Neuroinflammation in the paraventricular nucleus of the hypothalamus precipitates visceral pain induced by pancreatic cancer in mice
Highlight box
Key findings
• Targeted modulation of microglia and astrocytes in the paraventricular nucleus of the hypothalamus (PVN) ameliorates pancreatic cancer-induced visceral pain.
What is known and what is new?
• Neuroinflammation was involved in the regulation of pain.
• Neuroinflammation in the PVN precipitates visceral pain induced by pancreatic cancer in mice.
What is the implication, and what should change now?
• Targeting glia may be a potential approach for alleviating visceral pain in patients.
Introduction
Visceral pain is a significant form of chronic pain. According to statistics, the prevalence of visceral pain varies from 9% to 23% in different regions and countries worldwide (1). Pancreatic cancer-induced chronic visceral pain is also commonly observed in clinical settings, of which abdominal pain is a frequent symptom among patients (2). In China, the incidence of pancreatic cancer-induced visceral pain (PCVP) is as high as 68% (3), and advanced stages are associated with an incidence rate of up to 90% (4,5). Pancreatic cancer is an aggressive malignancy with a poor prognosis and is the eleventh most common cancer globally (6). In China alone, there were approximately 90,000 new cases of pancreatic cancer in 2015, ranking ninth worldwide (7). The substantial economic burden imposed on society and families by PCVP significantly affects individuals’ quality of life. However, the central mechanism underlying this type of visceral pain remains unclear, which has led to a lack of effective treatment options. Therefore, urgent research needs to be conducted on the neural mechanism responsible for PCVP.
Neuroinflammation in the central nervous system (CNS) primarily manifests through the activation of various glial cells, particularly microglia and astrocytes, which facilitate the release of inflammatory mediators leading to chronic pain (8-10). The close proximity between glia (astrocytes and microglia) neurons facilitates glial activation via neurotransmission, as glia express a diverse array of functional neurotransmitter receptors (11). These include ionotropic non-NMDA and NMDA receptors, as well as metabotropic glutamate (mGluR3 and mGluR5), purinergic, and substance P receptors. Upon activation of glia, signaling pathways such as mitogen-activated protein kinase 1 (MAPK1) and MAPK8 are initiated, leading to an upregulation in the synthesis of inflammatory factors including interleukin 1β (IL-1β), IL-6, tumor necrosis factor-alpha (TNF-α), prostaglandin E2 (PGE2), and nitric oxide (NO). Consequently, this cascade further contributes to the initiation and maintenance of chronic pain (12,13). The eliminator PLX5622 (C21H19F2N5O) and the inhibitor minocycline have been successfully used to target microglia to inhibit central sensitization to relieve chronic pain in animals (8,14-16). Additionally, studies have also explored emerging chemogenetic methods to inhibit astrocytes and thus regulate central inflammation (17-19). However, it is not yet known whether targeting microglia and astrocytes effectively alleviates PCVP.
Therefore, in this study, we sought to investigate the involvement of microglial cells and astrocytes in the regulation of PCVP in the paraventricular nucleus of the hypothalamus (PVN), a crucial brain region known for its role in regulating visceral pain. We comprehensively employed an animal model of PCVP, chemogenetics, and the inhibitor minocycline in this study. We observed an increase in both the number of microglia and astrocytes. Injections of the microglial inhibitor minocycline into the PVN alleviated visceral pain. Similarly, the chemogenetic inhibition of astrocytes alleviated visceral pain. These findings contribute to the advancement and refinement of the regulatory theory concerning PCVP and offer novel insights for identifying effective targets in treating PCVP. We present this article in accordance with the ARRIVE reporting checklist (available at https://jgo.amegroups.com/article/view/10.21037/jgo-24-42/rc).
Methods
Animals
Six-week-old male C57B/6N mice were purchased from the Experimental Animal Center of Xuzhou Medical University (Xuzhou, China). The mice were placed in a constant temperature and humidity environment with a 12-hour light/dark cycle with free access to water and food. All the experimental procedures were approved by the Animal Care and Use Committee of Shanghai Ninth People’s Hospital (No. SH9H-2023-A223-SB), in compliance with the national guidelines for the care and use of animals. A protocol was prepared before the study without registration.
Mouse model of PCVP
The primary tumor luciferase cell line (mPAKPC-luc) developed from the KPC (Pdx1-cre/LSL-Kras G12D/P53 R172H) mouse model (purchased from Gempharmatech, Nanjing, China) were resuscitated and cultured. Next, mPAKPC-luc cells at the logarithmic growth stage (the third to fourth generation after resuscitation) were collected. The culture medium was removed, and the cells were washed twice with Dulbecco’s Phosphate Buffered Saline, and then inoculated (the cell survival rate was measured before and after tumor implantation). C57BL/6 male mice were injected with mPAKPC-luc cells suspended in 100 µL of mixed medium [Matrigel: Phosphate Buffered Saline (PBS) =1:1] at the head of the pancreas with a sterile insulin needle to build an orthotopic tumor model. The sham groups were injected with the same volume of medium as a control. Seven days after incubation, the orthotopic tumor burdens were measured using an in vivo imaging system. Nociceptive testing was performed on days 12, 15, and 18 after incubation. When the mice in the experimental process met the following welfare criteria, euthanasia was conducted based on animal welfare standards, using excessive inhalation of 95% carbon dioxide to induce death: (I) persistent diarrhea; (II) sluggishness (inability to eat or drink); (III) hunched back and lying on side; (IV) reduced activity and symptoms of muscle atrophy; (V) difficulty breathing; (VI) a progressive decrease in body temperature; (VII) paralysis and convulsions; (VIII) continuous bleeding; (IX) an inability to move normally due to large tumors or for other reasons; (X) an inability to move normally due to severe ascites or increased abdominal circumference.
Behavioral analysis
Visceral pain was assessed based on the abdominal mechanical hyperalgesia test and hunch behavior scores. In all cases, the male mice were randomly and blindly separated into each group using the random number table method in this study, and the observations were made by two independent observers blinded to the experimental status of each mouse.
Abdominal mechanical hyperalgesia test
The behavioral analyses were performed as described previously with some modifications (20). Von Frey fiber (0.16 g) was vertically applied to the left upper abdomen for about 2 seconds, and this was repeated 10 times with a 5 min interval between each stimulation. A positive response was defined by the presence of the following behaviors: lifting, scratching, licking the abdomen, moving, or jumping immediately. The response rate was calculated as follows: response rate = number of positive response /10 trials.
Hunch scores
The hunch score was used to assess spontaneous visceral pain and was determined following previously described methods with some modifications (21). The scoring criteria for hunch behavior were as follows: 0—an absence of round-back posture, exploratory behavior, and normal coat appearance; 1—mild round-back posture, exploratory behavior, and a normal coat appearance; 2—severe round-back posture, accompanied by a slight reduction in exploratory behavior, slight piloerection, and intermittent abdominal contractions; 3—severe round-back posture, marked by significantly reduced exploratory behavior, moderate piloerection, and intermittent abdominal contractions; and 4—severe round-back posture, accompanied by little or no exploratory behavior, full-body piloerection, and immobility of the head. The mice were observed for a period of 300 s, and the average hunch score was calculated.
Immunofluorescence
The mice were deeply anesthetized with pentobarbital sodium [50 mg/kg, intraperitoneal (i.p.)], followed by a cardiac infusion of 20 mL of normal saline and 20 mL of 4% [weight/volume (w/v)] paraformaldehyde. The brain was then taken out and fixed overnight in 4% paraformaldehyde at 4 ℃. After brain preservation with 30% (w/v) sucrose, the 30-micron coronal section was sliced using a cryostat microtome for the purpose of immunofluorescence detection. The sections were subjected to five washes, followed by infiltration with 0.3% Triton-X-100 for 1 hour and subsequent sealing in a solution of PBS containing 5% normal goat serum and 2% bovine serum albumin for 2 hours. The brain sections were then incubated with anti-antigen-like family member b (CD11b) (rabbit, 1:500, #17800, Cell Signaling Technology, Boston, USA) and anti- glial fibrillary acidic protein (GFAP) (rabbit, 1:500, #80788, Cell Signaling Technology, Boston, USA) at 4 ℃ overnight. The sections were incubated with Alexa Fluor 594 donkey anti-rabbit (1:500, #A32754, Thermo Fisher Scientific, Waltham, USA) at room temperature for 2 hours. The images were acquired using a confocal laser microscope (LSM80, Zeiss, Germany) equipped with a 10× lens. For the cell quantification, three sections surrounding the PVN were manually counted using NIH ImageJ software (National Institutes of Health, Bethesda, USA). The sections had consistent coordinates across all the experimental groups.
Brain stereotactic injection of AAV and drug
The mice were deeply anesthetized with isoflurane and secured in a stereotaxic apparatus (RWD, Shenzhen, China). Following a skin incision to expose the skull, the coordinates of the PVN region were targeted using Paxinos and Franklin’s Atlas as the reference: (anteroposterior) 0.94 mm, (mediolateral) ±0.20 mm, and (dorsoventral) 5.10 mm from bregma. Micro syringes (Gaoge, Shanghai, China) were used for the precise microinjections of viruses and drugs before suturing the surgical incisions. Subsequently, the mice were returned to their cages after regaining consciousness.
For the chemogenetic manipulation, adenovirus-associated vector (AAV) 2/5-GfaABC1D-iβARK-mCherry (3.5×1012 VG/mL, 0.1 µL, BrainVTA, Wuhan, China) and AAV2/5-GfaABC1D-mCherry (3.1×1012 VG/mL, 0.1 µL, BrainVTA, Wuhan, China) were used to inhibit astrocytes (day 1). To examine the function of astrocytes, mice were intraperitoneally injected with 0.33 mg/mL of clozapine N-oxide (CNO) (0.2 mL/20 g, BrainVTA, Wuhan, China) from day 8 to day 21 and subjected to behavioral tests on days 14 and 21. After conducting the behavioral tests, brain slices were obtained for examination using a fluorescence microscope to confirm the accuracy of the virus injection site localization.
The microglia were modulated by an intracranial injection of minocycline (10 µg/µL, Sigma USA, 0.1 µL) or PBS (0.01M, Sigma USA, 0.1 µL) into the PVN of the mice prior to the behavioral assessments at both 30 and 60 min post-administration.
Statistical analysis
The data are presented as the mean ± standard deviation (SD). The statistical analyses were performed using GraphPad Prism 5.0 (GraphPad Software, San Diego, CA, USA). The two-sample t-test was used to compare the response rate. The Wilcoxon rank-sum test was employed to compare the hunch scores. A significance level of P<0.05 was considered statistically significant.
Results
Establishment of a PCVP mouse model
Visceral pain was assessed using the abdominal mechanical hyperalgesia test and the hunch score on days 12, 15, and 18 following injection of the mPAKPC-luc cells (Figure 1A). Significant differences were observed in both the abdominal mechanical hyperalgesia test results and the hunch scores on days 12 (Figure 1B,1C), 15 (Figure 1D,1E), and 18 (Figure 1F,1G) after the pancreatic injection of the mPAKPC-luc cells in the mice. Moreover, these differences became more pronounced over time. These behavioral findings confirm the successful establishment of a mouse model for PCVP.
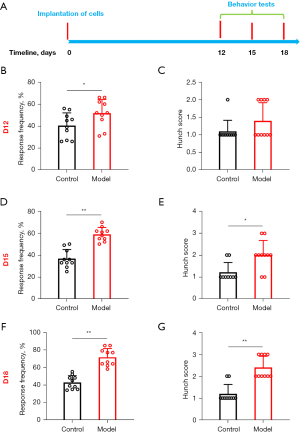
The number of both microglia and astrocytes in the PVN was increased in the PCVP mice
The immunofluorescence results showed that the number of PVN microglia marker CD11b cells in the PCVP mice was significantly increased (Figure 2). Similarly, the number of the PVN astrocyte marker GFAP was significantly increased (Figure 2). These results indicate that PVN microglia and astrocytes are involved in PCVP.
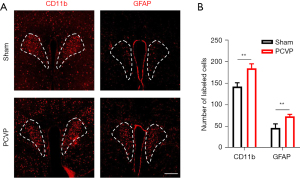
The microglia inhibitor minocycline relieved PCVP
We subsequently targeted microglia in an attempt to alleviate visceral pain. The PCVP mice were microinjected with the microglia inhibitor minocycline in the PVN (Figure 3A). Minocycline administration significantly downregulated both Von Frey responses and hunch scores at 30 (Figure 3B,3C) and 60 min (Figure 3D,3E) after the microinjection of minocycline into the PVN. Thus, these results suggest that targeting microglia relieves PCVP.
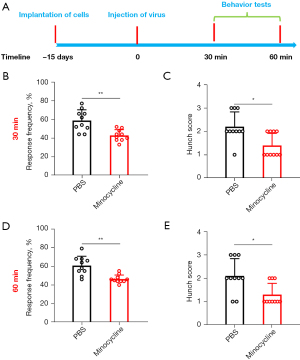
Chemogenetic inhibition of PVN astrocytes alleviated PCVP
To confirm the critical role of PVN astrocytes in the regulation of PCVP, we used chemogenetics to specifically inhibit PVN astrocytes in the PCVP mice (Figure 4A). Fluorescence images of the virus clarified the accuracy of the virus injection site (Figure 4B,4C). CNO was injected at 8 days after virus injection for 2 weeks. Based on the responses to the Von Frey fibers and hunch scores, visceral pain was elevated at days 14 and 21 after the virus injection. The responses to the Von Frey fibers and hunch scores were significantly reduced at days 14 (Figure 4D,4E) and 21 (Figure 4F,4G) after the virus injection. The behavioral results showed that the specific inhibition of PVN astrocytes alleviated PCVP.
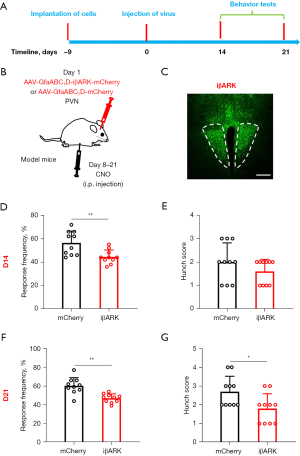
Discussion
In this study, we found that PVN microglia and astrocytes were activated in PCVP mice. Meanwhile, PVN injections of the microglia inhibitor minocycline relieved PCVP. The chemogenetic inhibition of astrocytes also relieved visceral pain. In conclusion, this study showed that PVN microglia and astrocytes modulated PCVP, which may provide a potential approach for alleviating visceral pain in patients.
As previous studies have shown, neuroinflammation in the CNS plays a crucial role in the pathogenesis of chronic pain (22,23). Specifically, microglia, immune cells residing in the CNS, are pivotal in driving central sensitization and neuropathic pain development (24-26). In pathological conditions such as these, activated microglia release proinflammatory cytokines, such as IL-1β and TNF-α, that augment pain perception by increasing neuronal excitability to facilitate synaptic transmission (27,28). Microglia were recently identified as mediators of central sensitization in a rat model of narcotic bowel-like syndrome (29). Some animal studies have successfully targeted microglia using the eliminator PLX5622 and the inhibitor minocycline to inhibit central inflammatory responses to treat CNS diseases, including chronic pain (14-16). For example, our previous study found that the injection of the microglia inhibitor minocycline into the PVN alleviated irritable bowel syndrome (IBS)-like visceral pain (8). Similarly, in this study, we found that PCVP was alleviated by the microinjection of minocycline to inhibit PVN microglia, which provides further evidence that microglia are effective targets for chronic pain treatment. In addition, studies have shown that microglia activate astrocytes, and astrocytes have a slower but longer effect on CNS disorders (30-32). Research has shown that the chemogenetic inhibition of astrocytes can be used to treat CNS inflammatory-related diseases, including pain (33-36). Therefore, we hypothesize that targeting astrocytes may confer analgesic effects. In line with this notion, the specific pharmacological inhibition of astrocytes in this study significantly ameliorated pain symptoms. Therefore, we believe that glial cells could be an effective potential target for the treatment of various types of pain, including pancreatic cancer.
However, it is crucial to acknowledge that certain issues need to be addressed. As is well known, microglia can be classified into many different types that mediate various functions. However, minocycline acts non-selectively on the entire microglial population, which may lead to numerous side effects. Similarly, the chemogenetic regulation of the overall astrocyte population may not only alleviate pain but may also bring about a multitude of adverse reactions. Researchers have identified two distinct subtypes of reactive astrocytes, namely A1-reactive and A2-reactive astrocytes. Neuroinflammation induces the activation of A1 astrocytes, which can potentially secrete neurotoxins leading to rapid neuronal death. Reversely, ischemia triggers the activation of A2 astrocytes, contributing to more efficient neuronal preservation. The dominant role played by A1-reactive astrocytes in chronic pain development is widely acknowledged; hence extensive research efforts have been dedicated to unraveling their involvement in this specific field (37). Therefore, future research should focus on identifying additional brain regions involved in neuroinflammation and use single-cell sequencing technology to identify specific glial cell subgroups implicated in pain progression to enable more precise regulation rather than indiscriminate modulation.
Conclusions
The present study demonstrated that the administration of minocycline, an inhibitor, in combination with the chemogenetic inhibition of astrocytes, effectively ameliorated visceral pain. These findings contribute to the advancement of the regulatory theory on PCVP and provide novel insights for identifying potential therapeutic targets in pancreatic diseases associated with visceral pain.
Acknowledgments
Funding: This study was supported by
Footnote
Reporting Checklist: The authors have completed the ARRIVE reporting checklist. Available at https://jgo.amegroups.com/article/view/10.21037/jgo-24-42/rc
Data Sharing Statement: Available at https://jgo.amegroups.com/article/view/10.21037/jgo-24-42/dss
Peer Review File: Available at https://jgo.amegroups.com/article/view/10.21037/jgo-24-42/prf
Conflicts of Interest: All authors have completed the ICMJE uniform disclosure form (available at https://jgo.amegroups.com/article/view/10.21037/jgo-24-42/coif). The authors have no conflicts of interest to declare.
Ethical Statement: The authors are accountable for all aspects of the work in ensuring that questions related to the accuracy or integrity of any part of the work are appropriately investigated and resolved. All the experimental procedures were approved by the Animal Care and Use Committee of Shanghai Ninth People’s Hospital (No. SH9H-2023-A223-SB), in compliance with the national guidelines for the care and use of animals.
Open Access Statement: This is an Open Access article distributed in accordance with the Creative Commons Attribution-NonCommercial-NoDerivs 4.0 International License (CC BY-NC-ND 4.0), which permits the non-commercial replication and distribution of the article with the strict proviso that no changes or edits are made and the original work is properly cited (including links to both the formal publication through the relevant DOI and the license). See: https://creativecommons.org/licenses/by-nc-nd/4.0/.
References
- Basso L, Benamar M, Mas-Orea X, et al. Endogenous control of inflammatory visceral pain by T cell-derived opioids in IL-10-deficient mice. Neurogastroenterol Motil 2020;32:e13743. [Crossref] [PubMed]
- Ilic M, Ilic I. Epidemiology of pancreatic cancer. World J Gastroenterol 2016;22:9694-705. [Crossref] [PubMed]
- Dobosz Ł, Kaczor M, Stefaniak TJ. Pain in pancreatic cancer: review of medical and surgical remedies. ANZ J Surg 2016;86:756-61. [Crossref] [PubMed]
- Mercadante S, Adile C, Giarratano A, et al. Breakthrough pain in patients with abdominal cancer pain. Clin J Pain 2014;30:510-4. [Crossref] [PubMed]
- Lindsay TH, Jonas BM, Sevcik MA, et al. Pancreatic cancer pain and its correlation with changes in tumor vasculature, macrophage infiltration, neuronal innervation, body weight and disease progression. Pain 2005;119:233-46. [Crossref] [PubMed]
- Carvajal G. Pancreatic Cancer Related Pain: Review of Pathophysiology and Intrathecal Drug Delivery Systems for Pain Management. Pain Physician 2021;24:E583-94. [PubMed]
- Chen W, Zheng R, Baade PD, et al. Cancer statistics in China, 2015. CA Cancer J Clin 2016;66:115-32. [Crossref] [PubMed]
- Ji NN, Meng QX, Wang Y, et al. Microglia-derived TNF-α inhibiting GABAergic neurons in the anterior lateral bed nucleus of the stria terminalis precipitates visceral hypersensitivity induced by colorectal distension in rats. Neurobiol Stress 2022;18:100449. [Crossref] [PubMed]
- Yuan T, Orock A, Greenwood-Van Meerveld B. Amygdala microglia modify neuronal plasticity via complement C1q/C3-CR3 signaling and contribute to visceral pain in a rat model. Am J Physiol Gastrointest Liver Physiol 2021;320:G1081-92. [Crossref] [PubMed]
- Yuan T, Manohar K, Latorre R, et al. Inhibition of Microglial Activation in the Amygdala Reverses Stress-Induced Abdominal Pain in the Male Rat. Cell Mol Gastroenterol Hepatol 2020;10:527-43. [Crossref] [PubMed]
- Porter JT, McCarthy KD. Astrocytic neurotransmitter receptors in situ and in vivo. Prog Neurobiol 1997;51:439-55. [Crossref] [PubMed]
- Milligan ED, Watkins LR. Pathological and protective roles of glia in chronic pain. Nat Rev Neurosci 2009;10:23-36. [Crossref] [PubMed]
- Parpura V, Basarsky TA, Liu F, et al. Glutamate-mediated astrocyte-neuron signalling. Nature 1994;369:744-7. [Crossref] [PubMed]
- Lu X, Chen L, Jiang C, et al. Microglia and macrophages contribute to the development and maintenance of sciatica in lumbar disc herniation. Pain 2023;164:362-74. [Crossref] [PubMed]
- Xu Z, Qin Z, Zhang J, et al. Microglia-mediated chronic psoriatic itch induced by imiquimod. Mol Pain 2020;16:1744806920934998. [Crossref] [PubMed]
- Sawicki CM, Kim JK, Weber MD, et al. Microglia Promote Increased Pain Behavior through Enhanced Inflammation in the Spinal Cord during Repeated Social Defeat Stress. J Neurosci 2019;39:1139-49. [Crossref] [PubMed]
- Kim JH, Rahman MH, Lee WH, et al. Chemogenetic stimulation of the G(i) pathway in astrocytes suppresses neuroinflammation. Pharmacol Res Perspect 2021;9:e00822. [Crossref] [PubMed]
- Bossuyt J, Van Den Herrewegen Y, Nestor L, et al. Chemogenetic modulation of astrocytes and microglia: State-of-the-art and implications in neuroscience. Glia 2023;71:2071-95. [Crossref] [PubMed]
- Lu JS, Yang L, Chen J, et al. Basolateral amygdala astrocytes modulate diabetic neuropathic pain and may be a potential therapeutic target for koumine. Br J Pharmacol 2023;180:1408-28. [Crossref] [PubMed]
- Selvaraj D, Hirth M, Gandla J, et al. A mouse model for pain and neuroplastic changes associated with pancreatic ductal adenocarcinoma. Pain 2017;158:1609-21. [Crossref] [PubMed]
- Sevcik MA, Jonas BM, Lindsay TH, et al. Endogenous opioids inhibit early-stage pancreatic pain in a mouse model of pancreatic cancer. Gastroenterology 2006;131:900-10. [Crossref] [PubMed]
- Ji RR, Chamessian A, Zhang YQ. Pain regulation by non-neuronal cells and inflammation. Science 2016;354:572-7. [Crossref] [PubMed]
- Ren K, Dubner R. Interactions between the immune and nervous systems in pain. Nat Med 2010;16:1267-76. [Crossref] [PubMed]
- Inoue K, Tsuda M. Microglia in neuropathic pain: cellular and molecular mechanisms and therapeutic potential. Nat Rev Neurosci 2018;19:138-52. [Crossref] [PubMed]
- Ji RR, Suter MR. p38 MAPK, microglial signaling, and neuropathic pain. Mol Pain 2007;3:33. [Crossref] [PubMed]
- Cui Y, Hu C, Niu C, et al. Electroacupuncture attenuates spared nerve injury-induced neuropathic pain possibly by promoting the progression of AMPK/mTOR-mediated autophagy in spinal microglia. Ann Transl Med 2022;10:1278. [Crossref] [PubMed]
- Old EA, Malcangio M. Chemokine mediated neuron-glia communication and aberrant signalling in neuropathic pain states. Curr Opin Pharmacol 2012;12:67-73. [Crossref] [PubMed]
- Burke NN, Fan CY, Trang T. Microglia in health and pain: impact of noxious early life events. Exp Physiol 2016;101:1003-21. [Crossref] [PubMed]
- Agostini S, Eutamene H, Cartier C, et al. Evidence of central and peripheral sensitization in a rat model of narcotic bowel-like syndrome. Gastroenterology 2010;139:553-63, 563.e1-5.
- Clark IC, Gutiérrez-Vázquez C, Wheeler MA, et al. Barcoded viral tracing of single-cell interactions in central nervous system inflammation. Science 2021;372:eabf1230. [Crossref] [PubMed]
- Sacristán C. Microglia and Astrocyte Crosstalk in Immunity. Trends Immunol 2020;41:747-8. [Crossref] [PubMed]
- Liddelow SA, Marsh SE, Stevens B. Microglia and Astrocytes in Disease: Dynamic Duo or Partners in Crime? Trends Immunol 2020;41:820-35. [Crossref] [PubMed]
- Xu Q, Ford NC, He S, et al. Astrocytes contribute to pain gating in the spinal cord. Sci Adv 2021;7:eabi6287. [Crossref] [PubMed]
- Takeda I, Yoshihara K, Cheung DL, et al. Controlled activation of cortical astrocytes modulates neuropathic pain-like behaviour. Nat Commun 2022;13:4100. [Crossref] [PubMed]
- Kohro Y, Matsuda T, Yoshihara K, et al. Spinal astrocytes in superficial laminae gate brainstem descending control of mechanosensory hypersensitivity. Nat Neurosci 2020;23:1376-87. [Crossref] [PubMed]
- Lu JS, Yang L, Chen J, et al. Basolateral amygdala astrocytes modulate diabetic neuropathic pain and may be a potential therapeutic target for koumine. Br J Pharmacol 2023;180:1408-28. [Crossref] [PubMed]
- Liddelow SA, Guttenplan KA, Clarke LE, et al. Neurotoxic reactive astrocytes are induced by activated microglia. Nature 2017;541:481-7. [Crossref] [PubMed]