cancers in adults (e.g., medical physics and dosimetric publications) were excluded. Additionally, letters to the editor and direct commentary to other articles were also excluded. Of the 60 publications remaining, an additional 19 were review articles, and though some were cited, they were not included in the primary analysis. Thus, 41 original investigations (28 published articles and 13 abstracts) were found to have sufficient focus and relevance to be incorporated into the review.
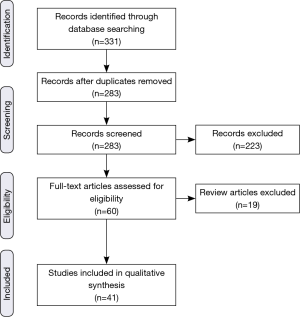
Results
Esophagus
A number of studies consistently report significant dose reduction to nearly all intrathoracic OARs with PBT, and increasing non-comparative clinical data support the efficacy and safety of PBT for esophageal cancer (Table 1). Early PBT reports for esophageal cancer come from Tsukuba University. Sugahara et al. (8) treated 46 patients (45 of whom had squamous cell carcinoma) with PBT and X-ray radiotherapy (XRT) (40/46, 87%) to a median XRT dose of 48 Gray (Gy) and PBT dose of 32 cobalt Gray equivalent (CGE). All patients were treated definitively without chemotherapy, with 22/46 (48%) designated medically inoperable. Five-year local control (LC), disease-specific survival (DSS), and overall survival (OS) was 57%, 67%, and 34% respectively, with median follow-up of 35 months. Grade 3 acute esophagitis occurred in 5 of 46 (11%) patients, with grade 3 late esophageal toxicity in 3 of 46 (7%) patients, and two (4%) cases of grade 5 esophageal toxicity (unspecified cause).
These data are consistent with subsequent publications from the same group (10). In a later report on 51 patients (50 squamous cell carcinoma), 24 of 51 (47%) were treated definitively without chemotherapy and were deemed inoperable. Median XRT dose was 46 Gy and PBT dose 36 CGE; 33 of 51 (65%) received both XRT and PBT, with the remainder receiving median 79 CGE PBT alone. With a median follow-up of 23 months, 5-year LC, progression-free survival (PFS), and OS were 38%, 14%, and 21%, respectively. Grade 3 acute esophagitis was similar to the earlier report (6/51 patients, 12%) with one case (2%) of grade 5 esophageal toxicity (ulcer-related hemorrhage).
The response of esophageal cancer, especially adenocarcinoma, to PBT and chemotherapy has been studied by investigators at M.D. Anderson Cancer Center. Lin et al. examined 62 patients (47/62, 76% with adenocarcinoma) that were treated with a median of 50.4 CGE PBT and concurrent chemotherapy, most commonly with 5-fluorouracil (5-FU) and a taxane or platinum-based chemotherapy (14). Twenty-nine patients (47%) were treated neoadjuvantly. With a median follow-up of 20 months, 3-year locoregional control (LRC), recurrence-free survival (RFS), distant metastasis-free survival (DMFS), and OS were 57%, 52%, 67%, and 52%, respectively. Grade 3 toxicities were as follows: esophagitis (6/62, 10%), dysphagia (6/62, 10%), nausea/emesis (5/62, 8%), dermatitis (2/62, 3%), fatigue (5/62, 8%), anorexia (3/62, 5%), and pneumonitis (1/62, 2%). There were no grade 4 or 5 toxicities with PBT as part of multimodality therapy in this series. Though surgery improved LRC (P=0.005) and RFS (P=0.05), there was no significant association with DM rates (P=0.24) or OS (P=0.33). When comparing 72 PBT-treated patients at the same institution (15) with 208 patients undergoing 3-dimensional conformal radiotherapy (3DCRT) and 164 patients undergoing intensity-modulated radiotherapy (IMRT), all with the aforementioned concurrent chemotherapy, pulmonary complications were 30% in the 3DCRT group, 24% with IMRT, and 14% with PBT (P=0.02 3DCRT vs. PBT; P values for IMRT vs. PBT not given). GI complications were 28% with 3DCRT, 18% with IMRT, and 18% with PBT (P=0.04 3DCRT vs. PBT). Median length of hospital stay was 12 days for 3DCRT, 10 days IMRT, and 8 days PBT (P<0.0001 3DCRT vs. PBT). Though no statistically significant difference between PBT and IMRT was elucidated, given that these were the smallest cohorts and qualitative numerical differences existed, larger sample sizes are needed to determine if PBT is associated with a statistically significant reduction in toxicities compared with IMRT.
The Japanese experience of concurrent cisplatin/5-FU with 60 CGE PBT in 40 squamous cell carcinoma patients treated definitively showed similar results (18). Twenty-four of 40 patients (60%) were medically inoperable, with the remainder refusing surgery. At a median follow-up of 24 months, 2-year LRC, DSS, and OS were 66%, 77%, and 75%, respectively. Three-year OS was 70%, with grade 3 esophagitis in 9/40 (22%) patients and grade 3 skin toxicity in two (5%) patients. Grade 3 late esophageal ulcers occurred in two (5%) patients. There were no grade 4–5 toxicities.
Emerging data presented at PTCOG 2015 (19,20) compared 110 patients that underwent PBT with 472 patients treated with XRT using 3DCRT (n=217) or IMRT (n=255) techniques. Most (535/582, 92%) patients had adenocarcinoma and all underwent concurrent fluoropyrimidine-based chemotherapy with RT (50.4 Gy or CGE). At median 28 months follow-up, 3-year OS was nonsignificantly but numerically lower for XRT (58%) versus 70% for PBT (P= nonsignificant); there was also a trend towards higher 90-day mortality with XRT (4.2% XRT vs. 0.9% PBT, P=0.13). PBT also statistically decreased grade 2 nausea (50% XRT vs. 29% PBT, P<0.001), grade ≥2 fatigue (33% XRT vs. 27% PBT, P<0.001), grade ≥2 hematologic toxicity (26% XRT vs. 2% PBT, P<0.001), postoperative pulmonary complications (28% XRT vs. 14% PBT, P=0.003), postoperative wound complications (15% XRT vs. 5% PBT, P=0.002), and length of hospital stay (12 days XRT vs. 9 days PBT, P<0.0001).
Table 1 lists several other articles examining PBT for esophageal cancers (9,11-13,16,17). Over a decade of published PBT data point to the safety and efficacy of PBT for esophageal cancers, with or without chemotherapy and/or surgery. Furthermore, PBT is beginning to show decreased clinical toxicity as compared to photons, including IMRT, thus actualizing its dosimetric potential; further work and experience is correspondingly needed to more precisely examine differences in PBT and IMRT toxicities. In that context, NRG Oncology is planning a randomized comparison of the two modalities.
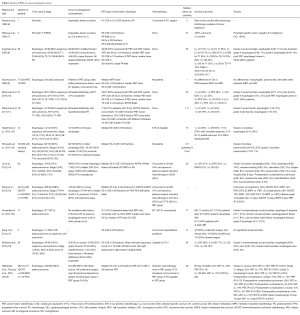
Full table
Stomach
The use of PBT in the management of gastric cancer has been limited to two case reports from Japan (Table 1). One medically inoperable gastric cancer patient was treated with 61 CGE PBT concurrently with 5-FU and tegafur and survived for two months with endoscopic and histologic evidence of tumor regression (6). Two patients with inoperable T1N0M0 cancer were treated with 86 and 83 CGE PBT without chemotherapy (7). Both patients developed persistent ulcers negative for malignancy and were alive at median follow-up of 21 months.
Pancreas
PBT for pancreatic cancer, especially in the pancreatic head, is an attractive option to decrease toxicity to multiple surrounding OARs, including the duodenum, stomach, bowel, liver, and kidneys (Table 2). Translating dosimetric data to evaluating reductions in clinical toxicities, work from the University of Florida (21) examined 22 patients with resected (n=5), borderline resectable (n=5), and unresectable (n=12) tumors who underwent PBT (50.4–59.4 CGE) with concurrent gemcitabine. At median follow-up of 11 months, there were no cases of grade ≥3 GI toxicity; in patients without anterior and left lateral fields, grade 2 GI toxicity was eliminated. Median survival of resected, borderline resectable, and unresectable patients was 11, 14, and 9 months, respectively. The same group examined 15 initially unresectable patients (treated with 59.4 CGE and concurrent capecitabine), of whom five (33%) were able to undergo resection after PBT (25). These patients had a median survival of 24 months; the only postoperative complications were wound infection, ischemic gastritis, and delayed gastric emptying seen in one (2%) patient each. The results are comparable to those of the Proton Cooperative Group registry of 22 patients (22), 8 of which were treated adjuvantly and the remainder definitively (50.4 CGE). With follow-up to 5 months, 9 patients died and there was one case each of grade 3 fatigue and grade 4 thrombocytopenia.
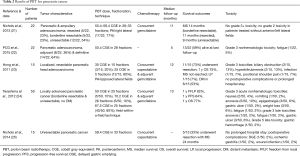
Full table
A phase I report from Harvard used concurrent PBT (25–30 CGE) with capecitabine in 15 resectable patients, followed by adjuvant gemcitabine (23). Eleven underwent resection, 10 of whom were alive at a median 12-month follow-up; median survival was not reached and 1-year OS was 75%. One patient (7%) had local progression and 8/15 patients (53%) developed DM. There were no postoperative complications, and four patients experienced six grade 3 toxicities (biliary obstruction, n=2; hyperbilirubinemia, n=2; infection, n=1; positional shoulder pain, n=1).
A phase I/II study from Japan (24) enrolled 50 locally advanced pancreatic cancer patients in one of three dose levels: 50 CGE (n=5), 70.2 CGE (n=5), or 67.5 CGE (n=40). All patients received concurrent and adjuvant gemcitabine. At a median follow-up of 13 months, 1-year freedom from local progression, PFS, and OS were 82%, 64%, and 77%, respectively. Grade 3 nonhematologic acute toxicities included nausea (n=2), emesis (n=1), anorexia (n=5), epigastralgia (n=3), gastric ulcer (n=1), weight loss (n=3), and fatigue (n=1). Grade 3 late toxicities included anorexia and fatigue in one patient each, and three patients with gastric ulcer, with one death from gastric hemorrhage. Clinically evident gastric ulcers were nearly five times fewer than endoscopically evident gastric ulcers, according to a separate report from the same group (26).
Biliary system & gallbladder
There are some small cohort experiences for the use of PBT for intra- or extrahepatic cholangiocarcinoma. One study utilized postoperative helium and neon ion RT in 22 patients with extrahepatic cholangiocarcinoma (27). Specifically, 16 patients were treated by helium ions (11 of which were treated only with helium ions), which have similar relative biological effectiveness (RBE) as PBT, to a median dose of 60 CGE. These patients were compared with 45 patients undergoing postoperative conventional photon RT (54 Gy) and 62 surgery-only patients. With a minimum follow-up of 5 years and removing palliatively-treated patients, the median survival of the surgery-only and postoperative photon RT group was 16 and 23 months for the PBT group (P=0.13).
Two different institutions in Japan treated cholangiocarcinomas with PBT (Table 3). Ohkawa et al. (38) used PBT (median dose 72.6 CGE) to treat 14 intrahepatic cases (thirteen of which were advanced-stage). Seven patients underwent pyrimidine analog chemotherapy, the timing of which was not mentioned. At a median follow-up of 12 months, 1-year OS was 50%, with two in-field local recurrences (LRs), seven out-of-field intrahepatic failures, and four with DM. There were no late grade ≥3 toxicities and two cases of acute grade ≥3 toxicities (myelosuppression and elevated transaminases).
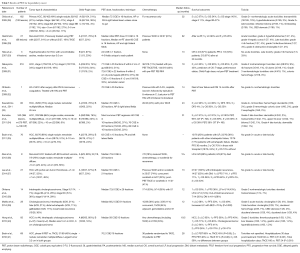
Full table
Makita and colleagues examined 28 patients, 10 of which were locally or regionally recurrent (39). Of the 18 primary tumors, 6 each were intrahepatic and hilar, 3 distal extrahepatic, and 3 gallbladder. Fifteen patients underwent adjuvant pyrimidine analog or gemcitabine-based chemotherapy, and median PBT dose was 68.2 CGE. With a median follow-up of 12 months, 1-year OS was consistent with the prior study at 49%, with PFS 30% and LC 68%. There was a strong correlation between biologically effective dose (BED) >70 Gy and LC (P=0.002). There was one case of grade 3 acute cholangitis and 7 cases of grade 3 late toxicity involving the duodenum or bile ducts. There were no grade 4–5 toxicities.
Liver
There has also been over a decade of published PBT results for the treatment of hepatocellular carcinoma (HCC), much of it from Tsukuba University. In a report from 2005 (28), 162 patients with 192 lesions (84% stages I or II) were treated with a median 72 CGE PBT and followed up for a median 32 months. Five-year LC was 87% and 5-year OS was 24%. The only grade ≥2 non-hematologic acute toxicities were transaminitis (18/185, 10%) and hyperbilirubinemia (3/185, 2%). The grade ≥2 late toxicities of infected biloma and GI bleeding occurred in two patients each (1%), and common bile duct stenosis occurred in one patient (1%).
The group later published a separate experience of 318 patients (31), which remains the largest cohort study to date. These patients were also mostly stage I or II (81%), and received a median 72.6 CGE PBT and followed up for a median 19 months. Though 5-year LC was similar at 83%, 5-year OS was 45%, likely due to more (56%) patients receiving arterial embolization/chemoembolization or other means of pre-PBT tumor control, and/or patients being of lower Child-Pugh class in this latter study. The treatment was very safe, with the only grade 3 nonhematologic toxicities being integumentary and colonic hemorrhage in four and one patients, respectively.
Along with these two high-volume studies, the group has also extensively published subgroup analyses on HCC associated with severe cirrhosis (42,43), severe ascites (30), portal vein tumor thrombosis (44,45), porta hepatis involvement (46), limited treatment options (47), elderly patients (48), LR (29,36), tumor adjacent to the GI tract (49), large-sized tumors (50), and altered fractionation (51,52).
Other reports from Japan have demonstrated similar outcomes. Sixty patients were treated to a median dose of 76 CGE and followed for a median of 43 months (33). Five-year LC, DFS, and OS were 86%, 4%, and 25%, respectively. The only grade 3 toxicity reported in this publication was one patient (2%) with hemorrhagic colonic ulcer. Komatsu and colleagues (34) treated a total of 386 lesions with PBT (n=278) or carbon ion RT (n=108). Treatment regimens were varied but all were significantly hypofractionated at 60–76 CGE in 10–20 fractions. With median follow-up of 31 months, 5-year LC and OS were 91 and 38%, respectively. There were no significant differences in outcomes between carbon ion and PBT. Grade 3 late skin toxicity was reported in four (2%) patients; and transaminitis, upper GI ulcer, and biloma in one patient (1%) each. The only grade 4 toxicity was dermatitis in one (1%) patient.
Phase II data from Loma Linda have been published, with a 76-patient cohort in which 47% of patients were Child-Pugh class B, as opposed to the majority of previous studies in which over 70% of patients are Child-Pugh class A (35). PBT was given at a dose of 63 CGE. Median PFS was 36 months, and 20% of patients recurred. Three-year OS in the 18 patients who underwent liver transplantation was 70%, as compared to 10% in the remainder. Though the poor survival is to be expected in light of the Child-Pugh distributions, no patients experienced grade 3+ acute or late toxicities. This report mirrors other studies demonstrating no grade ≥3 toxicities, presented in Table 3 (32,36,37).
The results of a prospective phase II trial of PBT in 83 patients with unresectable HCC (n=44), intrahepatic cholangiocarcinoma (n=37), or mixed (n=2) have recently been reported (40). The vast majority (80%) of patients had Child-Pugh grade A disease, and prior treatment (most frequently, chemotherapy) had been administered to 32% of HCC and 62% of cholangiocarcinoma patients. Median dose was 58 CGE in 15 fractions. At a median follow-up of 19.5 months (for survivors), 2-year LC was 95% for HCC and 94% for cholangiocarcinoma, with corresponding 2-year PFS of 40% and 26% respectively. OS at 2 years were 63% and 47%, respectively. Treatment was well-tolerated, with the only grade 3 toxicities being (in one patient each) thrombocytopenia, liver disease, gastric ulcer, and hyperbilirubinemia. There were no grade ≥4 toxicities.
The most direct comparison of PBT versus transarterial chemoembolization (TACE) is in press at the time of this manuscript (41). In this phase III trial, 33 patients underwent PBT and 36 received TACE. Clinicopathologic characteristics were similar between groups, and PBT dose was 70.2 CGE in 15 fractions. At a median 28-month follow-up, though there were no differences in OS, there were trends towards improved 2-year LC (88% PBT vs. 45% TACE; P=0.06) and 2-year PFS (48% PBT vs. 31% TACE; P=0.06) in the PBT arm. PBT also decreased the rate and duration of post-treatment hospitalizations (P<0.001). Though still small in sample size, these are the strongest data to date that PBT could be equivalent and likely superior to existing therapies like TACE for certain HCC cases. Further higher-volume trials are highly anticipated as a result.
Rectum
Despite mounting data demonstrating dosimetric superiority in nearly all OARs with PBT, there has not been a clinical toxicity comparison of IMRT and PBT to date. A report from the University of Pennsylvania prospectively evaluated seven patients with recurrent rectal cancer after prior chemoradiotherapy and surgery (53). The median dose of prior RT was 50.4 Gy, and the mean PBT dose was 61.2 CGE. All but one patient underwent concurrent 5-FU. Median follow-up was 19 months; at that time one patient had progressed, five had a partial response, and one a complete response. Two patients recurred locally and one distantly. Adverse effects were notable, with three cases of grade 3 diarrhea and one case of grade 3 abdominal pain. Two patients developed small bowel obstruction (one after salvage surgery), and later developed a rectovaginal fistula.
Next, Vitek and colleagues similarly analyzed six patients with pelvic failures (median prior RT dose 48 Gy) (54). A dose of 39–45 CGE PBT using a single posterior or opposing lateral fields (prone positioning) was employed. Two patients experienced out-of-field progression and reportedly all received “substantial symptom release”. At a median follow-up of one year, two patients of eight (including two patients with retroperitoneal relapse of chordoma and esophageal cancer) died, with no grade ≥3 toxicity reported. To date, there has not been an experience of PBT for primary rectal cancer, however.
Anus
Similar to that of rectal cancer, despite consistent dosimetric data, there has been only one Japanese case report detailing a 7-year recurrence-free interval of PBT (70 CGE) for unresected LR of anorectal cancer with distant metastases; chemotherapy was not given, but two lobectomies were reportedly performed for lung metastases (55). Thus, it is clear that PBT for anorectal cancer offers an open arena to explore clinical outcomes and toxicities to back up dosimetric superiority data.
Metastases
Metastases to the GI system, particularly the liver, make up a substantial proportion of all GI cancers that are treated with PBT (Table 4). Several abstracts from ASTRO and PTCOG by groups in Japan have provided a number of reports of clinical outcomes. Hashimoto et al. (56) treated 52 liver metastases in 35 patients to a median dose of 72.6 CGE. At a median follow-up of 15 months, 2-year OS and LC was 87.2% and 82.1% respectively. New out-of-field metastases developed in 20/35 (57%) of patients, and DM in 13/35 (13%) patients. The only grade ≥3 toxicity was a single patient with a grade 3 gastric ulcer. An update from the same institution (58) with 132 patients displayed 5-year OS of 24% and median survival of 1.6 years. The only late effects observed were cholangitis and rib fracture in one patient each.
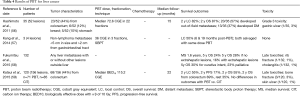
Full table
Another Japanese group presented an experience of 156 liver metastases in 120 patients (59). Though 71/156 (46%) were treated with PBT and the remainder with carbon ions, there were no differences in outcomes between modality. The median BED was 115.2 CGE. At a median 22.3 month follow-up, 2-year LC, PFS, and OS were 55%, 16.7%, and 54.5%, respectively. The only grade ≥3 toxicities were bone fracture in 3/120 (3%) patients and skin ulceration in 1/120 (1%), but it is unreported whether these patients received carbon ion or PBT. This report found statistically improved OS from colorectal primary tumors, which contrasts with no difference seen in another series (56).
A phase I trial at Loma Linda is enrolling patients with liver metastases for stereotactic body proton therapy (SBPT), which reported interim results of four lesions in three patients (57) with 12 CGE in 3 fractions. Two LRs occurred, which were re-irradiated with the same dose. No toxicities have occurred. Though the results are very immature, they underscore the ability of PBT and SBPT to salvage LRs, which remains to be tested in other tumor types.
Hence, in efforts to provide minimal toxicity to patients with GI metastases, PBT remains an attractive option that needs further study. Quality-of-life studies are also warranted to corroborate these clinical outcomes data as well.
Retroperitoneum
Though retroperitoneal cancers are traditionally not classified as GI neoplasms, the anatomic location of these tumors can be associated with significant GI toxicities during treatment. Therefore, PBT has been used for these malignancies (Table 5). Schneider et al. (61) studied 31 patients undergoing retroperitoneal PBT (median dose 72.3 CGE) and correlated dosimetry with no cases of acute grade ≥2 toxicity, although paraspinal tumors were included in addition to retroperitoneal tumors. At a mean follow-up of 5 years, 5-year LC and OS were 52% and 72%, respectively.
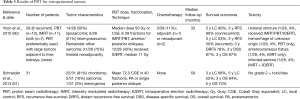
Full table
A report from Harvard described clinical outcomes of PBT and IMRT (60). PBT (n=10), IMRT (n=11), or both (n=7) were used in 28 tumors, 8 of which were recurrent. Three-quarters of RT was delivered neoadjuvantly, and the median dose was 50 Gy for both modalities. Intraoperative electron radiotherapy (IOERT) at a median dose of 11 Gy was used in 12/28 (43%) of patients due to positive posterior margins. With a median follow-up of 33 months, 3-year RFS and LC were 90% for nonrecurrent tumors; these values were 30 and 63%, respectively, for recurrent tumors. The 3-year distant RFS, DSS, and OS were 78%, 87%, and 87% respectively. Unfortunately, outcomes were not stratified between RT modality. Four patients experienced RT-related toxicities, one of which had neoadjuvant PBT alone and the other which had neoadjuvant PBT and IMRT, along with IOERT.
Discussion
To the best of our knowledge, this is the first report that has systematically reviewed the clinical outcomes and toxicities of PBT for GI cancers. The field of PBT has made much advancement in a relatively short amount of time. There is currently dosimetric data for each type of cancer discussed that demonstrates superiority of PBT over various other treatment techniques, including 3DCRT, IMRT, and/or volumetric-modulated arc therapy (VMAT) (62-70). However, determining whether there is a clinical benefit in toxicity profiles or outcomes is a key goal of current and ongoing research, including several prospective clinical trials directly comparing PBT with other RT techniques.
The improved dosimetry of PBT treatment planning has a number of advantages that can be clinically relevant. Less OAR dose can translate to safer dose-escalation in various tumors, which has already been tested for other cancers (71). Aforementioned studies have also demonstrated improved LC with higher BED values (39). Additionally, as the treatment of GI tumors often requires concurrent or sequential multimodality therapy with surgery and/or chemotherapy, PBT may be an ideal RT modality for treating GI malignancies, as it may more safely allow for multimodality therapy (72). Furthermore, because the total body integral dose has been linked to second malignancies (73), the low integral doses given by PBT, especially as compared to modalities such as IMRT or VMAT, may prove to decrease the risk of secondary malignancies (69). Further study is certainly warranted, especially in younger patients with most potential for tumor cure.
Though not a substitute for head-to-head clinical trials, PBT-based therapy for several GI malignancies has shown similar survival and decreased toxicities compared with historical reports. Available PBT/chemotherapy data for esophageal cancer result in similar to improved 5-year OS (50–70%) to the 3-year OS of 58% in the photon-based CROSS trial (74). Grade 3 esophagitis rates (5–12% without concurrent chemotherapy, 10–36% with concurrent chemotherapy) are also favorable to the fluoropyrimidine-based regimen in the CALGB 9781 trial (27% grade 3 esophagitis) (75). Grade 4 esophageal toxicities in the CALGB trial were documented in 15% of patients, a rate higher than has clinically been observed in the PBT studies examined for this review. Postoperative pulmonary (14%) and GI (18%) complications with PBT as reported by Lin et al. (14) are also favorable to photon reports; the pulmonary complication rates in the CROSS and CALGB trials were 33% and 46% respectively, and GI complication rates in those trials were 21% and 22%, respectively. Further encouraging head-to-head comparisons demonstrate clear decreases in both toxicities and postoperative complications of protons as compared with photons (19,20,76).
Regarding HCC, in addition to the multiple studies reporting no grade ≥3 toxicities, support from prospective phase II data (35,40) and a phase III trial (41) demonstrating numerical superiority of PBT have provided high-quality evidence that PBT is, at minimum, equivalent to existing standards of care. In this phase III trial, the decreased post-therapy hospitalization rate provides a pertinent endpoint for which PBT was clearly superior to TACE; these results have major implications for cost-effectiveness (77). Taken together, partially as a result of the relatively greater volume of published data on PBT for HCC, there have been recommendations recently proposed regarding PBT for various “levels” of HCC (incorporating staging, performance and Child-Pugh status, vascular invasion, etc.) (78).
Lastly, albeit with markedly less data than the aforementioned neoplasms, the very low rates of grade 3 nonhematologic toxicities with PBT in pancreatic cancer are also improvements over historical controls. For instance, grade 3 nausea (0–4%), vomiting (0–2%), fatigue (0–5%), and anorexia (0–10%) are generally lower than previous data, which have reported nausea in 16%, vomiting in 11%, and anorexia in 18% (79). Incidences of postoperative complications are also numerically less than that found in existing photon data (80). Additionally, publications of retroperitoneal PBT have produced a total of two toxicities amongst the 48 total patients in both reports, which is in stark contrast to an estimated 39% grade ≥3 toxicity rate seen in photon-based treatment as presented at ASTRO (81).
Because clinical utility of PBT has sprung ahead of corresponding technical study, the importance of medical physics and technological advances is important to incorporate into further outcomes data. For example, PBT beam arrangement (which in some aforementioned studies was relatively fixed/constant for all patients) must be carefully considered in light of individual anatomical OAR variations, as well as tumor size and location (82). Differences in beam arrangements can mean substantial dosimetric differences (83). Optimally accounting for bowel gas and respiratory motion, which if unaccounted for may result in range errors and misdosing of the tumor, is another realm that is increasingly being explored (83,84). Finally, dosimetric and clinical differences in proton pencil beam scanning versus passive scattering have only begun to be investigated (85). With greater time and clinical experience, however, answers to fundamental technical aspects of PBT may leave less outstanding questions and more streamlining of PBT, which can drive down the treatment costs that are known to be a major logistical hurdle for PBT implementation (77).
Aside from esophageal and liver cancer, the overall level of evidence for PBT in GI cancers remains low. However, experiences for these two neoplasms provide appropriate models for further study. In the absence of clinical data supporting the use of PBT for many cancers, evidence can come from cost-effectiveness and quality-of-life analyses as well. To date, there are no such analyses published. It is very plausible, however, that PBT will be shown to be cost-effective in certain circumstances and certain subgroups of patients across a number of GI malignancies. For instance, the 3-day decrease in mean hospital stay in PBT-treated esophageal cancer patients versus photon-treated patients (12 vs. 9 days) could likely translate into significant improvements in cost-effectiveness. Though cost-effectiveness is beyond the scope of this review (77), discerning a difference lies strongly whether clinical toxicities are reduced with a particular intervention, which lends itself well to GI cancers and their often-intimate anatomical relationship with various OARs.
What, then, is the role for PBT in treatment of an intrinsically heterogeneous group of GI malignancies? Based on the available data, despite clear potential advantages, there is no high-level evidence to support its routine for all patients use outside of a clinical protocol, aside from the recent phase III trial which would certainly benefit from longer follow-up (41). This being said, investigators should seek to utilize the paradigm of PBT for HCC—widespread institutional experiences eventually leading to phase II trials, and terminating in a phase III multi-institutional trial in a specific subpopulation. It is likely that PBT may not be the optimal choice in all patients, but careful patient selection of trials is paramount. It is known that clinical trials often select so-called “healthier” patients and exclude those with advanced ages, greater comorbidities, or worse performance status, but perhaps this is the subset that would benefit most from PBT and in which marginally improved differences in clinical toxicities could be discerned. Moreover, results can be enhanced by selection of nontraditional endpoints, such as hospitalization rates, cost-effectiveness, and/or quality of life. This notion is not particularly novel, as multiple ongoing PBT trials are utilizing similar endpoints. It would also exploit the fact that several GI neoplasms are often treated neoadjuvantly (e.g., rectal, pancreatic, and esophageal cancers), and improved local dosimetry could translate to meaningful differences in various operative/postoperative endpoints such has been demonstrated in esophageal cancer (19,20). In all likelihood, however, encouraging data from even one phase II/III trial can provide great evidence for further multi-institutional analyses with larger sample sizes and hence potentially increased sensitivities to detect differences in various outcomes. Hence, accrual and completion of several ongoing phase II/III studies is highly anticipated.
Conclusions
In summary, GI malignancies offer an exciting realm to actualize the great potential of PBT by precisely treating the appropriate tumor while minimizing surrounding OAR dose. We encourage publications of clinical outcomes of PBT for GI cancers in efforts to determine optimal oncologic care of patients with GI neoplasms, while striving to minimize morbidities and maximize quality of life.
Acknowledgements
None.
Footnote
Conflicts of Interest: MP Mehta has served as a consultant for Abbott, Bristol-Meyers-Squibb, Celldex, Cavion, Elekta, Novartis, Novocure, and Roche, has research funding from Novocure and Cellectar, and has served in a leadership capacity on the Pharmacyclics BOD (with stock options). SH Lin has research funding from Elekta, STCube Pharmaceuticals, Peregrine, Bayer, and Roche/Genentech, has served as consultant for AstraZeneca, and received honorarium from US Oncology and ProCure. The other authors have no conflicts of interest to declare.
References
- Verma V, Mehta MP. Clinical Outcomes of Proton Radiotherapy for Uveal Melanoma. Clin Oncol (R Coll Radiol) 2016;28:e17-e27. [Crossref] [PubMed]
- Verma V, Shah C, Mehta MP. Clinical Outcomes and Toxicity of Proton Radiotherapy for Breast Cancer. Clin Breast Cancer 2016;16:145-54. [Crossref] [PubMed]
- Plastaras JP, Dionisi F, Wo JY. Gastrointestinal cancer: nonliver proton therapy for gastrointestinal cancers. Cancer J 2014;20:378-86. [Crossref] [PubMed]
- Ask A, Johansson B, Glimelius B. The potential of proton beam radiation therapy in gastrointestinal cancer. Acta Oncol 2005;44:896-903. [Crossref] [PubMed]
- Moher D, Liberati A, Tetzlaff J, et al. Preferred reporting items for systematic reviews and meta-analyses: the PRISMA statement. Int J Surg 2010;8:336-41. [Crossref] [PubMed]
- Koyama S, Kawanishi N, Fukutomi H, et al. Advanced carcinoma of the stomach treated with definitive proton therapy. Am J Gastroenterol 1990;85:443-7. [PubMed]
- Shibuya S, Takase Y, Aoyagi H, et al. Definitive proton beam radiation therapy for inoperable gastric cancer: a report of two cases. Radiat Med 1991;9:35-40. [PubMed]
- Sugahara S, Tokuuye K, Okumura T, et al. Clinical results of proton beam therapy for cancer of the esophagus. Int J Radiat Oncol Biol Phys 2005;61:76-84. [Crossref] [PubMed]
- Xiaomao G, Liao ZX, Komaki R, et al. Concurrent chemotherapy and proton beam therapy for esophageal cancer. Int J Radiat Oncol Biol Phys 2009;75:S289-290. [Crossref]
- Mizumoto M, Sugahara S, Nakayama H, et al. Clinical results of proton-beam therapy for locoregionally advanced esophageal cancer. Strahlenther Onkol 2010;186:482-8. [Crossref] [PubMed]
- Mizumoto M, Sugahara S, Okumura T, et al. Hyperfractionated concomitant boost proton beam therapy for esophageal carcinoma. Int J Radiat Oncol Biol Phys 2011;81:e601-6. [Crossref] [PubMed]
- Hashimoto T, Ishikawa H, Okumura T, et al. Concurrent chemo-proton therapy using 5-fluorouracil and cisplatin for esophageal cancer. Int J Radiat Oncol Biol Phys 2012;84:S311-312. [Crossref]
- Echeverria AE, McCurdy M, Castillo R, et al. Proton therapy radiation pneumonitis local dose-response in esophagus cancer patients. Radiother Oncol 2013;106:124-9. [Crossref] [PubMed]
- Lin SH, Komaki R, Liao Z, et al. Proton beam therapy and concurrent chemotherapy for esophageal cancer. Int J Radiat Oncol Biol Phys 2012;83:e345-51. [Crossref] [PubMed]
- Wang J, Wei C, Tucker SL, et al. Predictors of postoperative complications after trimodality therapy for esophageal cancer. Int J Radiat Oncol Biol Phys 2013;86:885-91. [Crossref] [PubMed]
- Fernandes A, Berman AT, Mick R, et al. A Prospective Study of Proton Beam Reirradiation for Esophageal Cancer. Int J Radiat Oncol Biol Phys 2016;95:483-7. [Crossref] [PubMed]
- Kang JI, Wallen J, Mirshahidi H, et al. Early progress on a phase II study of proton chemotherapy for resectable esophageal cancer. Presented at Particle Therapy Co-Operative Group North America 1st Annual Meeting. Houston, Texas, 27-29 October 2014.
- Ishikawa H, Hashimoto T, Moriwaki T, et al. Proton beam therapy combined with concurrent chemotherapy for esophageal cancer. Anticancer Res 2015;35:1757-62. [PubMed]
- Hallemeier CL, Chuong M, Merrell KW, et al. Impact of neoadjuvant proton vs. photon chemoradiotherapy (CRT) on post-operative outcomes in patients with esophageal cancer treated with trimodality therapy – a multi-institutional analysis. Presented at Particle Therapy Co-Operative Group North America 2nd Annual Meeting. San Diego, California, 18-23 May 2015.
- Chuong MD, Bhooshan N, Allen PK, et al. A multi-institutional analysis of acute toxicity after neoadjuvant chemoradiation using photons or protons in trimodality esophageal cancer patients. Presented at Particle Therapy Co-Operative Group North America 2nd Annual Meeting. San Diego, California, 18-23 May 2015.
- Nichols RC Jr, George TJ, Zaiden RA Jr, et al. Proton therapy with concomitant capecitabine for pancreatic and ampullary cancers is associated with a low incidence of gastrointestinal toxicity. Acta Oncol 2013;52:498-505. [Crossref] [PubMed]
- Proton Cooperative Group. Proton Cooperative Group registry of patients treated for pancreatic cancer – enrollment and preliminary outcomes. Presented at Particle Therapy Co-Operative Group North America 2nd Annual Meeting. San Diego, California, 18-23 May 2015.
- Hong TS, Ryan DP, Blaszkowsky LS, et al. Phase I study of preoperative short-course chemoradiation with proton beam therapy and capecitabine for resectable pancreatic ductal adenocarcinoma of the head. Int J Radiat Oncol Biol Phys 2011;79:151-7. [Crossref] [PubMed]
- Terashima K, Demizu Y, Hashimoto N, et al. A phase I/II study of gemcitabine-concurrent proton radiotherapy for locally advanced pancreatic cancer without distant metastasis. Radiother Oncol 2012;103:25-31. [Crossref] [PubMed]
- Nichols RC, Morris CG, Bose D, et al. Feasibility of pancreatectomy after high dose proton therapy for unresectable pancreatic cancer. Presented at Particle Therapy Co-Operative Group North America 1st Annual Meeting. Houston, Texas, 27-29 October 2014.
- Takatori K, Terashima K, Yoshida R, et al. Upper gastrointestinal complications associated with gemcitabine-concurrent proton radiotherapy for inoperable pancreatic cancer. J Gastroenterol 2014;49:1074-80. [Crossref] [PubMed]
- Schoenthaler R, Phillips TL, Castro J, et al. Carcinoma of the extrahepatic bile ducts. The University of California at San Francisco experience. Ann Surg 1994;219:267-74. [Crossref] [PubMed]
- Chiba T, Tokuuye K, Matsuzaki Y, et al. Proton beam therapy for hepatocellular carcinoma: a retrospective review of 162 patients. Clin Cancer Res 2005;11:3799-805. [Crossref] [PubMed]
- Hashimoto T, Tokuuye K, Fukumitsu N, et al. Repeated proton beam therapy for hepatocellular carcinoma. Int J Radiat Oncol Biol Phys 2006;65:196-202. [Crossref] [PubMed]
- Hata M, Tokuuye K, Sugahara S, et al. Proton irradiation in a single fraction for hepatocellular carcinoma patients with uncontrollable ascites. Technical considerations and results. Strahlenther Onkol 2007;183:411-6. [Crossref] [PubMed]
- Nakayama H, Sugahara S, Tokita M, et al. Proton beam therapy for hepatocellular carcinoma: the University of Tsukuba experience. Cancer 2009;115:5499-506. [Crossref] [PubMed]
- Ohtsubo K, Watanabe H, Tsuchiyama T, et al. Advanced hepatocellular carcinoma treated effectively with irinotecan via hepatic arterial infusion followed by proton beam therapy. J Infect Chemother 2009;15:316-21. [Crossref] [PubMed]
- Kawashima M, Kohno R, Nakachi K, et al. Dose-volume histogram analysis of the safety of proton beam therapy for unresectable hepatocellular carcinoma. Int J Radiat Oncol Biol Phys 2011;79:1479-86. [Crossref] [PubMed]
- Komatsu S, Fukumoto T, Demizu Y, et al. Clinical results and risk factors of proton and carbon ion therapy for hepatocellular carcinoma. Cancer 2011;117:4890-904. [Crossref] [PubMed]
- Bush DA, Kayali Z, Grove R, et al. The safety and efficacy of high-dose proton beam radiotherapy for hepatocellular carcinoma: a phase 2 prospective trial. Cancer 2011;117:3053-9. [Crossref] [PubMed]
- Abei M, Okumura T, Fukuda K, et al. A phase I study on combined therapy with proton-beam radiotherapy and in situ tumor vaccination for locally advanced recurrent hepatocellular carcinoma. Radiat Oncol 2013;8:239. [Crossref] [PubMed]
- Lee SU, Park JW, Kim TH, et al. Effectiveness and safety of proton beam therapy for advanced hepatocellular carcinoma with portal vein tumor thrombosis. Strahlenther Onkol 2014;190:806-14. [Crossref] [PubMed]
- Ohkawa A, Mizumoto M, Ishikawa H, et al. Proton beam therapy for unresectable intrahepatic cholangiocarcinoma. J Gastroenterol Hepatol 2015;30:957-63. [Crossref] [PubMed]
- Makita C, Nakamura T, Takada A, et al. Clinical outcomes and toxicity of proton beam therapy for advanced cholangiocarcinoma. Radiat Oncol 2014;9:26. [Crossref] [PubMed]
- Hong TS, Wo JY, Yeap BY, et al. Multi-institutional phase II study of high-dose hypofractionated proton beam therapy in patients with localized, unresectable hepatocellular carcinoma and intrahepatic cholangiocarcinoma. J Clin Oncol 2016;34:460-8. [Crossref] [PubMed]
- Bush DA, Smith JC, Slater JD, et al. Randomized clinical trial comparing proton beam radiation therapy with transarterial chemoembolization for hepatocellular carcinoma: results of an interim analysis. Int J Radiat Oncol Biol Phys 2016;95:477-82. [Crossref] [PubMed]
- Ohara K, Okumura T, Tsuji H, et al. Radiation tolerance of cirrhotic livers in relation to the preserved functional capacity: analysis of patients with hepatocellular carcinoma treated by focused proton beam radiotherapy. Int J Radiat Oncol Biol Phys 1997;38:367-72. [Crossref] [PubMed]
- Hata M, Tokuuye K, Sugahara S, et al. Proton beam therapy for hepatocellular carcinoma patients with severe cirrhosis. Strahlenther Onkol 2006;182:713-20. [Crossref] [PubMed]
- Hata M, Tokuuye K, Sugahara S, et al. Proton beam therapy for hepatocellular carcinoma with portal vein tumor thrombus. Cancer 2005;104:794-801. [Crossref] [PubMed]
- Sugahara S, Nakayama H, Fukuda K, et al. Proton-beam therapy for hepatocellular carcinoma associated with povein tumor thrombosis. Strahlenther Onkol 2009;185:782-8. [Crossref] [PubMed]
- Mizumoto M, Tokuuye K, Sugahara S, et al. Proton beam therapy for hepatocellular carcinoma adjacent to the porta hepatis. Int J Radiat Oncol Biol Phys 2008;71:462-7. [Crossref] [PubMed]
- Hata M, Tokuuye K, Sugahara S, et al. Proton beam therapy for hepatocellular carcinoma with limited treatment options. Cancer 2006;107:591-8. [Crossref] [PubMed]
- Hata M, Tokuuye K, Sugahara S, et al. Proton beam therapy for aged patients with hepatocellular carcinoma. Int J Radiat Oncol Biol Phys 2007;69:805-12. [Crossref] [PubMed]
- Nakayama H, Sugahara S, Fukuda K, et al. Proton beam therapy for hepatocellular carcinoma located adjacent to the alimentary tract. Int J Radiat Oncol Biol Phys 2011;80:992-5. [Crossref] [PubMed]
- Sugahara S, Oshiro Y, Nakayama H, et al. Proton beam therapy for large hepatocellular carcinoma. Int J Radiat Oncol Biol Phys 2010;76:460-6. [Crossref] [PubMed]
- Fukumitsu N, Sugahara S, Nakayama H, et al. A prospective study of hypofractionated proton beam therapy for patients with hepatocellular carcinoma. Int J Radiat Oncol Biol Phys 2009;74:831-6. [Crossref] [PubMed]
- Mizumoto M, Okumura T, Hashimoto T, et al. Proton beam therapy for hepatocellular carcinoma: a comparison of three treatment protocols. Int J Radiat Oncol Biol Phys 2011;81:1039-45. [Crossref] [PubMed]
- Berman AT, Both S, Sharkoski T, et al. Proton reirradiation of recurrent rectal cancer: dosimetric comparison, toxicities, and preliminary outcomes. Int J Particle Ther 2014;1:2-13. [Crossref]
- Vitek P, Kubes J, Ondrova B, et al. Hypofractionated proton reirradiation of pelvic and retroperitoneal malignancies. Presented at Particle Therapy Co-Operative Group North America 2nd Annual Meeting. San Diego, California, 18-23 May 2015.
- Ie M, Yamaguchi T, Kinugasa Y, et al. Complete response of locally recurrent anorectal cancer to proton beam therapy alone--a case report. Gan To Kagaku Ryoho 2014;41:2623-5. [PubMed]
- Hashimoto T, Okumura T, Kanemoto A, et al. Proton beam therapy for metastatic liver cancer. Int J Radiat Oncol Biol Phys 2011;81:S353. [Crossref]
- Kang JI Jr, Slater JD, Grove R, et al. Early progress on a phase I study of stereotactic body proton therapy (SBPT) for liver metastases. Presented at Particle Therapy Co-Operative Group North America 1st Annual Meeting. Houston, Texas, 27-29 October 2014.
- Fukumitsu N, Okumura T, Numajiri H, et al. Proton beam therapy for metastatic liver tumors. Presented at Particle Therapy Co-Operative Group North America 2nd Annual Meeting. San Diego, California, 18-23 May 2015.
- Katsui K, Terashima K, Takagi M, et al. Clinical results of particle radiotherapy using protons or carbon ions liver oligometastases. Presented at Particle Therapy Co-Operative Group North America 2nd Annual Meeting. San Diego, California, 18-23 May 2015.
- Yoon SS, Chen YL, Kirsch DG, et al. Proton-beam, intensity-modulated, and/or intraoperative electron radiation therapy combined with aggressive anterior surgical resection for retroperitoneal sarcomas. Ann Surg Oncol 2010;17:1515-29. [Crossref] [PubMed]
- Schneider RA, Vitolo V, Albertini F, et al. Small bowel toxicity after high dose spot scanning-based proton beam therapy for paraspinal/retroperitoneal neoplasms. Strahlenther Onkol 2013;189:1020-5. [Crossref] [PubMed]
- Welsh J, Gomez D, Palmer MB, et al. Intensity-modulated proton therapy further reduces normal tissue exposure during definitive therapy for locally advanced distal esophageal tumors: a dosimetric study. Int J Radiat Oncol Biol Phys 2011;81:1336-42. [Crossref] [PubMed]
- Dionisi F, Avery S, Lukens JN, et al. Proton therapy in adjuvant treatment of gastric cancer: planning comparison with advanced x-ray therapy and feasibility report. Acta Oncol 2014;53:1312-20. [Crossref] [PubMed]
- Ding X, Dionisi F, Tang S, et al. A comprehensive dosimetric study of pancreatic cancer treatment using three-dimensional conformal radiation therapy (3DCRT), intensity-modulated radiation therapy (IMRT), volumetric-modulated radiation therapy (VMAT), and passive-scattering and modulated-scanning proton therapy (PT). Med Dosim 2014;39:139-45. [Crossref] [PubMed]
- Abboud MT, Crane C, Javle M, et al. Risk factors for gastrointestinal bleeding in patients treated with radiation therapy for intrahepatic cholangiocarcinoma. Int J Radiat Oncol Biol Phys 2011;81:S354. [Crossref]
- Wolff HA, Wagner DM, Conradi LC, et al. Irradiation with protons for the individualized treatment of patients with locally advanced rectal cancer: a planning study with clinical implications. Radiother Oncol 2012;102:30-7. [Crossref] [PubMed]
- Ojerholm E, Kirk ML, Thompson RF, et al. Pencil-beam scanning proton therapy for anal cancer: a dosimetric comparison with intensity-modulated radiotherapy. Acta Oncol 2015;54:1209-17. [Crossref] [PubMed]
- Scorsetti M, Mancosu P, Navarria P, et al. Stereotactic body radiation therapy (SBRT) for adrenal metastases: a feasibility study of advanced techniques with modulated photons and protons. Strahlenther Onkol 2011;187:238-44. [Crossref] [PubMed]
- Swanson EL, Indelicato DJ, Louis D, et al. Comparison of three-dimensional (3D) conformal proton radiotherapy (RT), 3D conformal photon RT, and intensity-modulated RT for retroperitoneal and intra-abdominal sarcomas. Int J Radiat Oncol Biol Phys 2012;83:1549-57. [Crossref] [PubMed]
- Wang J, Palmer M, Bilton SD, et al. Comparing proton beam to intensity modulated radiation therapy planning in esophageal cancer. Int J Particle Ther 2015;1:866-77. [Crossref]
- Coen JJ, Bae K, Zietman AL, et al. Acute and late toxicity after dose escalation to 82 GyE using conformal proton radiation for localized prostate cancer: initial report of American College of Radiology Phase II study 03-12. Int J Radiat Oncol Biol Phys 2011;81:1005-9. [Crossref] [PubMed]
- Simone CB 2nd, Rengan R. The use of proton therapy in the treatment of lung cancers. Cancer J 2014;20:427-32. [Crossref] [PubMed]
- Nguyen F, Rubino C, Guerin S, et al. Risk of a second malignant neoplasm after cancer in childhood treated with radiotherapy: correlation with the integral dose restricted to the irradiated fields. Int J Radiat Oncol Biol Phys 2008;70:908-15. [Crossref] [PubMed]
- van Hagen P, Hulshof MC, van Lanschot JJ, et al. Preoperative chemoradiotherapy for esophageal or junctional cancer. N Engl J Med 2012;366:2074-84. [Crossref] [PubMed]
- Tepper J, Krasna MJ, Niedzwiecki D, et al. Phase III trial of trimodality therapy with cisplatin, fluorouracil, radiotherapy, and surgery compared with surgery alone for esophageal cancer: CALGB 9781. J Clin Oncol 2008;26:1086-92. [Crossref] [PubMed]
- Chuong MD, Hallemeier CL, Jabbour SK, et al. Improving Outcomes for Esophageal Cancer using Proton Beam Therapy. Int J Radiat Oncol Biol Phys 2016;95:488-97. [Crossref] [PubMed]
- Verma V, Mishra MV, Mehta MP. A systematic review of the cost and cost-effectiveness studies of proton radiotherapy. Cancer 2016;122:1483-501. [Crossref] [PubMed]
- Dionisi F, Amichetti M. Proton therapy results in the treatment of hepatocellular carcinoma according to the Barcelona-Clinic liver cancer staging system. Int J Med Phys Clin Eng Radiat Oncol 2015;4:96-103. [Crossref]
- Huang PI, Chao Y, Li CP, et al. Efficacy and factors affecting outcome of gemcitabine concurrent chemoradiotherapy in patients with locally advanced pancreatic cancer. Int J Radiat Oncol Biol Phys 2009;73:159-65. [Crossref] [PubMed]
- Verma V, Li J, Lin C. Neoadjuvant Therapy for Pancreatic Cancer: Systematic Review of Postoperative Morbidity, Mortality, and Complications. Am J Clin Oncol 2016;39:302-13. [Crossref] [PubMed]
- Paryani NN, Zlotecki RA, Swanson EL, et al. Multimodality local therapy for retroperitoneal sarcoma. Int J Radiat Oncol Biol Phys 2012;82:1128-34. [Crossref] [PubMed]
- Bouchard M, Amos RA, Briere TM, et al. Dose escalation with proton or photon radiation treatment for pancreatic cancer. Radiother Oncol 2009;92:238-43. [Crossref] [PubMed]
- Plastaras JP, Ainsley CG, Vapiwala N, et al. Effect of beam arrangement on dosimetric parameters using scattered beam proton radiotherapy (PRT) for esophageal cancer. Int J Radiat Oncol Biol Phys 2008;72:S545. [Crossref]
- Yu J, Zhang X, Liao L, et al. Motion guided intensity modulated proton therapy for distal esophageal cancer. Presented at Particle Therapy Co-Operative Group North America 2nd Annual Meeting. San Diego, California, 18-23 May 2015.
- Chuong MD, Yam M, Li Z, et al. Is pencil beam scanning dosimetrically advantageous compared to passively scattered proton therapy for unresectable pancreatic cancer? Presented at Particle Therapy Co-Operative Group North America 2nd Annual Meeting. San Diego, California, 18-23 May 2015.