RRP12 suppresses cell migration and invasion in colorectal cancer cell via regulation of epithelial-mesenchymal transition
Highlight box
Key findings
• Ribosomal RNA processing 12 homolog (RRP12) is highly expressed in colorectal cancer (CRC) cell lines and clinical samples, associated with poor survival in CRC patients. Knockdown of RRP12 suppresses migration and invasion of CRC cells.
What is known and what is new?
• RRP12 as a nucleolar protein involved in ribosome subunit maturation and export, plays important roles in cell cycle-related processes and regulates the progression of various cancers. Bioinformatic-based analyses indicated that RRP12 could be a potential biomarker for prognostic assessment of CRC patients.
• RRP12 contributes to the EMT process of CRC cell lines in a ZEB1-mediated manner. RRP12 knockdown reverses metastasis of CRC cells in vivo.
What is the implication, and what should change now?
• RRP12 could be a potential therapeutic target for CRC therapy.
Introduction
Colorectal cancer (CRC) is the third most frequently diagnosed cancer and ranks fourth in cancer-related deaths worldwide (1). Most CRC patients are diagnosed at advanced stages due to the lack of obvious clinical symptoms. Metastatic CRC remains a major cause of death in patients despite substantial improvements in treatment options (2).
The epithelial-mesenchymal transition (EMT) process plays a crucial role in tumor metastasis (3). Epithelial cells lose polarity and intercellular contacts during EMT and acquire a mesenchymal phenotype. Accumulating evidence highlights that aberrant activation of EMT promotes tumor metastasis and leads to treatment resistance (4,5). The process of EMT is characterized by the loss of epithelial markers, such as E-cadherin and occludin, and the acquisition of mesenchymal markers, such as N-cadherin and vimentin. Studies have shown that transcription factors (TFs), such as Snail, Slug, and ZEB1/2, have the capability to regulate EMT during tumor progression. Zinc finger E-box binding homeobox 1 (ZEB1) is a key EMT-TF in the ZEB family and is involved in cellular plasticity, dissemination, and a dormant-to-proliferative phenotypic switch at the distant site as well as being a predictor of worse clinical outcomes in human cancers (6,7).
RRP12 is a stable component of both pre-40S and pre-60S particles, which mediates ribosomal subunit maturation and export (8). Research has demonstrated that RRP12 plays key roles in cell cycle processes and the repair of DNA damage (9,10). Here, we report some specific implications of RRP12 in the malignant biological processes of CRC. We had conducted bioanalysis previously, and found that RRP12 was clearly related to RNA transcription through Kyoto Encyclopedia of Genes and Genomes (KEGG) analysis results. Therefore, we considered that RRP12 might promote the invasion and metastasis of tumors by regulating TFs. Subsequently, we conducted reporter gene analysis and found that when RRP12 was interfered, only the expression of TF ZEB1 was abnormally increased, indicating that RRP12 promoted the invasion and metastasis of CRC through ZEB1. Nevertheless, the role of RRP12 in regulating EMT remains unclear.
In this study, we focused on the prognostic value and functional roles of RRP12 in CRC. The expression of RRP12 was assessed in clinical samples and the association between RRP12 expression and survival time of CRC patients was determined through The Cancer Genomes Atlas (TCGA) data. Knockdown of RRP12 was performed to investigate the influence on the aggressive biological behavior of CRC cells lines. The results showed that RRP12 knockdown in CRC cells inhibited the EMT process in vitro and in vivo. Meanwhile, we observed that RRP12 modulated the EMT process in a ZEB1-dependent manner. Our findings suggest that RRP12 could act as a prognostic biomarker of patients with CRC and as a therapeutic target for preventing cancer progression. We present this article in accordance with the ARRIVE and MDAR reporting checklists (available at https://jgo.amegroups.com/article/view/10.21037/jgo-23-254/rc).
Methods
Clinical specimen collection
During surgery, 6 fresh tissue specimens were collected, including cancer and cancer-adjacent tissues, in the Department of General Surgery of Shaanxi Provincial People’s Hospital. All patients were pathologically diagnosed with CRC. A total of 242 paraffin blocks of CRC tissues were selected with the clinicopathologic characteristics (reference period: January 2018 to June 2019). All patients had not received chemotherapy or radiotherapy before surgery. The research protocol was approved by the ethics committee of Shaanxi Provincial People’s Hospital (No. 2022R013), and obtained written informed consent was provided by all participants. The study was conducted in accordance with the Declaration of Helsinki (as revised in 2013).
Cell culture
All the colon cancer cell lines (HCT116, RKO, SW480, and LoVo) used in this study were purchased from Shanghai Cell Bank (the Chinese Academy of Sciences, Shanghai, China). Cells were cultured in Roswell Park Memorial Institute (RPMI) 1640 media (Invitrogen, Life Technologies, Carlsbad, CA, USA), supplemented with 10% fetal bovine serum (FBS, Gibco, Gaithersburg, MD, USA), 100 U/mL penicillin, and 100 µg/mL streptomycin (Life Technologies) according to the recommended culture method and incubated at 37 ℃ with a 5% CO2 atmosphere.
Cell transfection
RRP12 short hairpin RNA (shRNA; shRRP12) and negative control (shNC) were purchased from GeneChem Co., Ltd. (Shanghai, China). Cells (HCT116, SW480) were transfected with lentiviral particles [multiplicity of infection (MOI) =20], polybrene (5 µg/mL). pcDNA3.1-RRP12 and the corresponding control were obtained from GenePharma, Co., Ltd. (Suzhou, China) and transfected into HCT116 and SW480 cells respectively, according to the manufacturer’s protocol. In order to produce stable transfected cells, 100 transfected cells were seeded into a 10-cm Petri dish, with the medium changed 3 times a week. After 3 weeks, puromycin-resistant colonies were isolated and seeded into 96-well plates for the follow-up study.
Real-time polymerase chain reaction
TRIzol reagent (Invitrogen, USA) was used to extract total RNA from colorectal cells. Complementary DNA (cDNA) was synthesized by using a PrimeScript RT reagent kit (Fermentas, Beijing, China). The real-time polymerase chain reaction (RT-PCR) was performed using a SYBR Green PCR Kit (TaKaRa, Dalian, China), in the light of the manufacturer’s instructions. Glyceraldehyde 3-phosphate dehydrogenase (GAPDH) was used as the internal control, and the relative expression levels were assessed using the ΔΔCt method. The primers sequences for RT-PCR are listed in Table 1.
Table 1
Gene name | Sequence (5'-3') |
---|---|
TWIST | Forward: TTCAAAGAAACAGGGCGTGG |
Reverse: CCTTTCAGTGGCTGATTGGC | |
ZEB1 | Forward: TCCTGTCTAGAAGCAGATACGA |
Reverse: GCCTATGCTCCACTCCTTGC | |
RRP12 | Forward: GAATCCGGAAGTGACGCCAG |
Reverse: CGACTGTCAGGTCACTCCTTC | |
SNAIL | Forward: AAGATGCACATCCGAAGCCA |
Reverse: CATTCGGGAGAAGGTCCGAG | |
SLUG | Forward: AGACCCCCATGCCATTGAAG |
Reverse: CTTCTCCCCCGTGTGAGTTC | |
SIP1 | Forward: CCTCTGTAGATGGTCCAGTGA |
Reverse: GTTCCAGGTGGCAGGTCATT | |
GAPDH | Forward: AAAGCCTGCCGGTGACTAAC |
Reverse: TTCCCGTTCTCAGCCTTGAC |
RT-qPCR, real-time quantitative polymerase chain reaction.
Western blot
Radioimmunoprecipitation assay (RIPA) reagent was used to extract total protein from the stable transfected cell lines. The same quantity of protein (150 µg) was separated by 10% sodium dodecyl sulfate polyacrylamide gel electrophoresis (SDS-PAGE) and then transferred onto a polyvinylidene fluoride (PVDF) membrane. After incubation in 5% (w/v) skim milk powder dissolved in tris-buffered saline with Tween 20 (TBST) for 2 hours at room temperature, the immunoblots were sequentially probed with primary and secondary antibodies (anti-E-cadherin, CST, cat. no. 14472; anti-Fironecin, abcam, cat.no.ab6328; ani-β-Catenin, CST, cat.no.8480; ani-Mouse IgG, Santa-Cruz, cat.no.sc-2005; anti-Rabbit IgG, Santa Cruz, cat.no.sc-2004; anti-RRP12, genetex, cat.no.GTX120180). β-actin was used as the control group. Protein bands were visualized with enhanced chemiluminescence (ECL) reagent and then quantified using Image-Pro Plus 6.0 software (Media Cybernetics, Rockville, MD, USA).
Wound healing assay
Cells were cultured in a 6-well plate at a density of 5×105 cells/well. After confluence, the HCT116 and SW480 cells were scratched by a circle line using a sterile pipette tip. After that, PBS was used to wash cells for removing cell debris three times. The photographs were recorded at 8 and 48 hours to investigate the cell migration ability. An inverted microscope was used to obtain digital photographs.
Transwell assay
Cell invasion assay was carried out in the Transwell plates pre-coated with Matrigel at 37 ℃ for 1 hour [Becton, Dickinson, and Co. (BD) Biosciences, Franklin Lakes, NJ, USA]. HCT116 and SW480 cells were seeded into the upper component of the chamber at 5×104 cells/well in serum-free RPMI 1640 medium. The bottom wells of the chamber were filled with medium containing 10% FBS. After incubation at 37 ℃ for 48 hours, the cells on both sides of the Transwell membrane were fixed with 4% paraformaldehyde at 37 ℃ for 30 minutes and stained with 0.5% crystal violet for 5 minutes at room temperature. The cell migration assay was performed in a similar manner except that the upper cells that did not cross the Transwell membrane were removed smoothly before fixing and staining.
Detection of cell proliferation and viability
For cell proliferation analysis, the transfected HCT116 and SW480 cells were seeded at a concentration of 5×103 cells/well into 96-well plates. After incubation at 37 ℃ for 24 hours, 3-(4,5-dimethylthiazolyl-2)-2,5-diphenyltetrazolium bromide (MTT) assay (Boster, Wuhan, China) was performed to detect the cells’ viability. For cell apoptosis assessment, cells were processed using an Annexin-V FITC Apoptosis Detection Kit (Invitrogen, USA) after incubation for 48 hours and were then detected through flow cytometry (Tree Star, Inc., Ashland, OR, USA).
Tumor xenograft model
All the 6-week-old BALB/c nude female mice (purchased from Shanghai Ling Chang Biological Technology Co., Ltd., Shanghai, China) were divided into 2 groups, with four mice in each group. The mice were housed in SPF-level laboratories with free access to food and water and accommodated for 1 week prior to any experiments. The animal experiments were performed under a project license (No. 2022004) granted by the Ethics Committee of Medical and Biological Research of Medical school of Yan’an University, cooperated with Shaanxi Provincial People’s Hospital (the Third Affiliated Hospital of Xi’an Jiaotong University), in compliance with institutional guidelines for the care and use of animals. A protocol was prepared before the study without registration. The CRC cells were injected into the tail vein of the mice. After 4 weeks, these mice were observed by an IVIS imaging system (IVIS spectrum; Caliper Life Sciences, Mountain View, CA, USA). After the mice were sacrificed, the lung tissues with carcinoma metastases were isolated for paraffin embedding to perform hematoxylin and eosin (H&E) staining.
Immunohistochemistry assay
Both the sections of paraffin-embedded and formalin-fixed tumor tissues were deparaffinized in xylene and rehydrated through graded ethanol. Then, 3% H2O2 in methanol was used to block the endogenous peroxidase activity for 5 minutes at room temperature. Next, the sections were washed with phosphate-buffered saline (PBS) 3 times and incubated with goat serum for protein blocking. After incubation with the specific antibody of RRP12 at 4 ℃ overnight, the sections were washed with PBS another 3 times. At last, the sections were incubated with secondary antibodies for 30 minutes at room temperature, then counterstained with hematoxylin, dehydrated in solutions with graded alcohol, and mounted in Malinol (Muto Pure Chemicals, Tokyo, Japan).
Bioinformatics analysis
The Gene Expression Profiling Interactive Analysis (GEPIA; http://gepia.cancer-pku.cn/) web server, containing TCGA and Genotype-Tissue Expression (GTEx) datasets was used to conduct bioinformatics analysis. First, we uploaded the RRP12 gene to verify the consistency of gene expression between colorectal cell lines and TCGA/GTEx colon adenocarcinoma (COAD) datasets. Then we set the parameters as the threshold criteria: for example, log2FC cut off: 1.0 and P value cutoff: 0.01. Next, we performed analysis of overall survival (OS) and disease-free survival (DFS) of CRC patients. The patients in the TCGA/GTEx dataset were divided into high and low expression groups of RRP12 using the median transcripts per kilobase million (TPM) as a break point, and the significance was determined through a log-rank test with P<0.05. Then, functional enrichment analysis was performed using KEGG and Gene Ontology (GO) enrichment analysis to confirm the final potential key genes with the filter parameter, with P<1×10−10 as the threshold.
Statistical analysis
Statistical analysis was carried out using SPSS19.0 (IBM Corp., Armonk, NY, USA). All the data were presented as mean ± standard deviation (). Comparison of variables among different groups was carried out through one-way analysis of variance (ANOVA) followed by the post hoc Tukey’s multiple comparison test, 2-tailed Student’s t-test, or Fisher’s exact test as necessary. A Kaplan-Meier survival curve was applied to observe the influence of RRP12 expression on the prognosis of CRC patients. A P value <0.05 was considered statistically meaningful when the difference was shown. All experiments were repeated at least 3 times independently.
Results
RRP12 is highly expressed in CRC cell lines and clinical samples, associated with poor survival in CRC patients
To verify the potential role of RRP12 in the progression and prognosis of CRC, 3 pairs of non-metastatic and metastatic CRC tissue specimens were collected and the expression level of RRP12 were characterized by immunohistochemistry. High RRP12 expression was observed in the metastatic CRC specimens, whereas little staining was observed in non-metastatic specimens (Figure 1A). Western blot and RT-PCR analysis suggested that CRC tissues from metastatic disease had higher levels of RRP12 expression than those without metastasis, significantly (Figure 1B,1C). High levels of RRP12 expression were also detected in different types of CRC cell lines (Figure 1D). Kaplan-Meier curve analysis of datasets from TCGA was applied to evaluate the value of RRP12 in CRC prognosis, and indicating that high expression of RRP12 in CRC samples was associated with shorter OS (Figure 1E). These results indicated that aberrant expression of RRP12 may impact the prognosis of CRC. HCT116 and SW480 cells were chosen for further study.
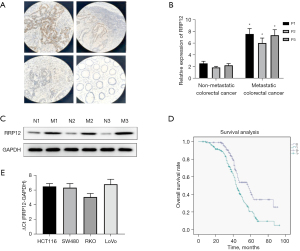
Correlation between RRP12 expression and clinicopathological characters in patients with CRC
Since the high expression of RRP12 was assessed in CRC tissues and cell lines, we next evaluated the correlation between RRP12 and clinical features. As shown in Table 2, among a total of 242 cases of CRC tissues, there was no significant association between RRP12 expression and age or gender (P=0.658 and P=0.94, respectively, Table 2). Hence, RRP12 expression was positively associated with lymph node metastasis, tumor-node-metastasis (TNM) stage, and poor differentiation in this population (P=0.046, P=0.002, and P=0.001, respectively, Table 2). These results all indicated that up-regulation of RRP12 possibly participates in the progression and metastasis of CRC.
Table 2
Characteristic | Number (total n=242) | RRP12 expression | P value | |
---|---|---|---|---|
Positive (n=135) | Negative (n=107) | |||
Age, years | 0.658 | |||
≥55 | 153 | 87 (56.9) | 66 (43.1) | |
<55 | 89 | 48 (53.9) | 41 (46.1) | |
Sex | 0.94 | |||
Male | 126 | 70 (55.6) | 56 (44.4) | |
Female | 116 | 65 (56.0) | 51 (44.0) | |
Differentiation degree | 0.001 | |||
High | 63 | 31 (49.2) | 32 (50.8) | |
Moderate | 92 | 36 (39.1) | 56 (60.9) | |
Low | 87 | 58 (66.7) | 29 (33.3) | |
TNM staging | 0.002 | |||
I | 40 | 13 (32.5) | 27 (67.5) | |
II | 57 | 29 (50.9) | 28 (49.1) | |
III | 73 | 43 (58.9) | 30 (41.1) | |
IV | 72 | 50 (69.4) | 22 (30.6) | |
Lymph node metastasis | 0.046 | |||
Yes | 174 | 104 (59.8) | 70 (40.2) | |
No | 68 | 31 (45.6) | 37 (54.4) |
Data are presented as n (%). TNM, tumor-node-metastasis.
Knockdown of RRP12 suppresses migration and invasion of CRC cells
In order to better understand the significance of RRP12 in CRC, stably RRP12-silencing cells and RRP12 over-expressing cells were generated. The knockdown and over-expressing efficiency of RRP12 was detected in CRC cells by RT-PCR and western blot assay (Figure 2A-2C). Then, the effect of RRP12 on the migration and invasion in both HCT116 and SW480 cell lines were investigated. Wound-healing assay (Figure 2D,2E) and Transwell assay (Figure 2F,2G) were conducted and cell motility was quantified. The results showed that RRP12 knockdown strongly inhibited, whereas RRP12 over-expressing strongly enhanced, both cell migration and invasion, compared with the shNC group in both cell lines, respectively (P<0.01, Figure 2D,2E; P<0.001, Figure 2F,2G). Thus, RRP12 was confirmed to promote cell motility and invasion capabilities of both HCT116 and SW480 cells.

RRP12 contributes to EMT process of CRC cell lines in a ZEB1-mediated manner
EMT plays an important role in tumor development and metastasis. TFs have been demonstrated to be associated with EMT in cancer progression, such as Twist, Snail, Slug, ZEB1, and SIP1 (3). In order to determine whether RRP12 participates in the process of EMT in CRC cells, stable RRP12-shRNA-transfected cells were established, and the expression levels of the above TFs were tested. Notably, the results showed that with the down-regulation of RRP12, only the expression levels of ZEB1 decreased significantly, whereas the other TFs had no obvious changes (Figure 3A,3B). Next, the relative levels of E-cadherin, fibronectin, β-catenin, and ZEB1 in RRP12 silencing and over-expressing CRC cells were detected through western blot assays. Compared with shNC cells, significantly increased expression of E-cadherin and β-catenin, but decreased expression of fibronectin and ZEB1, were detected in shRRP12 CRC cells (Figure 3C). A similar pattern of E-cadherin, fibronectin, β-catenin, and ZEB1 expression was detected in RRP12 over-expressing CRC cells (Figure 3D). Based on the above results, we proposed that RRP12 might be involved in the transcriptional regulation of ZEB1. We knocked down ZEB1 expression by shRNA in both HCT116 and SW480 cells lines and tested EMT markers through western blot assays (Figure 3E,3F). The ZEB1 knockdown cells showed up-regulation of E-cadherin and down-regulation of fibronectin. However, the expression levels of RRP12 had no significant change (Figure 3G). Next, we endeavored to investigate the role of ZEB1 in EMT process induced by RRP12. We proposed that the up-regulation of ZEB1 would restore the EMT. To explore whether RRP12 regulates EMT through ZEB1, stable shNC cells or shRRP12 cells were transfected with pcDNA3.1(+)-vector or pcDNA3.1(+)-ZEB1 respectively. Western blot assay showed that over-expression of ZEB1 could decrease the expression of the epithelial markers such as E-cadherin and restored the expression of the mesenchymal markers such as fibronectin (Figure 3H). Moreover, ZEB1 up-regulation significantly enhanced the migration and invasion abilities of shRRP12 cells (Figure 3I,3J). Taken together, our data suggest that ZEB1 plays a key role in RRP12-induced EMT.
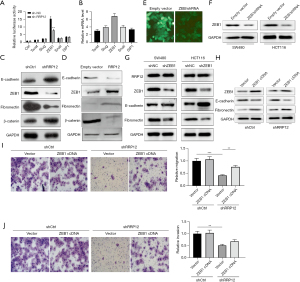
RRP12 knockdown reverses metastasis of CRC cells in vivo
The above results revealed that RRP12 knockdown reverses the EMT process and inhibits metastasis in CRC cells in vitro, which inspired us to explore whether silencing of RRP12 can inhibit metastasis and reverse EMT in vivo. Both HCT116 cells with RRP12 down-regulation and SW480 cells with RRP12 up-regulation were injected through the tail vein of 6-week-old nude mice, and the formation of lung metastases in mice was observed. The results showed that lung metastases grew much more slowly in the HCT116 shRRP12 group than in the shNC group (Figure 4A), whereas the tumor volume of lung metastases was bigger in the RRP12 up-regulation group than that in the shNC group in SW480 cells (Figure 4B). RT-PCR analysis displayed a decreased expression (Figure 4C) of ZEB1 in the shRRP12 group, but an increased expression in the RRP12 up-regulation group (Figure 4D), Western blot assay was carried out to analyze the expression levels of E-cadherin and fibronectin for the EMT-related markers in HCT116 cells with RRP12 down-regulation (Figure 4E) and SW480 cells with RRP12 up-regulation (Figure 4F), respectively, which was consistent with the results of experiments in vitro.
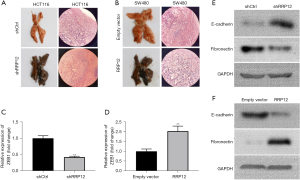
Identification of potential genes and pathways related with RRP12 through bioinformatic-based analyses
To determine whether RRP12 could be a potential biomarker for prognostic assessment of CRC patients, we collected and examined the datasets from TCGA and GTEx. The results showed that there was a significant overexpression of RRP12 in COAD compared with normal colorectal mucosa tissue (Figure 5A). In addition, RRP12 is up-regulated significantly in gastric cancer based on TCGA datasets (Figure 5B). Based on Gene Expression Omnibus (GEO) database analysis, patients with low-expression of PPR12 have longer OS and progression-free survival (PFS) than high-RRP12 expression, with significant difference in OS but a non-significant difference in PFS (Figure 5C,5D). To better understand the biological functions and cell localization of the RRP12 gene, GO analyses were conducted (Figure 5E). To assess the enrichment in cell signaling pathways of deferent expression of RRP12, KEGG (Figure 5F) was used to analyze the metabolic pathways that had significantly changed under experimental conditions, which is particularly important in mechanism studies.
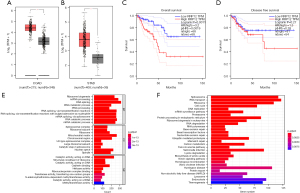
Discussion
In the current study, RRP12 knockdown participated in the EMT reversal process both in vitro and in vivo, and was shown to reduce the migration and invasion abilities of CRC cells. A positive association between RRP12 and progression of CRC cells was found, thus presenting a potential biomarker for the metastasis of CRC cells. Furthermore, this study indicated that the EMT process which was induced by PPR12 is mainly ZEB1-dependent in CRC cells.
PPR12, which involved in ribosome biosynthesis, plays crucial roles in cell cycle-related processes and the response to DNA damage (9). Existing evidence indicates that the morphological and functional changes of nucleoli are widespread in cancer cells, which are the result of increased demand for ribosomal organisms and are also characteristic of cell proliferation. Therefore, identifying the mechanisms involved could help to control cell proliferation. Increased ribosomal biogenesis might critically contribute to neoplastic transformation, by affecting translation as well as synthesis of proteins that play important roles in the process of tumorigenesis (11-13). Recent studies showed that RRP12 was crucial for cell survival during cytotoxic drug stress by inhibiting the stability of P53 in osteosarcoma cells. Therefore, targeting RRP12 may enhance the efficacy of chemotherapy for cancer (10,14).
TCGA and GEPIA are international public repositories that contain numerous datasets of cancer genomes. Several genes with diagnostic value for CRC have been found using these databases (15,16). A total of 9 differentially expressed genes (DEGs) involved in prognostic of COAD were analyzed from GEPIA datasets, and it was found that high expression of RRP12 participates in promoting cell growth and is relative to short survival of COAD patients. In addition, RRP12 is an essential gene in pan-cancer, including COAD (17,18). Likewise, using bioinformatics analysis, we found that RRP12 was expressed at significantly higher levels in CRC compared with normal colorectal mucosa tissue. Our study found that RRP12 is highly expressed in CRC cell lines and clinical samples, and associated with poor survival in CRC patients. Knockdown of RRP12 suppresses migration and invasion abilities of CRC cells. EMT-related markers and TFs were examined in CRC cells in this study. Epithelial markers, including E-cadherin and β-catenin, were upregulated, whereas mesenchymal markers, such as fibronectin, were markedly downregulated. In our study, ZEB1 exhibited a significantly decreased expression in shRRP12 CRC cells. From this standpoint, we propose that PPR12 induces EMT in a ZEB1-dependent manner in CRC cells.
ZEB1 belongs to the ZEB family of TFs, which binds to E-boxes of regulatory gene sequences, and acts as transcription repressors and activators, thereby suppressing epithelial genes and activating mesenchymal genes, respectively (19). Research has shown that ZEB1 is present in invasive regions of CRC, binding to E-box of CDH1 promoter and resulting in activation of the EMT process by repressing the expression of E-cadherin (20). Additionally, ZEB1 was found to reduce the expression of several cell polarity genes, resulting in impaired epithelial differentiation. ZEB1 could also induce drug resistance through EMT- dependent and independent manners, in which ZEB1 participates in DNA reparation through interacting with USP7 to stabilize CHK1 (21). Here, our data indicated that ZEB1 not only promotes tumor cell dissemination, but is necessary for RRP12-induced EMT in CRC cells. Combining our findings, we can infer that increased expression of RRP12 induced activation of ZEB1, which in turn promoted the EMT process of CRC cells. However, the exact mechanism between ZEB1 and RRP12 needs further investigation.
Taken together, observations in our study suggest that PPR12-induced EMT is mainly ZEB1-dependent, which acts as a mediator for enhancement of migration and invasion in CRC. Thus, targeting RRP12 may represent a promising direction for fatal tumor types, especially CRC.
Conclusions
Recurrence and metastasis of tumors induced by EMT is the main cause of mortality in advanced cancer patients. In this study, RRP12 knockdown increased expression of E-cadherin and β-catenin, reversed the EMT process in vitro and in vivo, and inhibited the motility and invasion capacities of CRC cells. Furthermore, ZEB1 was shown to be crucial for the RRP12-induced EMT in CRC. Thus, we believe that high expression of RRP12 to promote EMT and further enhance the capacity of migration and invasion in a ZEB1-dependent manner in CRC is demonstrable. Extra studies are necessary to elucidate the further mechanism between RRP12 and ZEB1 in regulating EMT. This study indicates that targeting RRP12 could be a potential approach to prevent CRC progression.
Acknowledgments
Funding: This work was financially supported by
Footnote
Reporting Checklist: The authors have completed the ARRIVE and MDAR reporting checklists. Available at https://jgo.amegroups.com/article/view/10.21037/jgo-23-254/rc
Data Sharing Statement: Available at https://jgo.amegroups.com/article/view/10.21037/jgo-23-254/dss
Peer Review File: Available at https://jgo.amegroups.com/article/view/10.21037/jgo-23-254/prf
Conflicts of Interest: All authors have completed the ICMJE uniform disclosure form (available at https://jgo.amegroups.com/article/view/10.21037/jgo-23-254/coif). The authors have no conflicts of interest to declare.
Ethical Statement: The authors are accountable for all aspects of the work in ensuring that questions related to the accuracy or integrity of any part of the work are appropriately investigated and resolved. The research protocol was approved by the ethics committee of Shaanxi Provincial People’s Hospital (No. 2022R013), and obtained written informed consent was provided by all participants. The study was conducted in accordance with the Declaration of Helsinki (as revised in 2013). The animal experiments were performed under a project license (No. 2022004) granted by the Ethics Committee of Medical and Biological Research of Medical school of Yan’an University, cooperated with Shaanxi Provincial People’s Hospital (the Third Affiliated Hospital of Xi’an Jiaotong University), in compliance with institutional guidelines for the care and use of animals.
Open Access Statement: This is an Open Access article distributed in accordance with the Creative Commons Attribution-NonCommercial-NoDerivs 4.0 International License (CC BY-NC-ND 4.0), which permits the non-commercial replication and distribution of the article with the strict proviso that no changes or edits are made and the original work is properly cited (including links to both the formal publication through the relevant DOI and the license). See: https://creativecommons.org/licenses/by-nc-nd/4.0/.
References
- Bray F, Ferlay J, Soerjomataram I, et al. Global cancer statistics 2018: GLOBOCAN estimates of incidence and mortality worldwide for 36 cancers in 185 countries. CA Cancer J Clin 2018;68:394-424. [Crossref] [PubMed]
- Siegel RL, Miller KD, Goding Sauer A, et al. Colorectal cancer statistics, 2020. CA Cancer J Clin 2020;70:145-64. [Crossref] [PubMed]
- Goossens S, Vandamme N, Van Vlierberghe P, et al. EMT transcription factors in cancer development re-evaluated: Beyond EMT and MET. Biochim Biophys Acta Rev Cancer 2017;1868:584-91. [Crossref] [PubMed]
- Lu W, Kang Y. Epithelial-Mesenchymal Plasticity in Cancer Progression and Metastasis. Dev Cell 2019;49:361-74. [Crossref] [PubMed]
- Aiello NM, Kang Y. Context-dependent EMT programs in cancer metastasis. J Exp Med 2019;216:1016-26. [Crossref] [PubMed]
- Spaderna S, Schmalhofer O, Wahlbuhl M, et al. The transcriptional repressor ZEB1 promotes metastasis and loss of cell polarity in cancer. Cancer Res 2008;68:537-44. [Crossref] [PubMed]
- Wellner U, Schubert J, Burk UC, et al. The EMT-activator ZEB1 promotes tumorigenicity by repressing stemness-inhibiting microRNAs. Nat Cell Biol 2009;11:1487-95. [Crossref] [PubMed]
- Oeffinger M, Dlakic M, Tollervey D. A pre-ribosome-associated HEAT-repeat protein is required for export of both ribosomal subunits. Genes Dev 2004;18:196-209. [Crossref] [PubMed]
- Dosil M. Ribosome synthesis-unrelated functions of the preribosomal factor Rrp12 in cell cycle progression and the DNA damage response. Mol Cell Biol 2011;31:2422-38. [Crossref] [PubMed]
- Zhang Y, Li CY, Ge W, et al. Exploration of the Key Proteins in the Normal-Adenoma-Carcinoma Sequence of Colorectal Cancer Evolution Using In-Depth Quantitative Proteomics. J Oncol 2021;2021:5570058. [Crossref] [PubMed]
- Montanaro L, Treré D, Derenzini M. Nucleolus, ribosomes, and cancer. Am J Pathol 2008;173:301-10. [Crossref] [PubMed]
- Choi YJ, Lee HW, Lee YS, et al. RRP12 is a crucial nucleolar protein that regulates p53 activity in osteosarcoma cells. Tumour Biol 2016;37:4351-8. [Crossref] [PubMed]
- Zhang H, Xia P, Ma W, et al. Development and Validation of an RNA Binding Protein-associated Prognostic Model for Hepatocellular Carcinoma. J Clin Transl Hepatol 2021;9:635-46. [Crossref] [PubMed]
- Wei C, Wang B, Chen ZH, et al. Validating RRP12 Expression and Its Prognostic Significance in HCC Based on Data Mining and Bioinformatics Methods. Front Oncol 2022;12:812009. [Crossref] [PubMed]
- Sun X, Hu Y, Zhang L, et al. Mining, Validation, and Clinical Significance of Colorectal Cancer (CRC)-Associated lncRNAs. PLoS One 2016;11:e0164590. [Crossref] [PubMed]
- Galamb O, Kalmár A, Barták BK, et al. Aging related methylation influences the gene expression of key control genes in colorectal cancer and adenoma. World J Gastroenterol 2016;22:10325-40. [Crossref] [PubMed]
- Hu M, Fu X, Si Z, et al. Identification of Differently Expressed Genes Associated with Prognosis and Growth in Colon Adenocarcinoma Based on Integrated Bioinformatics Analysis. Front Genet 2019;10:1245. [Crossref] [PubMed]
- Zhu F, Wei J, He D, et al. The miRNA125a-5p and miRNA125b-1-5p cluster induces cell invasion by down-regulating DDB2-reduced epithelial-to-mesenchymal transition (EMT) in colorectal cancer. J Gastrointest Oncol 2022;13:3112-22. [Crossref] [PubMed]
- Vu T, Datta PK. Regulation of EMT in Colorectal Cancer: A Culprit in Metastasis. Cancers (Basel) 2017;9:171. [Crossref] [PubMed]
- Spaderna S, Schmalhofer O, Hlubek F, et al. A transient, EMT-linked loss of basement membranes indicates metastasis and poor survival in colorectal cancer. Gastroenterology 2006;131:830-40. [Crossref] [PubMed]
- Zhang P, Sun Y, Ma L. ZEB1: at the crossroads of epithelial-mesenchymal transition, metastasis and therapy resistance. Cell Cycle 2015;14:481-7. [Crossref] [PubMed]
(English Language Editor: J. Teoh)