Detection and therapeutic implications of homologous recombination repair deficiency in pancreatic cancer: a narrative review
Introduction
Pancreas ductal adenocarcinoma (PDAC) remains one of the most lethal cancers worldwide and is projected to become the second most common cause of cancer death in the USA by 2030 (1). The carcinogenesis of PDAC follows a defined series of pathogenic alterations from normal mucosa to specific precursor lesions and ultimately invasive malignancy (2). It is characterized by early and rapid dissemination outside of the pancreas (3). Furthermore, most patients do not have early symptoms and there is no early detection test available. These factors usually result in a late stage of presentation. The mainstay of treatment for those with advanced disease is chemotherapy but the benefit of chemotherapy is small for most patients. There are currently no validated biomarkers for treatment selection for the majority of patients with PDAC. Less than 2% of patients have targetable genetic alterations such as microsatellite-instability-high (MSI-H), high tumor mutational burden (TMB) or NTRK fusions. Despite intensive investigations, additional biomarkers to guide therapy remain elusive.
Genomic sequencing studies of PDAC tumors show that up to 15% (4) of tumors harbor defects that lead to genomic instability due to deficient DNA repair (5). DNA repair pathways are critically important in protecting cells from exogenous and endogenous DNA damage. These pathways are frequently dysfunctional in cancer cells, leading to the accumulation of DNA damage and genomic instability (6). Homologous recombination deficiency (HRD) is a complex and dynamic tumor phenotype characterized by the inability to repair double-strand breaks (DSBs) in DNA via homologous recombination. Another highly conserved DNA repair process is the base excision repair pathway involving single-strand DNA breaks. Poly (ADP-ribose) polymerase (PARP) enzymes are key elements in this pathway. Approximately 5–8% of PDAC’s arise in association with pathogenic germline alterations in BRCA1/2 leading to deficiency in BRCA function and thus more dependence on PARP for DNA repair; these patients can benefit from maintenance therapy with PARP-inhibitors if they have a response to frontline therapy with a platinum-containing chemotherapy (7). Herein, we present this review of HRD in PDAC in accordance with the Narrative Review reporting checklist (available at https://jgo.amegroups.com/article/view/10.21037/jgo-23-85/rc).
Methods
In this study, the PubMed and Google Scholar databases were searched, and the retrieval time was limited from inception to Feb 2023, ensuring the retrieving articles are up to date. In addition, other methods, such as website and citation searches, were employed to retrieve the relevant articles. The key words used included “HRD”, “PDAC”, “BRCA”, and “PARP”. The initial search strategy was not limited to PDAC since other cancer types, such as breast and ovarian, may also have relevant HRD information. Conference abstracts and other literature which fulfilled the above criteria were included, and non-English language publications were excluded. Note that only pathogenic variants in BRCA1/2 and other HRD genes were included, and variants of uncertain significance were excluded. Both authors extracted the following data from each included study: name of first author, year of publication, study design, sample size, intervention/exposure, outcomes, covariates, statistical approaches, and main findings. Titles and abstracts of all identified articles and publicly available data sets were independently screened by both authors and each selected manuscript was double-checked by the other. No discrepancies in data extraction were found between the two authors. Table 1 summarizes the search strategy.
Table 1
Items | Specification |
---|---|
Date of search | 10/20/2022–5/22/2023 |
Databases and other sources searched | PubMed, Google Scholar, website and citation searches |
Search terms used | “HRD”, “PDAC”, “BRCA”, and “PARP” |
Timeframe | From inception to Feb 2023 |
Inclusion and exclusion criteria | Only pathogenic variants in BRCA1/2 and other HRD genes were included |
Conference abstracts and other grey literature that fulfilled criteria were included | |
Variants of uncertain significance were excluded | |
Reviews, letters, case reports, and publications not in English were excluded | |
Selection process | Both authors performed data extraction. Titles and abstracts of all identified articles and publicly available data sets were independently screened by two authors and each selected article was double-checked by the other. No discrepancies in data extraction were found between the two authors |
HRD, homologous recombination deficiency.
Frequency and implications of HRD
One of the key biological determinants of HRD are core pathogenic mutations in specific genes associated with double-strand DNA repair via HR; germline alterations in these genes (such as BRCA1/2, PALB2, RAD51C/D) occur in 3–10% (8) and somatic alteration in 15–17% (5,9). As illustrated in Figure 1, such alterations are associated with a characteristic genomic landscape in addition to an inflamed tumor microenvironment (TME), which in turn sensitizes to platinum-based chemotherapy and PARP-inhibitors, and potentially new treatment strategies including immunotherapy and other treatments targeting DNA-damage repair.
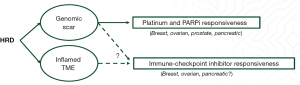
Among all the genes associated with HRD, the two most important are BRCA1 and BRCA2, which were cloned in 1994 (10) and 1995 (11) respectively. It is well established that these genes are strongly associated with hereditary breast and ovarian cancers. Hereditary BRCA1/BRCA2 mutations account for as high as 50% of all unselected breast cancers with a strong family history (6). Approximately 1 in 2 advanced ovarian cancer patients also have an HRD-positive tumor with a BRCA-like genomic scar even if BRCA1/2 do not harbor germline mutations (12). Pathogenic germline BRCA1/2 variants result in a distinct imprint on the PDAC genome (the “genomic scar”) characterized by increased genomic instability in these tumors. A pan-cancer analysis utilizing the Foundation Medicine database (including ~11,000 PDAC cases) revealed a median TMB of 1.7 muts/Mb in BRCA1/2 wild-type PDAC tumors, versus 3.5 muts/Mb with BRCA1/2 mono-allelic loss and 4.3 muts/Mb with bi-allelic loss (P<0.001) (13). Another marker of genomic instability, genomic loss of heterozygosity (gLOH-high), was significantly higher in PDAC tumors with bi-allelic loss of BRCA1/2 versus BRCA1/2 wild-type tumors (75% vs. 14%, P<0.001). Furthermore, BRCA mutations are associated with a higher rate of tumor programmed death-ligand 1 (PD-L1) positivity in another report using a different somatic profiling database from Caris Life Sciences (including ~2,800 PDAC cases); the PD-L1 combined positive score (CPS) was greater than 1 in 22% of BRCA-mutant PDAC tumors versus 11% in wild-type tumors (P<0.001) (14). In the Foundation Medicine pan-cancer analysis, similar results were seen in other tumor types harboring BRCA1/2 mutations. Tables 2,3 summarizes the main findings.
Table 2
Marker | BRCA wild-type, N=11,556 | BRCA mono-allelic loss, N=33 | BRCA bi-allelic loss, N=166 | P |
---|---|---|---|---|
TMB | 1.7 | 3.5 | 4.3 | <0.001 |
gLOH-high | 14.4% | 13.6% | 75% | <0.001 |
TMB, tumor mutational burden; gLOH, genomic loss of heterozygosity.
Table 3
Marker | BRCA-wild type, N=2,694 | BRCA-mutant, N=124 | P |
---|---|---|---|
MSI-H | 1.2% | 4.8% | 0.002 |
TMB | 6.5 | 8.7 | <0.001 |
PD-L1 CPS >1% | 11.2% | 21.8% | <0.001 |
MSI-H, microsatellite-instability-high; TMB, tumor mutational burden; PD-L1, programmed death-ligand 1; CPS, combined positive score.
The effects of germline BRCA1/2 alterations appear to be similar in other tumors in the hereditary breast/ovarian cancer (HBOC) syndrome. BRCA1-deficient breast cancers are associated with increased expression of PD-L1 and PD-1, higher abundance of tumor-infiltrating immune cells, and enrichment of T cell-inflamed signature (15). In prostate cancer, intra- and extra-tumoral immune cell infiltration is significantly higher in BRCA-mutant versus wild-type tumors (16).
The PDAC TME is characteristically tumor-permissive and immune-suppressive (3). However, the genomic scar that results from canonical HRD alterations such as BRCA appears to lead to higher genomic instability which in turn results in increased neoantigen expression. This reshapes the tumor microenvironment and has the potential to restore the antitumor immune response. A recent study by Samstein et al. demonstrated that mutations in BRCA1 and BRCA2 result in distinct mutational landscapes and differentially modulate the tumor-immune microenvironment (17). Gene expression programs related to both adaptive and innate immunity are found to be enriched specifically in BRCA2-deficient tumors. Moreover, the study also indicated that the response to immune checkpoint blockade (ICB) varies depending on whether the mutation is in BRCA1 or BRCA2, with truncating mutations in BRCA2 associated with a more favorable response compared to BRCA1 mutations. We will describe this in more detail in the treatment section below.
Taken together, these different lines of evidence all point to a different genomic and TME landscape in PDAC tumors associated with HRD, opening new opportunities for innovative strategies to target this subgroup.
HRD detection
Various academic and commercial next-generation sequencing (NGS) platforms have attempted to refine the process of classifying a tumor as HR-deficient beyond simply the presence of single-gene alterations.
DNA-single gene approach
The HRDetect is a weighted NGS model which focuses on the detection of two most studied genes BRCA1/BRCA2 and defines deficiency in terms of single nucleotide variants (SNVs) (18), structural variants (SVs) (19), and genomic instability patterns from both germline and somatic sequencing data (20). In this system, a supervised lasso logistic regression model was used to identify six critically distinguishing mutational signatures. HRDetect scores were then computed by aggregating six mutation signatures associated with HRD, which were then normalized and transformed. It was reported that this model could identify BRCA1/BRCA2 deficient breast cancer with 98.7% sensitivity (AUC 0.98) (20). This model was later validated independently and demonstrated evidence of its association with platinum response in advanced breast cancer (21). However, the model does not show high association with other less common HR repair genes such as RAD50, PALB2 and ATR.
DNA panel with genomic scar approach
Another popular strategy is to use DNA-based measures of genomic instability. Cells with HRD frequently display characteristics of genomic instability such as loss of heterozygosity (LOH), telomeric allelic imbalance (TAI), and large-scale state transition (LST). One such HRD score (22) is calculated based on unweighted summing of these three measures of genomic instability—HRD (defined as HRD score >42 and/or BRCA1 or BRCA2 mutation). This was evaluated as a predictive biomarker for neoadjuvant platinum-based therapy in two different breast cancer clinical cohorts. These HRD scores showed strong correlation with BRCA1/2 deficiency regardless of breast cancer subtype (23). A limitation of these techniques is that these genomic alterations that are detected at baseline can persist despite the development of resistance to platinum chemotherapy/PARPi and thus are not a dynamic marker of HRD (24).
DNA and RNA combined approaches
DNA-based NGS for the definition of the HRD phenotype are time-dependent given that multiple genomic hits are needed to reach the threshold for detection. In contrast to exomic sequencing approaches, transcriptomic profiling can uncover the dynamic state of HRD independently from the genomic scar which may be present at the time of diagnosis. The Tempus HRD platform (25) provides a composite score based on two modalities; HRD-DNA, which is a readout of genome-wide LOH (gwLOH) to predict HRD status (mainly for breast and ovarian cancers), and HRD-RNA, a logistic regression model trained on whole-exome capture RNA sequencing data which predicts HRD status across all other solid tumors to detect HRD driven by BRCA1/2 loss and non-BRCA1/2 mechanisms. This model has shown promising result of predicting HRD status across all types of solid tumors, with the best performance being in breast and ovarian cancer samples (26).
Commercially available HRD diagnostic assays
Multiple commercially available assays have incorporated the methodologies discussed above. These composite markers or scores, focusing on multi-omic approaches combining computational biology and artificial intelligence, can potentially improve detection accuracy and robustness. Table 4 (25,27-29) lists some of the assays available in the market. This list of assays is not exhaustive, and not all these tests are FDA-approved companion diagnostics.
Table 4
Laboratory name | Test name | HRD status determined by | Minimum sample requirement | Turnaround time (days) |
---|---|---|---|---|
Caris (27) | Molecular Intelligence Comprehensive Tumor Profiling | BRCA somatic mutation and LOH | FFPE tissue (block or 10 unstained slides; 4–6 needle biopsies acceptable) | 10–14 |
Foundation Medicine (28) | FoundationOne CDx | BRCA somatic mutation positive and/or LOH high | FFPE tissue (block + 1 H&E slide or 10 unstained slides + 1 H&E slide) | 12 or less |
Myriad (29) | myChoice CDx | BRCA germline and somatic mutation positive and/or positive HRD score (composite of LOH, TAI, and LST) | FFPE tissue (block or 8–20 unstained slides + 1 H&E slide | 14 or less |
Tempus (25) | Tempus xT HRD test | BRCA somatic mutation and/or LOH high Transcriptomic (RNA) data | Blood (8 mL), saliva, FFPE, tissue (block + 1 H&E slide or 10 unstained slides + 1 H&E slide) | 9–14 |
HRD, homologous recombination deficiency; LOH, loss of heterozygosity; FFPE, formalin-fixed paraffin-embedded; H&E, hematoxylin and eosin; TAI, telomeric allelic imbalance; LST, large-scale state transition.
HRD detection status in PDAC
The aforementioned HRD signatures have been developed using available databases of tumors that have undergone NGS. However, the BRCA-mediated genotype may produce different phenotypes and effects depending on the tumor primary site; the performance of these genome signatures in ovarian cancer and breast cancer may not be relevant to PDAC (26,30). Moreover, the reliability of NGS is critically dependent on the quality of the tumor sample; in general, pancreas biopsies (or even metastatic site biopsies in patients with PDAC) have less tumor cellularity (also referred to as “purity”) than resection specimens from breast or ovarian cancer. Furthermore, there are substantially fewer PDAC samples in most NGS databases than breast or ovarian which also limits the development of a reproducible HRD signature.
In an attempt to determine the best HRD classifier in PDAC, Golan et al. (31) analyzed 391 human PDAC samples and multiple HRD classifiers were applied including (I) the genomic instability score (GIS) from the Myriad MyChoice HRD assay; (II) substitution base signature 3 (SBS3); (III) HRDetect; and (IV) structural variant (SV) burden. Clinical outcomes such as survival and response to chemotherapy were also used to correlate with HRD status (i.e., platinum and PARP sensitivity as a surrogate for high HRD). The HRDetect (score >0.7) predicted gBRCA1/PALB2 deficiency with highest sensitivity (98%) and specificity (100%). At the same time, HRD genomic tumor classifiers suggested that features of HRD could be detected 7% to 10% of PDACs that do not carry gBRCA/PALB2. As for the clinical outcomes, HRD status was not necessarily prognostic in resected PDAC. However in advanced PDAC the GIS (P=0.02), SBS3 (P=0.03), and HRDetect score (P=0.005) were associated with platinum response and longer survival (31). There remains the need for prospective validation of this type of signature.
Treatment for HRD PDAC
The currently available clinical data guiding the treatment of PDAC patients with HRD are showing in Table 5 (4,7,32-41).
Table 5
Therapy category | Approach | Result | P |
---|---|---|---|
Platinum-based | Actionable mutations (mostly HRD) matched therapy vs. non matched therapy (4,32,33) | Median OS: 2.58 vs. 1.51 years | 0.0004 |
Nab-paclitaxel + gemcitabine + cisplatin in metastatic PDAC (34) | ORR: 71%, DCR 88% | N/A | |
First-line platinum in PDAC patients with or without HRD (35) | Median OS: 25.1 vs. 15.3 months | <0.01 | |
FOLFIRINOX in PDAC patients with or without DDR gene alterations (36) | Median PFS: 18.5 vs. 7.4 months | 0.005 | |
Gemcitabine + cisplatin with or without veliparib (37) | Median OS: 15.5 vs. 16.4 months | 0.6 | |
PARP-I’s | Maintenance olaparib vs. placebo (7) | PFS: 7.4 vs. 3.8 months | 0.004 |
Maintenance rucaparib (38) | Median OS: 23.5 months | N/A | |
Maintenance olaparib in platinum-sensitive vs. platinum-resistant patients (39) | Median OS: 10.5 vs. 5.4 months | 0.03 | |
Combination ICI +/− PARP | Ipilimumab + nivolumab in PDAC patients with germline HRD mutations (40) | ORR: 42%, DCR: 58% | N/A |
Maintenance niraparib with nivolumab or ipilimumab (41) | Median OS: 13.2 vs. 17.3 months | N/A |
HRD, homologous recombination deficiency; PDAC, pancreatic ductal adenocarcinoma; OS, overall survival; DDR, DNA damage repair; PFS, progression-free survival; PARP-I, poly (ADP-ribose) polymerase inhibitor; N/A, not available; ICI, immune checkpoint inhibitor; ORR, objective response rate; DCR, disease control rate.
Platinum-based therapy
Multiple data sets consistently show benefit for PDAC patients with HRD treated with platinum-based chemotherapy combinations. In the retrospective analysis from PanCan Know Your Tumor registry trial (4,32,33), PDAC patients who had actionable molecular alterations derived significant benefit from receiving a genomically-matched therapy. The median overall survival of patients who had actionable alterations receiving matched therapy was approximately 1 year longer than those with actionable alterations receiving unmatched therapy, or those without actionable alterations; the most common set of alterations in this study was in the HRD family of mutations (33). Other retrospective series (34,36) and prospective trials (37) have confirmed the same finding, and the use of platinum chemotherapy in these patients is now accepted as a standard of care.
PARP inhibitors
Maintenance therapy with Olaparib after at least 4 months of frontline platinum-based chemotherapy in patients with germline BRCA1/2 mutations in PDAC is now an established standard, but PARP-inhibitor resistance remains a challenge. Trials are underway to build on the results of the POLO trial including evaluating the combination of PARP inhibitor therapy and immunotherapy (42). A critical unmet need remains the development of strategies to either induce HRD or overcome innate or acquired resistance to HRD-targeted agents and thus expand the number of patients who may benefit from these therapies. In the POLO trial (7), maintenance with Olaparib resulted in a significant improvement in progression- free survival for patients with metastatic PDAC a germline BRCA mutation who had not progressed during platinum-based chemotherapy. This trial led to the approval of Olaparib in the USA in this setting. Recent prospective trials have expanded the population eligible for PARP inhibitors in PDAC to include patients with somatic BRCA2 (38) mutations as well as germline PALB2 mutations (39).
Immune checkpoint inhibitors (ICIs)
Despite the breakthroughs seen with ICIs in many malignancies, they have unfortunately been disappointing in unselected patients with PDAC (43). However, recent studies (40,41,44) have shown an association between germline HRD status in PDAC and response to ICIs, consolidating previous evidence of an association between BRCA1/2 variants in other tumors and immunotherapy response. In a recent report, Terrero et al. described a series of 12 patients with pathogenic germline variants in BRCA1/2 or RAD51C/D, a few of which achieved sustained complete responses to dual PD-1/CTLA-4 inhibition (40). The overall response rate was 42%, including 4 patients who achieved complete responses (all ongoing for 24 to 48 months) and another with a partial response. Additionally, Reiss et al. demonstrated superior effectiveness of ipilimumab/niraparib over nivolumab/niraparib for maintenance treatment of patients with metastatic PDAC after a response to first-line platinum therapy (41), underscoring the role of CTLA4 inhibition in this subgroup of PDAC patients. In the report from Terrero et al., archival tumor samples were subjected to transcriptomic analysis using the Nanostring platform (40). Tumors from responding patients had a significantly higher expression of the CCL4, CCL5 and CXCL10 than in non-responders. These co-regulated chemokines enable trafficking of immune effector populations into the TME and have been shown in a separate data set to be associated with a T-cell inflamed phenotype in PDAC (44). These clinical and translational data highlight the fact that this HRD subset may be an exception to the rule that PDAC generally does not benefit from ICI-based strategies.
Implications for future trial design and biomarker discovery
Targeting patients with PDAC whose tumors have HRD is still a relatively new area of investigation. Numerous clinical trials are currently underway aiming to make inroads into this subgroup. Table 6 (45-51) lists some ongoing phase 1 and phase 2 clinical trials that specifically target HRD-related cancers, including pancreatic cancer. The majority are investigating PARP-inhibitor or immunotherapy combinations.
Table 6
Trial identifier | Condition/disease | Intervention/treatment | Phase |
---|---|---|---|
NCT04150042 (45) The SHARON trial | Metastatic cancer with pathogenic BRCA1/2 or PALB2 germline variant | High-dose chemotherapy with autologous stem-cell rescue, combined with high-dose vitamin C | Phase 1 |
NCT04673448 (46) | Unresectable or metastatic breast, pancreatic, fallopian tube, ovarian or primary peritoneal cancer with pathogenic BRCA1/2 germline variant | Dostarlimab + niraparib | Phase 1 |
NCT04890613 (47) | Solid tumors with pathogenic germline BRCA2 and/or PALB2 variant | CX-5461 (RNA polymerase-1 inhibitor) | Phase 1 |
NCT04666740 (48) The POLAR trial | Metastatic PDAC with pathogenic germline or somatic HRD variant or response to frontline platinum-based therapy | Maintenance pembrolizumab + olaparib | Phase 2 |
NCT04858334 (49) The APOLLO trial | Resected PDAC with pathogenic germline BRCA1/2 or PALB2 variant | Adjuvant olaparib vs. placebo | Phase 2 |
NCT04548752 (50) The SWOG 2001 trial | Metastatic PDAC with pathogenic germline BRCA1/2 variants who have clinical benefit from frontline platinum-based therapy | Maintenance olaparib vs. olaparib + pembrolizumab | Phase 2 |
NCT05659914 (51) | Metastatic PDAC with pathogenic germline or somatic HRD variant who have clinical benefit from frontline platinum-based therapy | Maintenance olaparib + durvalumab | Phase 2 |
PDAC, pancreatic ductal adenocarcinoma; HRD, homologous recombination deficiency.
Given the absence of currently validated biomarkers for HRD PDAC apart from germline BRCA1/2, it is critical for ongoing and future trials to incorporate biospecimens for translational research. The gold-standard for defining HRD at this time could be a robust response to platinum-based therapy and these patients can be classified as HRD-positive. Genomic and/or transcriptomic signatures can then be correlated with the clinical phenotype to validate candidate biomarker signatures. Another promising area of investigation is the use of ctDNA to detect HRD. Although this approach is not yet validated, it is a potentially simpler method to determine HRD status and monitor the evolution of the HRD state over time.
Limitations
Our review has several limitations that should be taken into consideration. Firstly, the retrospective nature of data collection introduces biases, such as ascertainment bias, and inherent publication bias, where the available research on the topic may be skewed towards publishing only significant results, potentially leading to an incomplete picture of the overall evidence. Secondly, there may be baseline heterogeneity or unclear data on patient characteristics across different studies, such as geographic location, ethnicity, disease stage or patient age, which can affect the generalizability and applicability of the findings. Thirdly, there is variability in sequencing methodologies, which may introduce inconsistencies and impact the comparability of the results. Fourthly, the timing of tumor next-generation sequencing in relation to treatment potentially varies across studies. Finally, most of the studies that have looked at the impact of HRD alterations have been limited by small sample sizes and the process of validating genomic or transcriptomic biomarkers typically requires a large number of samples to improve the reliability.
Conclusions
In summary, to consistently detect clinically significant HRD status has proven to be challenging in general. In this review, we focused on the recent research work specifically targeting on developing reliable biomarkers to identify HRD positive PDAC patients who may benefit from exploiting HRD. Germline BRCA1 and BRCA2 loss are the most common HRD mutations and have helped to provide insight into other mechanisms of HRD as well. Currently, available HRD assays focus on a static measurement of the genotype scar, and these assays are typically done on specimens obtained at the time of diagnosis when patients are treatment-naive. However, BRCA1/2-deficient tumor cells may restore BRCA function over time, and current HRD assays are limited in providing real-time assessment of genomic instability of PDAC. Ultimately, whole-genome sequencing may provide almost all the necessary information that each of the discussed assays is intended to measure. However, until WGS becomes reasonably cost-effective, alternatives need to be found. Several novel HRD-targeted therapies for PDAC are currently being investigated in clinical trials, offering opportunities to prospectively develop and validate innovative HRD biomarkers to better select patients who will benefit most from these therapies.
Acknowledgments
Funding: This work was supported by The Helene & Bernard Herskowitz Fund for Pancreatic Cancer Research, The Esther Finkelstein Family Fund for Pancreatic Cancer Research, The Luis Rios Family Fund for Pancreatic Cancer Research, and the Gardner fund for Pancreatic Cancer Research to P. Hosein. Research reported in this publication was also supported by the NCI/NIH Award P30CA240139.
Footnote
Reporting Checklist: The authors have completed the Narrative Review reporting checklist. Available at https://jgo.amegroups.com/article/view/10.21037/jgo-23-85/rc
Peer Review File: Available at https://jgo.amegroups.com/article/view/10.21037/jgo-23-85/prf
Conflicts of Interest: Both authors have completed the ICMJE uniform disclosure form (available at https://jgo.amegroups.com/article/view/10.21037/jgo-23-85/coif). The authors have no conflicts of interest to declare.
Ethical Statement: The authors are accountable for all aspects of the work in ensuring that questions related to the accuracy or integrity of any part of the work are appropriately investigated and resolved.
Open Access Statement: This is an Open Access article distributed in accordance with the Creative Commons Attribution-NonCommercial-NoDerivs 4.0 International License (CC BY-NC-ND 4.0), which permits the non-commercial replication and distribution of the article with the strict proviso that no changes or edits are made and the original work is properly cited (including links to both the formal publication through the relevant DOI and the license). See: https://creativecommons.org/licenses/by-nc-nd/4.0/.
References
- Siegel RL, Miller KD, Wagle NS, et al. Cancer statistics, 2023. CA Cancer J Clin 2023;73:17-48. [Crossref] [PubMed]
- Mohammed S, Van Buren G 2nd, Fisher WE. Pancreatic cancer: advances in treatment. World J Gastroenterol 2014;20:9354-60. [PubMed]
- Robatel S, Schenk M. Current Limitations and Novel Perspectives in Pancreatic Cancer Treatment. Cancers (Basel) 2022;14:985. [Crossref] [PubMed]
- Pishvaian MJ, Bender RJ, Halverson D, et al. Molecular Profiling of Patients with Pancreatic Cancer: Initial Results from the Know Your Tumor Initiative. Clin Cancer Res 2018;24:5018-27. [Crossref] [PubMed]
- Waddell N, Pajic M, Patch AM, et al. Whole genomes redefine the mutational landscape of pancreatic cancer. Nature 2015;518:495-501. [Crossref] [PubMed]
- den Brok WD, Schrader KA, Sun S, et al. Homologous Recombination Deficiency in Breast Cancer: A Clinical Review. JCO Precis Oncol 2017;1:1-13. [Crossref] [PubMed]
- Golan T, Hammel P, Reni M, et al. Maintenance Olaparib for Germline BRCA-Mutated Metastatic Pancreatic Cancer. N Engl J Med 2019;381:317-27. [Crossref] [PubMed]
- Holter S, Borgida A, Dodd A, et al. Germline BRCA Mutations in a Large Clinic-Based Cohort of Patients With Pancreatic Adenocarcinoma. J Clin Oncol 2015;33:3124-9. [Crossref] [PubMed]
- Bailey P, Chang DK, Nones K, et al. Genomic analyses identify molecular subtypes of pancreatic cancer. Nature 2016;531:47-52. [Crossref] [PubMed]
- Friedman LS, Ostermeyer EA, Szabo CI, et al. Confirmation of BRCA1 by analysis of germline mutations linked to breast and ovarian cancer in ten families. Nat Genet 1994;8:399-404. [Crossref] [PubMed]
- Wooster R, Bignell G, Lancaster J, et al. Identification of the breast cancer susceptibility gene BRCA2. Nature 1995;378:789-92. [Crossref] [PubMed]
- Frey MK, Pothuri B. Homologous recombination deficiency (HRD) testing in ovarian cancer clinical practice: a review of the literature. Gynecol Oncol Res Pract 2017;4:4. [Crossref] [PubMed]
- Sokol ES, Pavlick D, Khiabanian H, et al. Pan-Cancer Analysis of BRCA1 and BRCA2 Genomic Alterations and Their Association With Genomic Instability as Measured by Genome-Wide Loss of Heterozygosity. JCO Precis Oncol 2020;4:442-65. [Crossref] [PubMed]
- Seeber A, Zimmer K, Kocher F, et al. Molecular characteristics of BRCA1/2 and PALB2 mutations in pancreatic ductal adenocarcinoma. ESMO Open 2020;5:e000942. [Crossref] [PubMed]
- Wen WX, Leong CO. Association of BRCA1- and BRCA2-deficiency with mutation burden, expression of PD-L1/PD-1, immune infiltrates, and T cell-inflamed signature in breast cancer. PLoS One 2019;14:e0215381. [Crossref] [PubMed]
- Jenzer M, Keß P, Nientiedt C, et al. The BRCA2 mutation status shapes the immune phenotype of prostate cancer. Cancer Immunol Immunother 2019;68:1621-33. [Crossref] [PubMed]
- Samstein RM, Krishna C, Ma X, et al. Mutations in BRCA1 and BRCA2 differentially affect the tumor microenvironment and response to checkpoint blockade immunotherapy. Nat Cancer 2021;1:1188-203. [Crossref] [PubMed]
- Alexandrov LB, Nik-Zainal S, Wedge DC, et al. Signatures of mutational processes in human cancer. Nature 2013;500:415-21. [Crossref] [PubMed]
- Nik-Zainal S, Davies H, Staaf J, et al. Landscape of somatic mutations in 560 breast cancer whole-genome sequences. Nature 2016;534:47-54. [Crossref] [PubMed]
- Davies H, Glodzik D, Morganella S, et al. HRDetect is a predictor of BRCA1 and BRCA2 deficiency based on mutational signatures. Nat Med 2017;23:517-25. [Crossref] [PubMed]
- Zhao EY, Shen Y, Pleasance E, et al. Homologous Recombination Deficiency and Platinum-Based Therapy Outcomes in Advanced Breast Cancer. Clin Cancer Res 2017;23:7521-30. [Crossref] [PubMed]
- Telli ML, Timms KM, Reid J, et al. Homologous Recombination Deficiency (HRD) Score Predicts Response to Platinum-Containing Neoadjuvant Chemotherapy in Patients with Triple-Negative Breast Cancer. Clin Cancer Res 2016;22:3764-73. [Crossref] [PubMed]
- Timms KM, Abkevich V, Hughes E, et al. Association of BRCA1/2 defects with genomic scores predictive of DNA damage repair deficiency among breast cancer subtypes. Breast Cancer Res 2014;16:475. [Crossref] [PubMed]
- Wang Y, Park JYP, Pacis A, et al. A Preclinical Trial and Molecularly Annotated Patient Cohort Identify Predictive Biomarkers in Homologous Recombination-deficient Pancreatic Cancer. Clin Cancer Res 2020;26:5462-76. [Crossref] [PubMed]
- Tempus. The Tempus HRD Test. [cited 2023 1/28]; Available online: www.tempus.com/wp-content/uploads/2020/05/Tempus-Tech-Spotlight-The-Tempus-HRD-Test.pdf.
- Leibowitz BD, Dougherty BV, Bell JSK, et al. Validation of genomic and transcriptomic models of homologous recombination deficiency in a real-world pan-cancer cohort. BMC Cancer 2022;22:587. [Crossref] [PubMed]
- Caris. Comprehensive Molecular Profiling. [cited 2023 1/28]; Available online: www.carislifesciences.com/products-and-services/molecular-profiling/.
- FoundationMedicine. Our proven portfolio. That’s our foundation. . [cited 2023 1/28]; Available online: https://www.foundationmedicine.com/portfolio.
- Myriad. MYCHOICE® CDX MYRIAD HRD COMPANION DIAGNOSTIC TEST. [cited 2023 1/28]; Available online: https://myriad.com/genetic-tests/mychoicecdx-tumor-test/.
- Jonsson P, Bandlamudi C, Cheng ML, et al. Tumour lineage shapes BRCA-mediated phenotypes. Nature 2019;571:576-9. [Crossref] [PubMed]
- Golan T, O'Kane GM, Denroche RE, et al. Genomic Features and Classification of Homologous Recombination Deficient Pancreatic Ductal Adenocarcinoma. Gastroenterology 2021;160:2119-2132.e9. [Crossref] [PubMed]
- Pishvaian MJ, Blais EM, Brody JR, et al. Outcomes in Patients With Pancreatic Adenocarcinoma With Genetic Mutations in DNA Damage Response Pathways: Results From the Know Your Tumor Program. JCO Precis Oncol 2019;3:1-10. [Crossref] [PubMed]
- Pishvaian MJ, Blais EM, Brody JR, et al. Overall survival in patients with pancreatic cancer receiving matched therapies following molecular profiling: a retrospective analysis of the Know Your Tumor registry trial. Lancet Oncol 2020;21:508-18. [Crossref] [PubMed]
- Jameson GS, Borazanci E, Babiker HM, et al. Response Rate Following Albumin-Bound Paclitaxel Plus Gemcitabine Plus Cisplatin Treatment Among Patients With Advanced Pancreatic Cancer: A Phase 1b/2 Pilot Clinical Trial. JAMA Oncol 2019;6:125-32. [Crossref] [PubMed]
- Park W, Chen J, Chou JF, et al. Genomic Methods Identify Homologous Recombination Deficiency in Pancreas Adenocarcinoma and Optimize Treatment Selection. Clin Cancer Res 2020;26:3239-47. [Crossref] [PubMed]
- Palacio S, McMurry HS, Ali R, et al. DNA damage repair deficiency as a predictive biomarker for FOLFIRINOX efficacy in metastatic pancreatic cancer. J Gastrointest Oncol 2019;10:1133-9. [Crossref] [PubMed]
- O'Reilly EM, Lee JW, Zalupski M, et al. Randomized, Multicenter, Phase II Trial of Gemcitabine and Cisplatin With or Without Veliparib in Patients With Pancreas Adenocarcinoma and a Germline BRCA/PALB2 Mutation. J Clin Oncol 2020;38:1378-88. [Crossref] [PubMed]
- Reiss KA, Mick R, O'Hara MH, et al. Phase II Study of Maintenance Rucaparib in Patients With Platinum-Sensitive Advanced Pancreatic Cancer and a Pathogenic Germline or Somatic Variant in BRCA1, BRCA2, or PALB2. J Clin Oncol 2021;39:2497-505. [Crossref] [PubMed]
- Javle M, Shacham-Shmueli E, Xiao L, et al. Olaparib Monotherapy for Previously Treated Pancreatic Cancer With DNA Damage Repair Genetic Alterations Other Than Germline BRCA Variants: Findings From 2 Phase 2 Nonrandomized Clinical Trials. JAMA Oncol 2021;7:693-9. [Crossref] [PubMed]
- Terrero G, Datta J, Dennison J, et al. Ipilimumab/Nivolumab Therapy in Patients With Metastatic Pancreatic or Biliary Cancer With Homologous Recombination Deficiency Pathogenic Germline Variants. JAMA Oncol 2022;8:1-3. [Crossref] [PubMed]
- Reiss KA, Mick R, Teitelbaum U, et al. Niraparib plus nivolumab or niraparib plus ipilimumab in patients with platinum-sensitive advanced pancreatic cancer: a randomised, phase 1b/2 trial. Lancet Oncol 2022;23:1009-20. [Crossref] [PubMed]
- Crowley F, Park W, O'Reilly EM. Targeting DNA damage repair pathways in pancreas cancer. Cancer Metastasis Rev 2021;40:891-908. [Crossref] [PubMed]
- O'Reilly EM, Oh DY, Dhani N, et al. Durvalumab With or Without Tremelimumab for Patients With Metastatic Pancreatic Ductal Adenocarcinoma: A Phase 2 Randomized Clinical Trial. JAMA Oncol 2019;5:1431-8. [Crossref] [PubMed]
- Romero JM, Grünwald B, Jang GH, et al. A Four-Chemokine Signature Is Associated with a T-cell-Inflamed Phenotype in Primary and Metastatic Pancreatic Cancer. Clin Cancer Res 2020;26:1997-2010. [Crossref] [PubMed]
- NCT04150042. SHARON: A Clinical Trial for Metastatic Cancer With a BRCA or PALB2 Mutation Using Chemotherapy and Patients' Own Stem Cells. [cited 2023 5/20/2023]; Available online: https://clinicaltrials.gov/ct2/show/NCT04150042.
- NCT04673448. Niraparib and TSR-042 for the Treatment of BRCA-Mutated Unresectable or Metastatic Breast, Pancreas, Ovary, Fallopian Tube, or Primary Peritoneal Cancer. [cited 2023 5/20]; Available online: https://clinicaltrials.gov/ct2/show/NCT04673448.
- NCT04890613. Study of CX-5461 in Patients With Solid Tumours and BRCA1/2, PALB2 or Homologous Recombination Deficiency (HRD) Mutation. [cited 2023 5/20]; Available online: https://clinicaltrials.gov/ct2/show/NCT04890613.
- NCT04666740. A Study of Pembrolizumab and Olaparib for People With Metastatic Pancreatic Ductal Adenocarcinoma and Homologous Recombination Deficiency or Exceptional Treatment Response to Platinum-Based Therapy. [cited 2023 5/20]; Available online: https://clinicaltrials.gov/ct2/show/NCT04666740
- NCT04858334. APOLLO: A Randomized Phase II Double-Blind Study of Olaparib Versus Placebo Following Curative Intent Therapy in Patients With Resected Pancreatic Cancer and a Pathogenic BRCA1, BRCA2 or PALB2 Mutation. [cited 2023 5/20]; Available online: https://clinicaltrials.gov/ct2/show/NCT04858334.
- NCT04548752. Testing the Addition of Pembrolizumab, an Immunotherapy Cancer Drug to Olaparib Alone as Therapy for Patients With Pancreatic Cancer That Has Spread With Inherited BRCA Mutations. [cited 2023 5/20]; Available online: https://clinicaltrials.gov/ct2/show/NCT04548752.
- NCT05659914. Olaparib and Durvalumab (MEDI4736) in Patients With Metastatic Pancreatic Cancer and DNA Damage Repair Genes Alterations. [cited 2023 5/20]; Available online: https://clinicaltrials.gov/ct2/show/NCT05659914.