Management and mechanism of calciphylaxis in a patient treated with the FGFR inhibitor pemigatinib—a case report
Highlight box
Key findings
• We describe a rare complication of calciphylaxis associated with pemigatinib, a small molecule fibroblast growth factor receptor (FGFR)2 inhibitor. The patient’s treatment plan and proposed pathophysiology are discussed.
What is known and what is new?
• Pemigatinib associated calciphylaxis has been described before in two patients taking pemigatanib with a similar method of treatment.
• Currently, no literature describes such a rare case while simultaneously highlighting the complicated molecular signaling and proposed mechanism underlying calciphylaxis.
What is the implication, and what should change now?
• This case report serves as a roadmap for not only the treatment and understanding of calciphylaxis in patients on pemigatinib therapy, but also to better recognize the signs, symptoms, and laboratory data to begin care in a timely manner. calciphylaxis can help patients avoid switching from their FGFR inhibitor therapy.
Introduction
The fibroblast growth factor (FGF) pathway has become a promising target for drug development in recent years. Genetic mutations and rearrangements in FGF receptors (FGFRs) were described in the development and progression of various cancer types (1). Several FGFR2 and FGFR3 inhibitors are approved for clinical use in cholangiocarcinoma (CCA) and bladder cancer, while others progress through various phase II/III clinical trials (1).
Pemigatinib, a small molecule inhibitor of FGFRs (FGFR1–3), received accelerated approval in April 2020 in the USA for the treatment of patients with locally advanced or metastatic CCA, harboring FGFR2 fusions or rearrangement, who failed on front-line gemcitabine-based systemic therapy. This approval is based on results from FIGHT 202, a phase 2 multicenter, open-label, single-arm clinical trial in adults with disease progression following at least one previous treatment, treated with pemigatinib (2). Use of pemigatinib, like other FGFR inhibitor (FGFRi), is associated with hyperphosphatemia and dermatological adverse events (AEs) (3). Rarely, FGFRi can cause calciphylaxis, triggered by unique roles of FGF signaling in calcification and vascular biology. In this case report, we describe a patient with metastatic CCA who developed calciphylaxis after beginning pemigatinib therapy that responded to disease modifying therapy. We also describe the molecular underpinnings of FGFR inhibition in relation to calcium tissue deposition and vascular calcification, along with management strategy for this rare and serious side effect. We present this case in accordance with the CARE reporting checklist (available at https://jgo.amegroups.com/article/view/10.21037/jgo-23-139/rc).
Case presentation
A 50-year-old Caucasian male with past medical history of cirrhosis, secondary to nonalcoholic steatohepatitis (since 2015), was diagnosed with CCA in October 2017. This patient also has history of thrombocytopenia secondary to his liver disease for which he underwent a partial splenic embolization. This was then complicated by a splenic vein thrombosis for which anticoagulation (enoxaparin 1 mg/kg twice daily for a month and later 1.5 mg/kg/day) was started. At the time of his CCA diagnosis, the patient was found to have a 7.5 cm lesion in segment 2 of the liver. His alpha-fetoprotein (AFP) level was normal. As such, biopsy of the liver lesion revealed adenocarcinoma, consistent with CCA. He underwent left lobar yttrium-90 treatment in February 2018. A repeat CT scan in 2 months revealed metastatic disease in the lungs and mediastinal lymph nodes. Subsequently, the patient was started on palliative combination chemotherapy with gemcitabine and carboplatin in July 2018. After 17 months, his disease progressed. Subsequently patient was enrolled on a phase II clinical trial, involving 5-fluorouracil (5-FU), liposomal irinotecan, and nivolumab in December 2019. This regimen lasted 3 months. Next-generation sequencing of his tumor revealed FGFR2 fusion (FGFR2-TAX1BP1). The patient was then started on pemigatinib (13.5 mg/day) in June 2020. He was found to have profound hyperphosphatemia (phosphorous level 7.5 mg/dL (normal range, 2.7–4.5 mg/dL) a week after the initiation of therapy initiation (Figure 1). Of note, patient has normal kidney functions (blood urea nitrogen, creatinine, filtration rate) calcium, and phosphorous level before starting pemigatinib. Sevelamer (an oral phosphate binder) was started and pemigatinib was held. With improvement in phosphorus level, pemigatinib therapy was reinitiated at a lower dose of 9 mg/day). Over the next month, he was noted to have rebound hyperphosphatemia and bilateral tender, stellate, purpuric and blistering plaques with an erythematous border over the patient’s inner thighs (Figure 2). Pemigatinib was discontinued. He was started on empiric antibiotic therapy. Further workup revealed normal white blood cell count, renal functions, and vitamin D level. C-reactive protein of 22.9 mg/dL (normal values, 0.1–0.5 mg/dL), parathyroid hormone (PTH) of 10.1 pg/mL (reference range, 15–65 pg/mL), and negative blood/wound cultures, anti-neutrophil cytoplasmic antibodies (ANCAs), anti-nuclear antibodies (ANAs), rheumatoid factor (RF), cryoglobulin, and lupus anticoagulant. CT imaging of the extremities was negative for superficial and deep tissue infection. Work up failed to reveal infectious or autoimmune causes of skin lesions; therefore, a skin biopsy was pursued. A punch biopsy revealed subcutaneous septal, focal vascular and dermal calcifications with no evidence of leukocytoclastic vasculitis or carcinoma (Figure 3) consistent with calciphylaxis. Cultures from skin biopsy were negative.
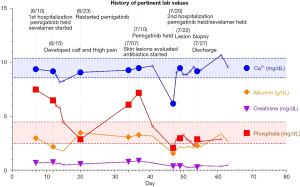
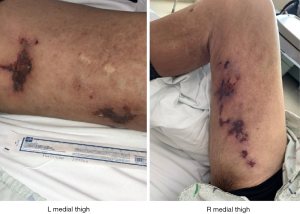
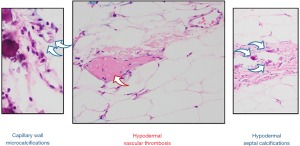
The patient’s management was coordinated via a multi-disciplinary team. Pain was addressed with morphine, oxycodone, gabapentin, and topical 1% ketamine cream. Intravenous sodium thiosulfate (STS) was briefly initiated and switched to topical STS with xeroform dressings for outpatient management, along with oral diltiazem. Three weeks after initiation of this treatment, his painful lesions were noted to improve significantly PTH and phosphate levels normalized. The patient elected to pursue hospice care and passed away about 2 months after stopping therapy due to disease progression.
All procedures performed in this study were in accordance with the ethical standards of the institutional and/or national research committee(s) and with the Helsinki Declaration (as revised in 2013). Since the patient was deceased, written informed consent was obtained from the patient’s wife for the publication of this case report and accompanying images. A copy of the written consent is available for review by the editorial office of this journal.
Discussion
CCA is a malignancy of bile ducts, arising from intrahepatic, and extrahepatic bile ducts, with heterogeneity in etiology, clinical features, molecular targets, and treatments (4). The incidence of CCA in the United States and around the world is increasing (5). Depending upon the stage of the disease, treatment includes surgery (hepatobiliary resection), local therapies including embolization, stereotactic radiation, chemotherapy, and targeted therapies (6). About 60% of the patients will present with a low chance for resectability, because of locally advanced nature or metastatic disease. Recent developments in CCA treatment clinical trials led to the approval of chemo/immunotherapy (gemcitabine/cisplatin/durvalumab) as first-line therapy (7). Second-line therapies include 5-FU-based chemotherapy and targeted therapies. The advances in molecular profiling utilizing next-generation sequencing platforms in CCA patients led to the identification of driver mutations. These mutations/aberrations include IDH1/2 mutations, HER2 amplification/mutations, FGFR2 fusion and rearrangement, aberrations in the RAS/RAF pathway (KRAS, BRAF), deficient mismatch repair pathway, and high tumor mutational burden (TMB). There are several other mutations with low frequency including NTRK, and RET oncogene aberrations (4).
The human FGFR family (FGFR1–4) are highly conserved transmembrane receptor tyrosine kinases, crucial to organogenesis, tissue repair/regeneration, cell survival, and proliferation (1). As somatic aberrations in FGFs and their receptors are known drivers of several solid tumors, several novel selective and pan-FGFRis are in clinical development. Pemigatinib is an FGFRi with sub-nanomolar activity against FGFR1–3 approved in 2020 based on the open-label single-arm FIGHT-202 trial for metastatic CCA (2). In this trial, median duration of response was 9.1 months and an overall response rate of 36% in heavily pretreated population. Of these patients, 45% experienced serious AEs. Dose interruptions due to an AE occurred in 43% of patients, dose reductions occurred in 14%, and permanent discontinuation occurred in 9%. In pemigatinib clinical trials, hyperphosphatemia was seen in 92% of patients. The mean time to develop hyperphosphatemia was 8 days. Most of the events are low grade and only 29% of patients required phosphate lowering therapy (4). Dermatological toxicities were also common with FGFRis such as pemigatinib. Common dermatological side effects are alopecia, nail toxicity, dry skin, and palmar-plantar erythrodysesthesia syndrome (PPES). Most of these are grade 1–2 and easily manageable. However, calciphylaxis is very rare and only a few cases are reported, especially for pemigatinib (8,9). Calciphylaxis is defined by the deposition of calcium hydroxyapatite in the skin and soft tissues. Calciphylaxis is associated with ischemic skin lesions from crystalline deposition in the dermal microvasculature and subsequent thrombosis, in contrast to metastatic calcinosis cutis (MCC), associated with nodular crystalline skin deposits (10). Few cases of FGFRi-associated MCC have been reported in association with use of infigratinib, pemigatinib, and an undisclosed pan-FGFRi. Calciphylaxis has been described in patients taking pemigatinib and erdafitinib. Patients with calciphylaxis often suffer from significant morbidity, experiencing frequent hospitalizations, excruciating pain, and sequelae of poor wound healing. With 1-year mortality rates around 50%, mitigating or reversing the condition becomes vitally important (10). While calciphylaxis often occurs when the product of calcium and phosphorus serum levels exceed 70 mg2/dL2 or with kidney dysfunction, it infrequently occurs in the absence of any derangement in the kidney-vascular-bone axis (11). Risk factors for developing calciphylaxis include female sex, end-stage renal disease, warfarin use, systemic hypercoagulability, connective tissue diseases, repeated skin trauma from injections, diabetes, and liver disease to name the most prevalent (6). Among these, our patient only had liver disease and low molecular weight heparin (enoxaparin) as risk factors. Our patient developed characteristic histopathology of calciphylaxis with microvasculature involvement while having normal renal function. While the patient’s skin lesions precipitated with pemigatinib-induced hyperphosphatemia, they continued to worsen in the absence of pemigatinib or an elevated calcium-phosphate product until disease-modifying therapy was initiated. To rationalize this mechanistically, one can examine the relationship of FGF signaling to calcification and vascular biology.
While the pathogenesis of calciphylaxis is unclear, there is a plethora of in-vitro data outlining a common pathway. Risk factors (e.g., hypercalcemia, hyperphosphatemia) initially stimulate vascular smooth muscle cells (VSMCs) to transdifferentiate from a contractile phenotype to an osteoblast-like state, upregulating transcription factors important in bone formation (e.g., RUNX2) (12). Once transdifferentiated, they produce matrix vesicles filled with calcium and phosphate that precipitate calcium hydroxyapatite deposits into the tunica media of dermal-hypodermal arterioles. The driving force behind the proliferation of these transdifferentiated cells depends on the balance of osteogenic promoters and inhibitors.
BMP2 and BMP4—two key promoters—utilize the TGF-β (i.e., SMAD) pathway to upregulate NF-κB and subsequently RUNX2 levels, establishing a feed-forward growth loop (12). These promoters are upregulated by VEGFA from nearby affected adipocytes, and negatively regulated by FGF2-FGFR2 mediated inhibition of Smad signaling. FGF2 also induces production of matrix Gla protein (MGP), a vitamin K-activated gene heavily involved in mineralization and a potent inhibitor of transdifferentiation and matrix vesicle release. Moreover, BMP4 requires reactive oxygen species (ROS) for activity, mediating vascular calcification through the p38 MAPK pathway. FGFR blockade causes intracellular ROS build-up, secondary to degradation of c-myc. Inhibiting the FGF23-Klotho-FGR1 phosphate regulation pathway also leads to hyperphosphatemia, directly and indirectly mediating tissue mineralization (13). Normally, FGF23 prevents hyperphosphatemia by inhibiting enzymatic activation of vitamin D, and separately inhibiting FGF1/Klotho complexes in the proximal renal tubule linked to renal phosphate reuptake transporters. In a severe combined immunodeficient (SCID)-mice tumor xenograft model, pan-FGFR inhibition led to high serum phosphate levels, with subsequent soft tissue mineralization throughout the great vessels, kidney, myocardium, and gastric tissues (13). FGF23 knockout mice demonstrate widespread vessel calcification and soft tissue calcification, while in vitro knockout of klotho in VSMCs recapitulates the osteogenic RUNX2-mediated transdifferentiation and subsequent vascular calcification seen when exposing VSMCs to high phosphate levels (14).
Thus, FGF pathway inhibition mediates calciphylaxis through two avenues: facilitating high calcium-phosphate product that directly and indirectly powers a feed-forward loop of osteogenic VSMC transdifferentiation that fuels vascular and tissue calcification. Multidisciplinary management, depicted in Figure 4, can ameliorate both avenues. Risk factor mitigation (e.g., phosphate binders, medication reconciliation) breaks the stimulus. STS has multiple roles as a disease modifying therapy. STS chelates calcium from precipitated minerals into excretable calcium thiosulfate. Moreover, it depletes ROS buildup and inhibits adipocyte induced increase of VSMC ability in a dose-dependent manner (15). In contrast, Diltiazem inhibits calcium influx into VSMCs and damaged tissues while vitamin K maintains MGP activation (16). A phase 3 clinical trial is underway for phytic acid (SNF472, NCT04195906), which binds to forming/formed calcium hydroxyapatite crystals and physically disrupts crystal growth.
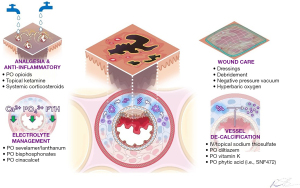
Conclusions
In summary, FGF signaling inhibition by pemigatinib or other FGFRi can be connected to key biochemical events related to calciphylaxis pathophysiology. Our patient’s clinical and histological findings both align with experiences from other reported cases with FGFRi-induced calciphylaxis or other uremic or non-uremic etiologies. Key to our patient’s successful management was efficient multidisciplinary teamwork (a coordinated effort including medical oncology, nephrology, pathology, dermatology). Importantly, the use of disease modifying therapies including STS and diltiazem along with aggressive pain and wound care improved patient symptoms and thereby quality of life. This is the only case of pemigatinib related calciphylaxis outside of the clinical trial setting providing a framework for management. This report takes a deeper dive into the molecular mechanisms of this adverse effect and gives readers an example on how to care for and manage patients with this condition.
Acknowledgments
We acknowledge the patient and his family, staff at the cancer center.
Funding: None.
Footnote
Reporting Checklist: The authors have completed the CARE reporting checklist. Available at https://jgo.amegroups.com/article/view/10.21037/jgo-23-139/rc
Peer Review File: Available at https://jgo.amegroups.com/article/view/10.21037/jgo-23-139/prf
Conflicts of Interest: All authors have completed the ICMJE uniform disclosure form (Available at https://jgo.amegroups.com/article/view/10.21037/jgo-23-139/coif). The authors have no conflicts of interest to declare.
Ethical Statement: The authors are accountable for all aspects of the work in ensuring that questions related to the accuracy or integrity of any part of the work are appropriately investigated and resolved. All procedures performed in this study were in accordance with the ethical standards of the institutional and/or national research committee(s) and with the Helsinki Declaration (as revised in 2013). Since the patient was deceased, written informed consent was obtained from the patient’s wife for the publication of this case report and accompanying images. A copy of the written consent is available for review by the editorial office of this journal.
Open Access Statement: This is an Open Access article distributed in accordance with the Creative Commons Attribution-NonCommercial-NoDerivs 4.0 International License (CC BY-NC-ND 4.0), which permits the non-commercial replication and distribution of the article with the strict proviso that no changes or edits are made and the original work is properly cited (including links to both the formal publication through the relevant DOI and the license). See: https://creativecommons.org/licenses/by-nc-nd/4.0/.
References
- Chandana SR, Babiker HM, Mahadevan D. Clinical complexity of utilizing FGFR inhibitors in cancer therapeutics. Expert Opin Investig Drugs 2020;29:1413-29. [Crossref] [PubMed]
- Abou-Alfa GK, Sahai V, Hollebecque A, et al. Pemigatinib for previously treated, locally advanced or metastatic cholangiocarcinoma: a multicentre, open-label, phase 2 study. Lancet Oncol 2020;21:671-84. [Crossref] [PubMed]
- Lacouture ME, Sibaud V, Anadkat MJ, et al. Dermatologic Adverse Events Associated with Selective Fibroblast Growth Factor Receptor Inhibitors: Overview, Prevention, and Management Guidelines. Oncologist 2021;26:e316-26. [Crossref] [PubMed]
- Storandt MH, Kurniali PC, Mahipal A, et al. Targeted Therapies in Advanced Cholangiocarcinoma. Life (Basel) 2023;13:2066. [Crossref] [PubMed]
- Qurashi M, Vithayathil M, Khan SA. Epidemiology of cholangiocarcinoma. Eur J Surg Oncol 2023; Epub ahead of print. [Crossref] [PubMed]
- Surya H, Abdullah M, Nelwan EJ, et al. Current Updates on Diagnosis and Management of Cholangiocarcinoma: from Surgery to Targeted Therapy. Acta Med Indones 2023;55:361-70. [PubMed]
- Rizzo A, Brandi G. First-line Chemotherapy in Advanced Biliary Tract Cancer Ten Years After the ABC-02 Trial: "And Yet It Moves!". Cancer Treat Res Commun 2021;27:100335. [Crossref] [PubMed]
- Griffith P, Jedrych J, Sunshine J, et al. Calciphylaxis Cutis Associated With Fibroblast Growth Factor Receptor (FGFR) Inhibitor Therapy: A New Challenge. Cureus 2022;14:e21478. [Crossref] [PubMed]
- Qian J, Li JN, Rose EK, et al. Fibroblast growth factor receptor inhibitor therapy induced calcinosis cutis treated with sodium thiosulfate. JAAD Case Rep 2022;31:128-32. [Crossref] [PubMed]
- Bibeau K, Féliz L, Lihou CF, et al. Progression-Free Survival in Patients With Cholangiocarcinoma With or Without FGF/FGFR Alterations: A FIGHT-202 Post Hoc Analysis of Prior Systemic Therapy Response. JCO Precis Oncol 2022;6:e2100414. [Crossref] [PubMed]
- Kodumudi V, Jeha GM, Mydlo N, et al. Management of Cutaneous Calciphylaxis. Adv Ther 2020;37:4797-807. [Crossref] [PubMed]
- Gomes F, La Feria P, Costa C, et al. Non-Uremic Calciphylaxis: A Rare Diagnosis with Limited Therapeutic Strategies. Eur J Case Rep Intern Med 2018;5:000986. [Crossref] [PubMed]
- Dayanand P, Sandhyavenu H, Dayanand S, et al. Role of Bisphosphonates in Vascular calcification and Bone Metabolism: A Clinical Summary. Curr Cardiol Rev 2018;14:192-9. [Crossref] [PubMed]
- Courbon G, Martinez-Calle M, David V. Simultaneous management of disordered phosphate and iron homeostasis to correct fibroblast growth factor 23 and associated outcomes in chronic kidney disease. Curr Opin Nephrol Hypertens 2020;29:359-66. [Crossref] [PubMed]
- Yanochko GM, Vitsky A, Heyen JR, et al. Pan-FGFR inhibition leads to blockade of FGF23 signaling, soft tissue mineralization, and cardiovascular dysfunction. Toxicol Sci 2013;135:451-64. [Crossref] [PubMed]
- Lim K, Lu TS, Molostvov G, et al. Vascular Klotho deficiency potentiates the development of human artery calcification and mediates resistance to fibroblast growth factor 23. Circulation 2012;125:2243-55. [Crossref] [PubMed]