Association between type 2 diabetes, alcohol intake frequency, age at menarche, and gallbladder cancer: a two-sample Mendelian randomization study
Highlight box
Key findings
• Type 2 diabetes (T2D) emerged as a potentially protective factor against gallbladder cancer (GBC), whereas neither alcohol intake frequency (AIF) nor age at menarche (AAM) demonstrated a causal relationship with GBC risk.
What is known and what is new?
• The relationship between T2D, AIF, AAM, and GBC remains unclear.
• T2D was found to be a protective factor against GBC, while AIF and AAM demonstrated no relationship with GBC.
What is the implication, and what should change now?
• This work provides epidemiological evidence supporting the association between T2D, AIF, AAM, and GBC.
Introduction
Gallbladder cancer (GBC) is the predominant malignancy of the biliary tract and is notorious for its exceptionally poor survival prognosis (1-4). Often diagnosed at advanced stages due to its deep anatomical positioning and nonspecific symptoms, numerous patients with GBC miss the opportunity for potentially curative surgical interventions (5-8). With the deepened understanding of GBC, various risk factors including chronic inflammation and biliary tree abnormalities have been identified (1). However, the relationship between type 2 diabetes (T2D) and GBC remains ambiguous. Although some observational studies have suggested that T2D might increase the risk of GBC, confounding factors such as body mass index (BMI) and gender disparities make establishing a clear causal connection challenging (9-12). Indeed, a study has reported evidence refuting a direct causal relationship between T2D and GBC (13). Although alcohol consumption is recognized as a risk factor for various types of cancer (14-17), the link between alcohol intake frequency (AIF) and GBC remains understudied. The higher prevalence of GBC in females is thought to be influenced, in part, by estrogen. This hypothesis is backed by both preclinical research and epidemiological data (18-21). High exogenous estrogen exposure seems to increase the risk of biliary tract cancer (18), pointing to a possible association between age at menarche (AAM) and GBC via its effect on estrogen levels.
Despite previous studies plausibly indicating the presence of physiological connections between T2D, AIF, AAM, and GBC, the possibility of confounders and biases puts into doubt a clear causal link (22-24). Hence, a robust statistical method is strongly needed to verify these associations. Mendelian randomization (MR), which employs single-nucleotide polymorphisms (SNPs) as instrumental variables (IVs) that correlate strongly with the exposure under investigation, may be such a method. MR can be used to establish a causal relationship between exposures and their outcomes (25). The strength of MR stems from the random distribution of alleles during meiosis, rendering MR analyses less susceptible to the effects of unobserved confounders and thus a reliable tool for determining causality (26). In this study, two-sample MR was employed to characterize the causal relationships between T2D, AIF, AAM, and GBC. We present this article in accordance with the STROBE-MR reporting checklist (available at https://jgo.amegroups.com/article/view/10.21037/jgo-24-358/rc).
Methods
Study design and procedure
SNPs associated with T2D, AIF, and AAM were identified as IVs to determine the causal effects of these factors on GBC. The MR analysis in this study strictly adhered to three primary assumptions: (I) the chosen SNPs were strongly associated with the exposures of interest; (II) the IVs were not related to potential confounders; and (III) the genetic variants employed as IVs were not associated with any alternative pathways influencing the outcomes. The overall study framework is depicted in Figure 1, and a summary of the study procedure is presented in Figure 2.
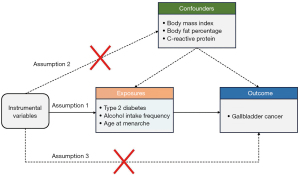
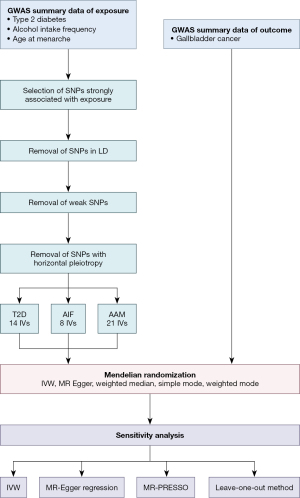
Exposure data and outcome data selection
SNPs associated with exposures (T2D, AIF, and AAM) and the outcome (GBC) were retrieved from the Integrative Epidemiology Unit (IEU) Open Genome-Wide Association Study (GWAS) database (https://gwas.mrcieu.ac.uk/). The data for T2D (GWAS ID: ebi-a-GCST006867) comprised 655,666 Europeans with 5,030,727 SNPs, the data for AIF (GWAS ID: ukb-b-5779) comprised 462,346 Europeans and 9,851,867 SNPs, and the data for AAM (GWAS ID: ukb-b-3768) comprised 243,944 Europeans and the same number of SNPs. For GBC, the data set (GWAS ID: ieu-a-1057) comprised 907 East Asians (pathological results of all cases were registered into Biobank Japan) and 425,707 SNPs. The measurement of all exposure traits is collected uniformly from the UK Biobank (UKB) database and presented in the form of standard deviation (SD) or the logarithmic form of the odds ratio (logOR).
IV selection
To ensure the validity of the MR analysis, a stringent selection process was conducted to identify which IVs met the three assumptions of the MR analysis. This rigorous selection process aimed to obtain representative IVs that could provide reliable estimates of causal effects. We employed a genome-wide significance threshold (P<5×10−8) to identify SNPs with a strong exposure correlation. This stringent threshold ensured the selection of SNPs with robust associations with the exposure variable. Next, we took measures to ensure the independence of the selected SNPs by removing those in linkage disequilibrium (LD) from our analysis. Specifically, we applied a threshold of r2=0.01 within a window of 10,000 kb to exclude SNPs that were in high LD with each other. This approach minimized the potential biases caused by the inclusion of correlated SNPs in the analysis. We then used the F test to assess the strength of IVs, as documented previously (27). Strong genetic variants (F>10) were retained for further analysis. Finally, we applied PhenoScanner (http://www.phenoscanner.medschl.cam.ac.uk/) to avoid horizontal pleiotropy (28). Any pleiotropic SNPs related to the risk factors of GBC, including BMI, body fat percentage, and C-reactive protein, were manually removed (Figure 1) (29).
MR analysis
To assess causality, several statistical methods were employed, including the inverse variance-weighted (IVW) method, weighted median estimate, weighted mode estimate, simple mode estimate, and MR-Egger regression (30). The IVW method was used as the primary estimation method to calculate the aggregated effect of all selected SNPs. This method assumed the absence of pleiotropy and heterogeneity, and it provided an overall estimate of the causal effect by weighting the individual SNP effects based on their inverse variances (30). The weighted median estimate could provide causal estimates consistent with the ultimate effect even in the presence of up to 50% invalid SNPs (31). Weighted-mode estimate and simple-mode estimate were two additional MR methods employed to evaluate the robustness of the results that violated the assumptions of the IVs (32). The effect estimate obtained through MR-Egger regression could provide an estimate of the true causal effect when all IVs were invalid (33).
Sensitivity analysis
MR-Egger regression was used to assess the presence of horizontal pleiotropy (33). For MR-Egger regression, if the vertical intercept was close to zero and statistically nonsignificant (P>0.05), the absence of horizontal pleiotropy was indicated. Scatter plots were used to visualize the results of the horizontal pleiotropy test and to demonstrate the relationship between the IVs and the outcome variable. Additionally, funnel plot analyses were performed to evaluate both the pleiotropy and robustness of the results. We employed the leave-one-out method to identify SNPs with substantial effects on the total causal effects. After sequential removal of each SNP, the pooled effects of the surplus SNPs were computed. To assess heterogeneity, both MR-Egger regression and IVW analysis were conducted. If the P value associated with the Cochran Q statistics was below 0.05, the presence of heterogeneity among the estimates derived from different IVs was indicated. To identify the presence of horizontal pleiotropy and outliers, we employed the Mendelian Randomization Pleiotropy Residual Sum and Outlier (MR-PRESSO) global test and MR-PRESSO outlier test (34).
Statistical analysis
In the presence of significant heterogeneity, a random-effects model was employed; otherwise, a fixed-effects model was used (35). To assess the causality between exposure and outcome, we applied the Bonferroni correction method to set the significance threshold for the P value. This method adjusts the P value threshold, accounting for multiple comparisons and minimizing the likelihood of false-positive results. P<0.016 (0.05/3 exposures) was considered statistically significant. For other tests, the threshold for statistical significance was defined as P<0.05. All MR analyses were conducted using the MR-PRESSO and two-sample MR packages in RStudio (version 4.1.1).
Ethics statement
The study was conducted in accordance with the Declaration of Helsinki (as revised in 2013) (36).
Results
IVs included in the study
For T2D, AIF, and AAM, 14, 8, and 21 IVs were selected, respectively, to assess their relationship with GBC. Table S1 shows the characteristics of the included IVs. The F statistics for these genetic variants exceeded 10, indicating that all IVs were strongly correlated with their corresponding exposures. Additionally, all included IVs affected outcomes through exposures and were not associated with other confounders.
MR estimates of T2D, AIF, and AAM
The MR analysis suggested that T2D has a negative association with GBC [odds ratio (OR) =0.044; 95% confidence interval (CI): 0.004–0.55; P=0.015, IVW; Figure 3], indicating that T2D acts as a protective factor against GBC. This observation was consistent with the findings from the simple mode and weighted mode methods. Although this pattern was not mirrored in the results of the MR-Egger analysis (OR =0.041; 95% CI: 6.17E−07 to 2,692.92; P=0.58) or weighted median method (OR =0.036; 95% CI: 0.0009–1.45; P=0.078), the overarching direction of the pooled effect from these two methods aligned with that of the IVW method.
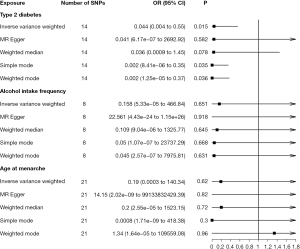
Furthermore, our analysis revealed there to be no causal relationship between AIF and GBC risk (OR =0.158; 95% CI: 5.33E−05 to 466.84; P=0.65, IVW; Figure 3). This nonassociation was uniformly reflected across all four methods employed in this study. Similarly, no significant association was observed between AAM and GBC (OR =0.19; 95% CI: 0.0003–140.34; P=0.62, IVW; Figure 3).
Sensitivity analysis
The causal association between T2D and GBC displayed no signs of horizontal pleiotropy, as evidenced by an intercept close to zero and a P value greater than 0.05 in the MR-Egger regression analysis (Figure 4, Table 1). Neither the IVW method nor the MR-Egger method detected any significant heterogeneity. Furthermore, the MR-PRESSO analysis did not identify any external IVs (Table 1). Funnel plots of T2D and AAM showed a symmetrical distribution (Figure S1). The leave-one-out test revealed that no individual IV exerted a disproportionate influence on the pooled MR estimation (Figure S2).
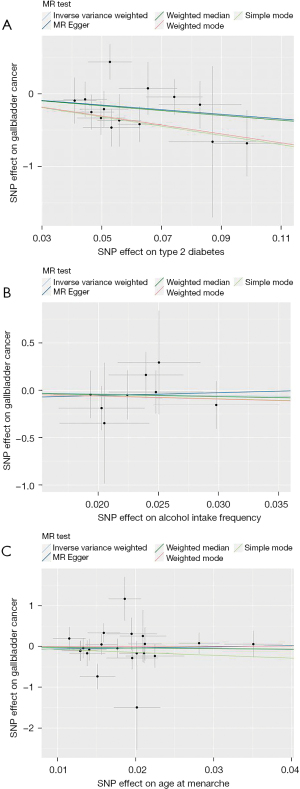
Table 1
Exposure | Pleiotropy | Heterogeneity | MR-PRESSO | ||||||
---|---|---|---|---|---|---|---|---|---|
MR-Egger | Inverse variance-weighted | MR-Egger | |||||||
Intercept | P value | Q | P value | Q | P value | P value | |||
T2D | 0.0045 | 0.99 | 11.76 | 0.55 | 11.76 | 0.47 | 0.55 | ||
AIF | −0.12 | 0.87 | 1.94 | 0.96 | 1.91 | 0.93 | 0.96 | ||
AAM | −0.08 | 0.70 | 19.23 | 0.51 | 19.08 | 0.45 | 0.56 |
MR, Mendelian randomization; T2D, type 2 diabetes; AIF, alcohol intake frequency; AAM, age at menarche; MR-PRESSO, Mendelian Randomization Pleiotropy Residual Sum and Outlier.
Discussion
In this study, our objective was to investigate the causal associations between T2D, AIF, AAM, and GBC—three established risk factors for numerous types of cancers. Our findings indicated an inverse correlation between T2D and the risk of GBC. However, we observed no significant relationship between AIF, AAM, and GBC.
The causal relationship between T2D and GBC has been a controversial issue in prior observational studies. Some have reported an increased risk of GBC to be associated with T2D (10,11,37), while others posit that T2D might be a protective factor against GBC (38). Additionally, one study found no significant association between T2D and GBC (13). These divergent results underscore the lack of understanding in the T2D-GBC relationship. Several factors underpin these inconsistencies: (I) given that BMI is a widely recognized risk factor for both T2D and GBC, any disparity in baseline BMI between study groups introduces a confounding variable that might skew the perceived association; (II) besides BMI, the presence of gallstones, another risk factor for GBC, can also bias the results if not evenly distributed among baseline characteristics; (III) the design limitations of case-control studies make it challenging to deduce causality. The study conducted by Sheng et al. (39) revealed a negative correlation between T2D and GBC but a positive correlation between the homeostasis model assessment of insulin resistance (HOMA-IR) and GBC. Consequently, we hypothesized that insulin resistance, rather than diabetes itself, is the risk factor for GBC. This mechanism can be elucidated at the molecular level. Insulin can stimulate malignant transformation and cancer cell proliferation as indicated in multiple studies (40,41). In insulin-resistant patients, augmented insulin production might drive the oncogenesis of GBC (42). This leads us to speculate that the protective effect of diabetes against GBC may stem from antidiabetic medications such as metformin, which enhance insulin sensitivity (43).
The literature offers conflicting views regarding the link between AIF and GBC. A study reported no significant association (44), while others reported a positive one (23). Although alcohol might prevent gallstone formation by reducing cholesterol levels in the bile (45), it could also boost GBC risk by inducing oxidative stress and DNA damage (23). The relationship between AIF and GBC merits further scrutiny. Our funnel plot’s reliability was hampered by the inclusion of only eight SNPs. However, previous testing has provided evidence ruling out the presence of heterogeneity and horizontal pleiotropy.
Given the higher GBC incidence in females, possibly due to the increased expression of estrogen receptors in GBC cells (46), we were motivated to explore the relationship between AAM and GBC. Several studies have consistently reported that a higher AAM is associated with an increased risk of GBC (24,47,48). It is important to acknowledge BMI as a potential confounder, influencing both puberty onset and the end result. One strength of MR is its capacity to diminish confounding effects through the random allocation of alleles, rendering our findings more robust against BMI’s influence.
Some limitations to this study should be acknowledged. Firstly, ethnic variations between European and East Asian populations might have introduced confounders due to allele frequency. Secondly, there are few IVs strongly related to exposure, and the sample size of GBC in this study suggests that larger samples are essential for more definitive conclusions. Lastly, it is worth noting that certain results exhibited broad CIs due to IV variations. Yet, the heterogeneity test conducted in our study indicated a lack of significant heterogeneity between these variables. The limited SNP count may explain these expansive CIs.
Conclusions
Our study demonstrated that T2D may serve as a protective factor, as it was linked to a reduced risk of GBC. Furthermore, we discerned no causal relationship between either AIF or AAM and GBC.
Acknowledgments
The abstract of this manuscript has been previously published in the 16th World Congress of the International Hepato-Pancreato-Biliary Association, 15–18 May, 2024, Cape Town, South Africa.
We are grateful to Doctor Zheng Zhou from the Department of Endoscopy, Sir Run-Run Shaw Hospital, for her assistance in polishing the manuscript.
Funding: This work was supported by
Footnote
Reporting Checklist: The authors have completed the STROBE-MR reporting checklist. Available at https://jgo.amegroups.com/article/view/10.21037/jgo-24-358/rc
Peer Review File: Available at https://jgo.amegroups.com/article/view/10.21037/jgo-24-358/prf
Conflicts of Interest: The authors have completed the ICMJE uniform disclosure form (available at https://jgo.amegroups.com/article/view/10.21037/jgo-24-358/coif). The authors have no conflicts of interest to declare.
Ethical Statement: The authors are accountable for all aspects of the work in ensuring that questions related to the accuracy or integrity of any part of the work are appropriately investigated and resolved. The study was conducted in accordance with the Declaration of Helsinki (as revised in 2013).
Open Access Statement: This is an Open Access article distributed in accordance with the Creative Commons Attribution-NonCommercial-NoDerivs 4.0 International License (CC BY-NC-ND 4.0), which permits the non-commercial replication and distribution of the article with the strict proviso that no changes or edits are made and the original work is properly cited (including links to both the formal publication through the relevant DOI and the license). See: https://creativecommons.org/licenses/by-nc-nd/4.0/.
References
- Valle JW, Kelley RK, Nervi B, et al. Biliary tract cancer. Lancet 2021;397:428-44. [Crossref] [PubMed]
- Torre LA, Siegel RL, Islami F, et al. Worldwide Burden of and Trends in Mortality From Gallbladder and Other Biliary Tract Cancers. Clin Gastroenterol Hepatol 2018;16:427-37. [Crossref] [PubMed]
- de Savornin Lohman E, Belkouz A, Nuliyalu U, et al. Adjuvant treatment for the elderly patient with resected gallbladder cancer: a SEER-Medicare analysis. J Gastrointest Oncol 2022;13:3227-39. [Crossref] [PubMed]
- Signorelli C, Marrucci E, Cristi E, et al. Colon metastasis from recurrent gallbladder cancer: a case report. AME Case Rep 2021;5:21. [Crossref] [PubMed]
- Roa JC, García P, Kapoor VK, et al. Gallbladder cancer. Nat Rev Dis Primers 2022;8:69. [Crossref] [PubMed]
- Baiu I, Visser B. Gallbladder Cancer. JAMA 2018;320:1294. [Crossref] [PubMed]
- Cao J, Shao H, Hu J, et al. Identification of invasion-metastasis associated MiRNAs in gallbladder cancer by bioinformatics and experimental validation. J Transl Med 2022;20:188. [Crossref] [PubMed]
- Fontana AP, Russolillo N, Errigo D, et al. Role of laparoscopy in the surgical management of gallbladder cancer—a narrative review. Laparosc Surg 2023;7:7. [Crossref]
- Shebl FM, Andreotti G, Rashid A, et al. Diabetes in relation to biliary tract cancer and stones: a population-based study in Shanghai, China. Br J Cancer 2010;103:115-9. [Crossref] [PubMed]
- Alkhayyat M, Abou Saleh M, Qapaja T, et al. Epidemiology of gallbladder cancer in the Unites States: a population-based study. Chin Clin Oncol 2021;10:25. [Crossref] [PubMed]
- Jamal MM, Yoon EJ, Vega KJ, et al. Diabetes mellitus as a risk factor for gastrointestinal cancer among American veterans. World J Gastroenterol 2009;15:5274-8. [Crossref] [PubMed]
- Jain K, Sreenivas V, Velpandian T, et al. Risk factors for gallbladder cancer: a case-control study. Int J Cancer 2013;132:1660-6. [Crossref] [PubMed]
- Malik H, Izwan S, Ng J, et al. Incidence and management of gallbladder cancer in cholecystectomy specimens: a 5-year tertiary centre experience. ANZ J Surg 2023;93:2481-6. [Crossref] [PubMed]
- Driutti M, Dal Maso L, Toffolutti F, et al. Breast cancer deaths attributable to alcohol consumption: Italy, 2015-2019. Breast 2023;71:96-8. [Crossref] [PubMed]
- Amri F, Koulali H, Jabi R, et al. Pancreatic cancer: experience from an emerging country in North Africa. J Cancer Res Clin Oncol 2023;149:14297-302. [Crossref] [PubMed]
- Reggidori N, Bucci L, Santi V, et al. Landscape of alcohol-related hepatocellular carcinoma in the last 15 years highlights the need to expand surveillance programs. JHEP Rep 2023;5:100784. [Crossref] [PubMed]
- Yuan S, Chen J, Ruan X, et al. Smoking, alcohol consumption, and 24 gastrointestinal diseases: Mendelian randomization analysis. Elife 2023;12:e84051. [Crossref] [PubMed]
- Kilander C, Mattsson F, Lu Y, et al. Exogenous estrogen and the risk of biliary tract cancer - a population-based study in a cohort of Swedish men treated for prostate cancer. Acta Oncol 2016;55:846-50. [Crossref] [PubMed]
- Srivastava A, Sharma KL, Srivastava N, et al. Significant role of estrogen and progesterone receptor sequence variants in gallbladder cancer predisposition: a multi-analytical strategy. PLoS One 2012;7:e40162. [Crossref] [PubMed]
- Sumi K, Matsuyama S, Kitajima Y, et al. Loss of estrogen receptor beta expression at cancer front correlates with tumor progression and poor prognosis of gallbladder cancer. Oncol Rep 2004;12:979-84. [PubMed]
- Matsumoto T, Seno H. Updated Trends in Gallbladder and Other Biliary Tract Cancers Worldwide. Clin Gastroenterol Hepatol 2018;16:339-40. [Crossref] [PubMed]
- Gu J, Yan S, Wang B, et al. Type 2 diabetes mellitus and risk of gallbladder cancer: a systematic review and meta-analysis of observational studies. Diabetes Metab Res Rev 2016;32:63-72. [Crossref] [PubMed]
- Park JH, Hong JY, Han K, et al. Light-to-Moderate Alcohol Consumption Increases the Risk of Biliary Tract Cancer in Prediabetes and Diabetes, but Not in Normoglycemic Status: A Nationwide Cohort Study. J Clin Oncol 2022;40:3623-32. [Crossref] [PubMed]
- Andreotti G, Hou L, Gao YT, et al. Reproductive factors and risks of biliary tract cancers and stones: a population-based study in Shanghai, China. Br J Cancer 2010;102:1185-9. [Crossref] [PubMed]
- Davey Smith G, Hemani G. Mendelian randomization: genetic anchors for causal inference in epidemiological studies. Hum Mol Genet 2014;23:R89-98. [Crossref] [PubMed]
- Smith GD, Ebrahim S. 'Mendelian randomization': can genetic epidemiology contribute to understanding environmental determinants of disease? Int J Epidemiol 2003;32:1-22. [Crossref] [PubMed]
- Pierce BL, Ahsan H, Vanderweele TJ. Power and instrument strength requirements for Mendelian randomization studies using multiple genetic variants. Int J Epidemiol 2011;40:740-52. [Crossref] [PubMed]
- Staley JR, Blackshaw J, Kamat MA, et al. PhenoScanner: a database of human genotype-phenotype associations. Bioinformatics 2016;32:3207-9. [Crossref] [PubMed]
- Barahona Ponce C, Scherer D, Brinster R, et al. Gallstones, Body Mass Index, C-Reactive Protein, and Gallbladder Cancer: Mendelian Randomization Analysis of Chilean and European Genotype Data. Hepatology 2021;73:1783-96. [Crossref] [PubMed]
- Burgess S, Bowden J, Fall T, et al. Sensitivity Analyses for Robust Causal Inference from Mendelian Randomization Analyses with Multiple Genetic Variants. Epidemiology 2017;28:30-42. [Crossref] [PubMed]
- Bowden J, Davey Smith G, Haycock PC, et al. Consistent Estimation in Mendelian Randomization with Some Invalid Instruments Using a Weighted Median Estimator. Genet Epidemiol 2016;40:304-14. [Crossref] [PubMed]
- Hartwig FP, Davey Smith G, Bowden J. Robust inference in summary data Mendelian randomization via the zero modal pleiotropy assumption. Int J Epidemiol 2017;46:1985-98. [Crossref] [PubMed]
- Bowden J, Davey Smith G, Burgess S. Mendelian randomization with invalid instruments: effect estimation and bias detection through Egger regression. Int J Epidemiol 2015;44:512-25. [Crossref] [PubMed]
- Verbanck M, Chen CY, Neale B, et al. Detection of widespread horizontal pleiotropy in causal relationships inferred from Mendelian randomization between complex traits and diseases. Nat Genet 2018;50:693-8. [Crossref] [PubMed]
- Hemani G, Bowden J, Davey Smith G. Evaluating the potential role of pleiotropy in Mendelian randomization studies. Hum Mol Genet 2018;27:R195-208. [Crossref] [PubMed]
- World Medical Association Declaration of Helsinki. ethical principles for medical research involving human subjects. JAMA 2013;310:2191-4. [Crossref] [PubMed]
- Chodick G, Heymann AD, Rosenmann L, et al. Diabetes and risk of incident cancer: a large population-based cohort study in Israel. Cancer Causes Control 2010;21:879-87. [Crossref] [PubMed]
- Qi J, He P, Yao H, et al. Cancer risk among patients with type 2 diabetes: A real-world study in Shanghai, China. J Diabetes 2019;11:878-83. [Crossref] [PubMed]
- Sheng BW, Zhang JQ, Chen M, et al. The inverse association between fasting blood glucose and the occurrence of gallbladder cancer in type 2 diabetes mellitus patients: a case-control study. J Cancer Res Clin Oncol 2023;149:10387-98. [Crossref] [PubMed]
- Pollak M. The insulin and insulin-like growth factor receptor family in neoplasia: an update. Nat Rev Cancer 2012;12:159-69. [Crossref] [PubMed]
- Vigneri R, Sciacca L, Vigneri P. Rethinking the Relationship between Insulin and Cancer. Trends Endocrinol Metab 2020;31:551-60. [Crossref] [PubMed]
- Nomoto H, Kito K, Iesaka H, et al. Favorable Effect of Pemafibrate on Insulin Resistance and β-Cell Function in Subjects with Type 2 Diabetes and Hypertriglyceridemia: A Subanalysis of the PARM-T2D Study. Pharmaceutics 2023;15:1838. [Crossref] [PubMed]
- Dutta S, Shah RB, Singhal S, et al. Metformin: A Review of Potential Mechanism and Therapeutic Utility Beyond Diabetes. Drug Des Devel Ther 2023;17:1907-32. [Crossref] [PubMed]
- McGee EE, Jackson SS, Petrick JL, et al. Smoking, Alcohol, and Biliary Tract Cancer Risk: A Pooling Project of 26 Prospective Studies. J Natl Cancer Inst 2019;111:1263-78. [Crossref] [PubMed]
- Schwesinger WH, Kurtin WE, Johnson R. Alcohol protects against cholesterol gallstone formation. Ann Surg 1988;207:641-7. [Crossref] [PubMed]
- Chen M, Juengpanich S, Li S, et al. Bortezomib-Encapsulated Dual Responsive Copolymeric Nanoparticles for Gallbladder Cancer Targeted Therapy. Adv Sci (Weinh) 2022;9:e2103895. [Crossref] [PubMed]
- Jackson SS, Adami HO, Andreotti G, et al. Associations between reproductive factors and biliary tract cancers in women from the Biliary Tract Cancers Pooling Project. J Hepatol 2020;73:863-72. [Crossref] [PubMed]
- Shin A, Song YM, Yoo KY, et al. Menstrual factors and cancer risk among Korean women. Int J Epidemiol 2011;40:1261-8. [Crossref] [PubMed]
(English Language Editors: J.Y. Yeo and J. Gray)