Promotion of perineural invasion of cholangiocarcinoma by Schwann cells via nerve growth factor
Highlight box
Key findings
• Schwann cells (SCs) were found to promote the progression and neuroinvasion of cholangiocarcinoma (CCA).
What is known and what is new?
• Current research has shown that SCs exist and play an important role in CCA.
• We found that SCs can act on the tropomyosin receptor kinase A (TrkA) of CCA cells by secreting nerve growth factor (NGF), thereby promoting their invasion—including nerve invasion—and metastasis and NGF acts on the receptor of bile duct cancer cells, thus offering a new avenue for the clinical treatment of CCA.
What is the implication, and what should change now?
• The condition of CCA neuroinvasion may be approached and the corresponding drugs developed via this newly discovered route.
Introduction
Cholangiocarcinoma (CCA) is a highly lethal adenocarcinoma of the hepatobiliary system and can be divided into intrahepatic, perihilar, and distal types (1). Extrahepatic CCA is a malignant tumor originating from extrahepatic bile duct epithelium. Due to its insidious onset and atypical early clinical symptoms, the overall prognosis is poor and the 5-year survival rate is less than 10% (2). In recent years, the emergence of targeted and immunotherapy has achieved good efficacy in hepatoma carcinoma and other diseases but not in CCA. Among the treatment options, surgery is currently the only one that is likely to lead to long-term survival for patients with CCA (3). Although surgery and chemotherapy have made some progress in the treatment of CCA, a portion of patients still experience local recurrence and distant metastasis after surgery, with one of the key contributing factors that leads to the recurrence of CCA being the occurrence of perineural invasion (PNI). PNI involves cancer cells infiltrating and breaking through the outer membrane of bile duct. More specifically, these cells tend to surround the peripheral nerve fibers and nerve tissue, colonizing the nerve tissue or perineural space and even penetrating the nerve bundle membrane into the nerve fibers or promoting endogenous nerve cell growth (4), a process that may prove to be another route of metastasis. Once the tumor infringes upon the nerve, patients often experience intense pain and poor quality of life. Moreover, may make true radical resection during surgery practically infeasible, which limits the curative effect of surgery. This is also one of the root causes of postoperative recurrence, as a previous study has also shown that nerve invasion is an important factor for tumor recurrence and poor prognosis (5).
Schwann cells (SCs) are the main glial cells constituting the peripheral nervous system (PNS). SCs are also referred to as nerve membrane cells because SCs surround the outside of nerve fiber axons. SCs, as an important part of the PNS, play an important role in the formation of the myelin sheath and the repair and regeneration of neurons after injury (6). A study has shown that the destruction of nerve fibers around pancreatic cancer can induce SCs to produce nerve growth factor (NGF), which in turn acts as a tumor promoter (7). In this study, we examined the role of NGF, characterized its association with SCs, and elucidated its potential clinical value in predicting PNI and informing the selection of appropriate surgical approaches for CCA.
Epithelial-mesenchymal transition (EMT) is a biological process in which epithelial cells are transformed into cells with a mesenchymal phenotype through a specific process. It plays an important role in embryonic development, chronic inflammation, tissue reconstruction, cancer metastasis, and a variety of fibrotic diseases (8). EMT has been considered to be an important driver of tumor progression, from initiation to metastasis, and studies have shown that EMT is involved in SC-induced metastasis of salivary adenoid cystic, pancreatic, and lung cancers (9,10). An additional purpose of this study was thus to investigate the relationship between SCs and CCA metastasis and poor prognosis. It was found that SCs promote the proliferation, invasion, EMT, and metastasis of CCA cells. Biliary duct cancer cells can enhance the expression of NGF in SCs, forming a positive feedback loop. Blocking this reciprocal feedback attenuates SC-induced CCA progression, revealing a potential therapeutic target for CCA therapy. However, further studies are needed to uncover the molecular mechanisms underlying the interaction between SCs and CCA. We present this article in accordance with the ARRIVE and MDAR reporting checklists (available at https://jgo.amegroups.com/article/view/10.21037/jgo-24-309/rc).
Methods
Cell cultures
RSC96 SCs were obtained from the Chinese Academy of Sciences Type Culture Collection Cell Bank (species: rat CBTCCCAS; Shanghai, China). CCA cell lines CCLP-1 and HuCCT1 were purchased from the Cell Bank of the Chinese Academy of Sciences (Species: human; Shanghai, China). All cell lines were cultured in high glucose medium [Dulbecco’s modified eagle medium (DMEM); Gibco, Thermo Fisher Scientific, Waltham, MA, USA] containing 10% fetal bovine serum (FBS; Gibco) and 1% penicillin/streptomycin (Gibco) in a 5% CO2 at 37 ℃.
Cell migration and invasion assays
Cell migration and invasion assays were performed using a 24-well plate with a 8.0-µm aperture Transwell invasion chamber (Corning, NY, USA). Cells (1×105) with 0.2 mL FBS-free medium were added to the upper well, while SCs or high-glucose DMEM with 10% FBS was added to the bottom. At 36 h into the invasion experiment, the film was fixed with 4% polyformaldehyde at room temperature for 30 min and then stained with crystal violet staining solution (Solarbio, Beijing, China) for 30 min. Cells in each chamber were quantified by a count of five random regions at 20× magnification.
Wound healing assay
The cancer cells were cultured in a high glucose medium containing 10% FBS to a six-well plate until. 80% of the petri dish. Subsequently, a 200-µL pipette tip was used to make a scratch in the center of petri dish. After cells were rinsed twice with phosphate-buffered saline (PBS), fresh medium was added. The scratch images were collected at 0 and 48 h, and ImageJ software (US National Institutes of Health, Bethesda, MD, USA) was used for processing.
Western blotting
The protein samples were separated via electrophoresis and transferred to nitrocellulose (NC) membrane. After being enclosed in 5% skim milk powder for 2 hours, the NC membrane was incubated with antibodies at 4 ℃ overnight. The next day, they were incubated with secondary antibodies (Abcam, Cambridge, UK) for 1 hour. The strips were collected using an imaging system (BioRad, Hercules, CA, USA). Finally, ImageJ software was used to analyze the gray values of the strip. The primary antibodies used were as follows: glycerine aldehyde phosphate dehydrogenase (GAPDH) (catalog No. ab181602; Abcam), NGF, neural cadherin (N-cadherin), epithelial cadherin (E-cadherin), TWIST, and SNAIL (catalog No. ET1606-29, ET1607-37, EM0502, RT1635, and ER1706-22, respectively; HuaBio, Woburn, MA, USA).
Histological analysis
The tissues were embedded in optimal cutting temperature compound (OCT) and then cut into 7-µm slices. Sections were fixed with 5% paraformaldehyde and then stained with hematoxylin and eosin (HE) or immunofluorescence. For immunofluorescence staining the sections were treated with FBS for 2 hours and incubated at 4 ℃ overnight with primary antibody (GFAP, Abcam). On the second day, the slices were incubated with the corresponding secondary antibody for 1 hour. After nucleus staining with 4’,6-diamidino-2-phenylindole (DAPI) (Solarbio) for 30 min, fluorescence images were captured under a fluorescence microscope (Leica, Wetzlar, Germany).
In vivo PNI assay
Nonobese diabetic-severe combined immunodeficiency disease (NOD-SCID) mice were purchased from Beijing Weitong Lihua Laboratory Animal Technology Co., LTD, and randomly divided into four groups, namely CCLP-1, HuCCT1, CCLP-1-NGF, and HUCCT1-NGF, with 6 mice in each group. CCLP-1-NGF, and HUCCT1-NGF are the addition of exogenous NGF to the cell culture medium. All injected cells (1×106) were adjacent to the sciatic nerve. After 4 weeks, the mice were killed, and the PNI of the tumor was measured. The animal experiment was approved by the Ethics Committee of Experimental Animal Center of Zhengzhou University (No. 2023-KY-1364-002), in compliance with Zhengzhou University guidelines for the care and use of animals. A protocol was prepared before the study without registration.
Cell Counting Kit 8 (CCK8) experiment
After 10 µL of cells were removed from the 96-well plate and continued to culture for an appropriate time, 10 µL CCK8 solution was added to the cell culture plate and continued to incubate in the incubator at 37 ℃ for 4 hours. Then we measured the absorbance of 450 nm wavelength with microcoder (Thermo Fisher).
Colony formation experiment
The cells were diluted to 1×103 cells/mL, and the number of cells per well was 1,000 cells per well, and the exogenous drug was added to the 6-well plate after 1 day of culture. When visible clones appear in the petri dish, the culture can be stopped, fixed for 15 min after washing, and the fixing solution is discarded. Add 0.1% crystal violet to dye for 30 min, wash the dye slowly with running water, and dry in the air. Then we took pictures with a microscope and counted them.
Statistical analysis
GraphPad Prism 9 (GraphPad Software, Inc., La Jolla, CA, USA) was used for data analysis, and all analyses were repeated at least three times. We used the t-test to analyze the expression of NGF in SCs under different conditions and the χ2 test to analyze the correlation between PNI and NGF in CCA. Kaplan-Meier survival analysis was used to analyze the survival time of patients in different groups. We used R-studio software to conduct Kyoto Encyclopedia of Genes and Genomes (KEGG) enrichment analysis, compared foreground genes with background genes, calculated the enrichment significance (P value), and further corrected to get a corrected P value (Q value). With Q value≤0.05 as the threshold, the pathway meeting this condition was defined as the pathway significantly enriched in differentially expressed genes.
Results
The occurrence of PNI in CCA
Immunofluorescence staining of CCA tissues showed that SCs were present in the tumors, and the number of SCs in tumor tissues accompanied by PNI was much higher than that in normal tissues or those tissues without PNI (Figure 1A,1B). In addition, Kaplan-Meier survival analysis showed that patients with CCA and PNI had a shorter overall survival time than did those without PNI (Figure 1C). In summary, SCs were enriched in CCA tissues, indicating the presence of PNI in CCA, which was associated with poor prognosis in patients with CCA. SCs are one of the common cell types in peripheral nerves and play a role in nerve repair and regeneration. SCs have reversible dedifferentiation and redifferentiation functions. After nerve injury, SCs dedifferentiate, lose their ability to myelinate, become more mobile, and engage in a series of interactions with cells in direct contact during repair (11). Direct interactions between SCs and tumor cells can occur at multiple levels, including with direct expression of myelin-associated glycoproteins and nerve cell adhesion molecule 1 (NCAM1), as well as various transforming growth factors (TGFs) (12). In pancreatic cancer, SCs release neurotrophic factors that promote cell growth, survival and maintenance (13). Therefore, neurotrophic factor may be a bridge linking tumor and nerve interaction.
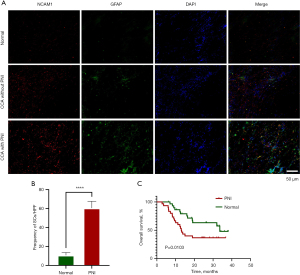
CCA cells in promoting the secretion of NGF via SCs in vitro
A previous study has reported that SCs migrate into colon cancer tissue before the invasion of tumor into the nerve (14). In order to study the interaction between SCs and CCA cells, we stimulated SCs with CCA cell supernatant, and the CCK8 results showed that both CCLP-1 and HuCCT1 could significantly increase the optical density at 450 nm (OD450) value of SCs (Figure 2A). Subsequently, wound healing experiments and Transwell experiments showed that CCA promoted the migration of SCs cells (Figure 2B-2D). SCs are important glial cells in PNS, which can regulate the expression of various neurotrophic factors including NGF (15). NGF, a polymer composed of three subunits, was the earliest neurotrophic factor to be discovered and the most thoroughly studied one. A previous study has shown that coculture of SCs with pancreatic cancer cells can increase the production of NGF in SCs (16). We performed transcriptome sequencing on SCs cocultured with CCLP-1 or HuCCT1 and found that there were significant differences in the expression levels of NGF among many different genes (Figure 2E-2G). Therefore, we verified whether the SCs stimulated by CCA overexpressed NGF. NGF and its blockers GW-441756 were added to SCs for culture. The CCK8 experiment showed that NGF could promote the activity of SCs, and this could be reversed by GW-441756 (Figure 3A). Western blotting showed that NGF secretion in SCs was enhanced after coculture with CCA (Figure 3B). Kyoto Encyclopedia of Genes and Genomes (KEGG) enrichment analysis also indicated that the SCs differential genes after CCA stimulation were involved in the nervous system and related pathways of cell growth and apoptosis (Figure 3C). Furthermore, the colony formation experiment revealed that NGF could promote the colony growth of SCs, which could be inhibited by GW-441756. Finally, the Transwell experiments demonstrated that NGF could promote the invasion of SCs, which could be inhibited by GW-441756 (Figure 4A,4B). This suggests that NGF can promote SC proliferation and migration. Taken together, our data suggest that CCA increases the level of NGF secreted by SCs, which in turn promotes tumor proliferation and migration.
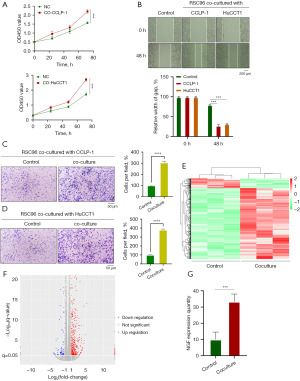
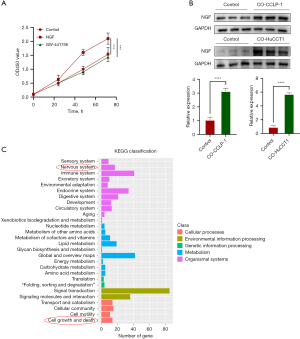
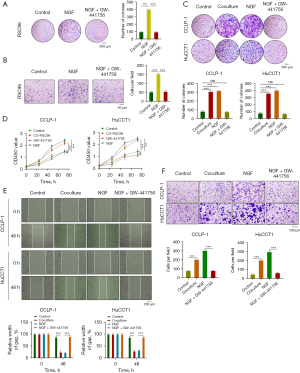
NGF secreted by SCs enhanced the proliferation, migration, and invasion of CCA in vitro
Next, we examined the role of SCs in the progression of CCA in vitro. Colony formation experiments showed that the number and size of colonies of both CCLP-1 and HuCCT1 cells increased after stimulation with SC supernatant compared with the control cells. This was consistent with the results generated by the addition of exogenous NGF, which could be prevented by the addition of NGF-specific blocker GW-441756 (Figure 4C). In addition, CCK8 experiments showed that SCs significantly enhanced the proliferation activity of CCLP-1 and HuCCT1 cells. This was consistent with the results generated by the addition of exogenous NGF, and the addition of NGF-specific inhibitor GW-441756 inhibited this proliferation (Figure 4D). Wound healing experiments showed that coculture with SCs enhanced CCA cell migration, which was consistent with the results from the addition of exogenous NGF, which in turn could be inhibited by the NGF-specific inhibitor GW-441756 (Figure 4E). Similarly, Transwell experiments showed that CCA cells cocultured with SCs exhibited enhanced invasion, which was consistent with the resulted yielded from the addition of exogenous NGF, which could be inhibited by GW-441756 (Figure 4F). In conclusion, SCs can promote the proliferation, migration, and invasion of CCA cells through NGF.
NGF promoted CCA proliferation, migration, and invasion through tropomyosin receptor kinase A (TrkA)
TrkA is a high-affinity receptor for NGF on the cell membrane, and a study has shown its binding with NGF binding can promote the progression of ovarian cancer (17). Therefore, we conducted a series of experiments targeting NGF and its receptor TrkA. CCK8 experiments showed that NGF could promote the proliferation of CCA cell lines, with this process being inhibited by the TrkA blocker GNF5837 (Figure 5A). Colony formation experiments showed that the number of colonies in both CCLP-1 and HuCCT1 increased after the addition of NGF compared to the control cells, and this trend was reversed by the TrkA blocker GNF5837 (Figure 5B). Transwell experiments showed that the migration ability of CCA cells was enhanced after the addition of NGF, but this ability could be inhibited by the TrkA blocker GNF5837 (Figure 5C). In addition, wound healing experiments also obtained the same results (Figure 5D,5E), suggesting that NGF acts on CCA cells through its specific receptor TrkA, thereby promoting their progression.
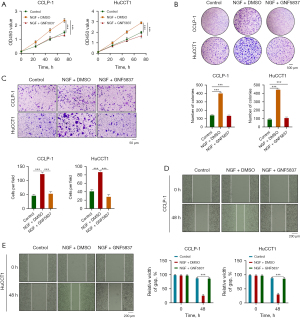
SCs promoted the EMT of CCA cells by secreting NGF and acting on TrkA
EMT is a reversible cellular procedure that causes epithelial cells to briefly enter a quasimesenchymal cell state and undergo changes in appearance (18). EMT plays an important role in specific steps of embryogenesis, such as gastrula formation, tissue morphogenesis during development, and wound healing in adults; however, EMT activation in the vast majority of cancer, promotes progression by endowing the tumor with the characteristics of proliferation and metastasis (19). After activation of EMT, The expression of many marker molecules is altered, such as E-cadherin and N-cadherin, thus altering the characteristics of tumor cells. This can include modification of tumor initiation characteristics, motility, and transmission ability, along with increased resistance to the killing of commonly used chemotherapy drugs (20). NGF has two types of receptors on the cell membrane, one with high affinity, called TrkA, and the other with low affinity, called p75NTR (21). A study has shown that the combination of NGF and TrkA in colon cancer promotes the proliferation, migration and invasion of colon cancer cells (14). Therefore, we treated the CCA cell lines CCLP-1 and HuCCT1 with SC supernatants, exogenous NGF, NGF-specific blockers GW-441756, and TrkA blockers and agonists (GNF5837 and G667C), respectively, and then determined various proteins specific to EMT and analyzed any resulting changes. The results showed that compared with the control cells, both cell lines showed upregulated E-cadherin expression after coculture and addition of exogenous NGF or TrkA agonist G667C, while the addition of NGF blocker GW-441756 or TrkA blocker GNF5837 reversed this phenomenon. The change in N-cadherin was opposite to that of E-cadherin. In addition, the expression of EMT-specific markers TWIST and SNAIL increased regardless of coculture with SCs or addition of exogenous NGF or TrkA receptor agonist G667C, while the addition of NGF blocker GW-441756 or TrkA receptor blocker GNF5837 reversed this (Figure 6A-6C). This suggests that NGF can promote EMT in CCA and thus promote its progression.

NGF contributed to PNI of CCA in vivo
To further explore the interaction between SCs and CCA in vivo, we established a xenotransplantation model with NOD-SCID immunodeficient mice. We injected CCA cells or NGF-cultured CCA cells next to the sciatic nerve in mice, euthanized the mice 4 weeks after, and compared the size and weight of the tumors and the presence of PNI. The results showed that the volume and weight of tumors in the mice increased after the injection of cancer cells with NGF (Figure 7A-7C). We found PNI in the tumor anatomy, and HE and immunofluorescence staining showed the presence of nerve fibers in the tumor (Figure 7D-7F). After biopsy staining of tumor specimens, it was found that the PNI ratio of CCLP-1 tumor cultured with NGF was 83.3% (5/6), which was much higher than that of the control group (1/6, 16.7%). In HuCCT1 tumors, after the addition of NGF, all six mice developed PNI, representing an incidence of 100% (6/6), while that of the control group was 33.3% (2/6) (Figure 7G). This suggests that NGF can promote CCA to produce PNI in vivo.
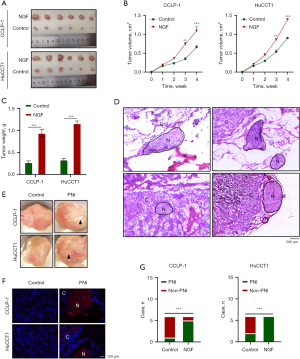
Discussion
The incidence of CCA in gastrointestinal tumors is increasing rapidly (22). Because early symptoms of CCA are not obvious and difficult to detect, most patients are already in the advanced stage when they visit a doctor, and the treatment effect is very poor. Moreover, the low efficacy of surgical resection, the insensitivity to chemotherapy, and the difficulty of targeted therapy due to tumor heterogeneity necessitate the development of new therapeutic modalities for CCA (23). PNI has been acknowledged to figure prominently in tumor development; in this process, tumor cells may infiltrate into the perineural space, thereby persisting after tumor resection and causing postoperative recurrence. In cervical cancer, tumor cells infiltrate into the perineal nerve space and are preserved after tumor removal, leading to local recurrence (24). PNI is associated with poor prognosis in a variety of tumors, including stomach cancer (25), colon cancer (26), and cervical cancer (27). In cervical cancer, PNI can be used as an indication to guide surgery and postoperative adjuvant treatment (27). In our previous study, it was found that PNI is present in CCA and is associated with postoperative local recurrence and poor prognosis of patients, indicating that the presence or absence of PNI may be a key consideration for the choice of treatment.
SCs are important glial cells in the nervous system and play an important role in the progression of tumors (15). A study has shown that SCs activate the phosphatidyl inositol 3-kinase/protein kinase B/glycogen synthesis kinase 3β (PI3K/AKT/GSK-3β) pathway via the chemokine CXCL5-CXCR2 axis, increasing SNAIL and TWIST expression in lung cancer cells and thus promoting EMT and metastasis of lung cancer (9). Research also indicates that SCs migrate into tumor tissue early in tumor progression and embed themselves in cancer clusters, disrupting contact between cells. The protrusions that cancer cells form at the site of SC contact are not sufficient to disperse cancer cells from the cluster (28). We found that CCA can promote the proliferation of SCs and the secretion of NGF, thus promoting proliferation, metastasis, and PNI. In addition, SCs were observed in CCA tissues, which may be related to the tumor’s destruction of adjacent nerve tissue, causing SCs to migrate to perform repair functions. Studies have shown that before the peripheral nerves begin to be invaded by tumors, SCs migrate to colon cancer cells instead of normal colon cells (25,26); in our study, CCA was found to promote the proliferation and migration of SCs, so the presence of SCs in CCA may be related to this process, but additional study is needed to further clarify the related mechanism (29). In addition, transcriptome sequencing in our study indicated that SCs stimulated by CCA had increased NGF secretion, which is attributable to SCs promoting CCA progression. NGF can promote the growth, development, differentiation, and maturation of central and peripheral neurons; maintain the normal function of the nervous system; and accelerate the repair of the nervous system after injury (30). In recent years, many studies have shown that NGF contributes substantially to the development of tumors, which can promote the proliferation, metastasis, and progression of tumors, such as those of breast cancer and ovarian cancer (31,32). Other findings suggest that the overexpression of NGF can significantly accelerate the growth and invasion of gastric tumors (33). In pancreatic cancer, NGF is involved in tumor proliferation and metastasis (34). Our study demonstrated that NGF can promote EMT in CCA through its specific receptor TrkA, thereby promoting CCA progression and the development of PNI.
TrkA is a high-affinity receptor for NGF and is associated with nervous system development and damage repair (35). We found that NGF can specifically bind to TrkA to drive the EMT process of CCA and on this basis, promote the proliferation, metastasis, and invasion of CCA. TrkA plays a bridging role in the process of SCs influence in CCA, and the discovery of its effect may represent a new means for treating CCA. In addition, in some other solid tumors, such as in breast cancer (36) and prostate cancer (37), NGF can also promote tumor EMT in this fashion, thus promoting tumor progression. Therefore, blocking the binding of NGF and its specific receptor TrkA can be used to treat a variety of tumors and may provide a critical benefit in the treatment of clinical diseases.
Although the influence of SCs on the occurrence and development of CCA through NGF has extensively fully studied, no clear findings exist regarding the communication between CCA cells and nervous system SCs in the early stage of CCA development. There is now evidence that exosomes may act as a bridge between tumors and nerves at an early stage (38). Exosomes are extracellular vesicles that contain microRNA that can be used for intercellular communication. Studies have shown that exosomes containing miR21-5p may act on colon cancer cells, thereby promoting their proliferation and metastasis (39,40). However, the relationship between cancer cells and nerve fibers in CCA remains to be further studied.
Conclusions
We found that there is a mutually reinforcing effect between SCs and CCA, leading to the progression of CCA. Targeted blocking of NGF receptor TrkA may be a potential therapeutic strategy in treating CCA. However, further research is needed to clarify the exact molecular mechanism of crosstalk between SCs and CCA.
Acknowledgments
Funding: This work was supported in part by
Footnote
Reporting Checklist: The authors have completed the ARRIVE and MDAR reporting checklists. Available at https://jgo.amegroups.com/article/view/10.21037/jgo-24-309/rc
Data Sharing Statement: Available at https://jgo.amegroups.com/article/view/10.21037/jgo-24-309/dss
Peer Review File: Available at https://jgo.amegroups.com/article/view/10.21037/jgo-24-309/prf
Conflicts of Interest: All authors have completed the ICMJE uniform disclosure form (available at https://jgo.amegroups.com/article/view/10.21037/jgo-24-309/coif). N.T. has served as a consultant for Helsinn, TEMPUS, Astrazeneca, Genentech, Exelixis. The other authors have no conflicts of interest to declare.
Ethical Statement: The authors are accountable for all aspects of the work in ensuring that questions related to the accuracy or integrity of any part of the work are appropriately investigated and resolved. The animal experiment was approved by the Ethics Committee of Experimental Animal Center of Zhengzhou University (No. 2023-KY-1364-002), in compliance with Zhengzhou University guidelines for the care and use of animals.
Open Access Statement: This is an Open Access article distributed in accordance with the Creative Commons Attribution-NonCommercial-NoDerivs 4.0 International License (CC BY-NC-ND 4.0), which permits the non-commercial replication and distribution of the article with the strict proviso that no changes or edits are made and the original work is properly cited (including links to both the formal publication through the relevant DOI and the license). See: https://creativecommons.org/licenses/by-nc-nd/4.0/.
References
- Brindley PJ, Bachini M, Ilyas SI, et al. Cholangiocarcinoma. Nat Rev Dis Primers 2021;7:65. [Crossref] [PubMed]
- Banales JM, Marin JJG, Lamarca A, et al. Cholangiocarcinoma 2020: the next horizon in mechanisms and management. Nat Rev Gastroenterol Hepatol 2020;17:557-88. [Crossref] [PubMed]
- Beaufrère A, Calderaro J, Paradis V. Combined hepatocellular-cholangiocarcinoma: An update. J Hepatol 2021;74:1212-24. [Crossref] [PubMed]
- Li J, Kang R, Tang D. Cellular and molecular mechanisms of perineural invasion of pancreatic ductal adenocarcinoma. Cancer Commun (Lond) 2021;41:642-60. [Crossref] [PubMed]
- Zhang JF, Tao LY, Yang MW, et al. CD74 promotes perineural invasion of cancer cells and mediates neuroplasticity via the AKT/EGR-1/GDNF axis in pancreatic ductal adenocarcinoma. Cancer Lett 2021;508:47-58. [Crossref] [PubMed]
- Deborde S, Gusain L, Powers A, et al. Reprogrammed Schwann Cells Organize into Dynamic Tracks that Promote Pancreatic Cancer Invasion. Cancer Discov 2022;12:2454-73. [Crossref] [PubMed]
- Zhang W, He R, Yang W, et al. Autophagic Schwann cells promote perineural invasion mediated by the NGF/ATG7 paracrine pathway in pancreatic cancer. J Exp Clin Cancer Res 2022;41:48. [Crossref] [PubMed]
- Zhang N, Ng AS, Cai S, et al. Novel therapeutic strategies: targeting epithelial-mesenchymal transition in colorectal cancer. Lancet Oncol 2021;22:e358-68. [Crossref] [PubMed]
- Zhou Y, Shurin GV, Zhong H, et al. Schwann Cells Augment Cell Spreading and Metastasis of Lung Cancer. Cancer Res 2018;78:5927-39. [Crossref] [PubMed]
- Su D, Guo X, Huang L, et al. Tumor-neuroglia interaction promotes pancreatic cancer metastasis. Theranostics 2020;10:5029-47. [Crossref] [PubMed]
- Nocera G, Jacob C. Mechanisms of Schwann cell plasticity involved in peripheral nerve repair after injury. Cell Mol Life Sci 2020;77:3977-89. [Crossref] [PubMed]
- Na'ara S, Amit M, Gil Z. L1CAM induces perineural invasion of pancreas cancer cells by upregulation of metalloproteinase expression. Oncogene 2019;38:596-608. [Crossref] [PubMed]
- Banh RS, Biancur DE, Yamamoto K, et al. Neurons Release Serine to Support mRNA Translation in Pancreatic Cancer. Cell 2020;183:1202-1218.e25. [Crossref] [PubMed]
- Han S, Wang D, Huang Y, et al. A reciprocal feedback between colon cancer cells and Schwann cells promotes the proliferation and metastasis of colon cancer. J Exp Clin Cancer Res 2022;41:348. [Crossref] [PubMed]
- Martyn GV, Shurin GV, Keskinov AA, et al. Schwann cells shape the neuro-immune environs and control cancer progression. Cancer Immunol Immunother 2019;68:1819-29. [Crossref] [PubMed]
- Demir IE, Boldis A, Pfitzinger PL, et al. Investigation of Schwann cells at neoplastic cell sites before the onset of cancer invasion. J Natl Cancer Inst 2014;106:dju184. [Crossref] [PubMed]
- Garrido MP, Vallejos C, Girardi S, et al. NGF/TRKA Promotes ADAM17-Dependent Cleavage of P75 in Ovarian Cells: Elucidating a Pro-Tumoral Mechanism. Int J Mol Sci 2022;23:2124. [Crossref] [PubMed]
- Nieto MA, Huang RY, Jackson RA, et al. EMT: 2016. Cell 2016;166:21-45. [Crossref] [PubMed]
- Krebs AM, Mitschke J, Lasierra Losada M, et al. The EMT-activator Zeb1 is a key factor for cell plasticity and promotes metastasis in pancreatic cancer. Nat Cell Biol 2017;19:518-29. [Crossref] [PubMed]
- Manfioletti G, Fedele M. Epithelial-Mesenchymal Transition (EMT) 2021. Int J Mol Sci 2022;23:5848. [Crossref] [PubMed]
- Lee S, Hwang C, Marini S, et al. NGF-TrkA signaling dictates neural ingrowth and aberrant osteochondral differentiation after soft tissue trauma. Nat Commun 2021;12:4939. [Crossref] [PubMed]
- Rodrigues PM, Olaizola P, Paiva NA, et al. Pathogenesis of Cholangiocarcinoma. Annu Rev Pathol 2021;16:433-63. [Crossref] [PubMed]
- Wang M, Chen Z, Guo P, et al. Therapy for advanced cholangiocarcinoma: Current knowledge and future potential. J Cell Mol Med 2021;25:618-28. [Crossref] [PubMed]
- Poddar P, Maheshwari A. Surgery for cervical cancer: consensus & controversies. Indian J Med Res 2021;154:284-92. [Crossref] [PubMed]
- Deng J, You Q, Gao Y, et al. Prognostic value of perineural invasion in gastric cancer: a systematic review and meta-analysis. PLoS One 2014;9:e88907. [Crossref] [PubMed]
- Huang Y, He L, Dong D, et al. Individualized prediction of perineural invasion in colorectal cancer: development and validation of a radiomics prediction model. Chin J Cancer Res 2018;30:40-50. [Crossref] [PubMed]
- Zhu Y, Zhang G, Yang Y, et al. Perineural invasion in early-stage cervical cancer and its relevance following surgery. Oncol Lett 2018;15:6555-61. [Crossref] [PubMed]
- Deborde S, Omelchenko T, Lyubchik A, et al. Schwann cells induce cancer cell dispersion and invasion. J Clin Invest 2016;126:1538-54. [Crossref] [PubMed]
- Duchalais E, Guilluy C, Nedellec S, et al. Colorectal Cancer Cells Adhere to and Migrate Along the Neurons of the Enteric Nervous System. Cell Mol Gastroenterol Hepatol 2017;5:31-49. [Crossref] [PubMed]
- Rocco ML, Soligo M, Manni L, et al. Nerve Growth Factor: Early Studies and Recent Clinical Trials. Curr Neuropharmacol 2018;16:1455-65. [Crossref] [PubMed]
- Bruno F, Arcuri D, Vozzo F, et al. Expression and Signaling Pathways of Nerve Growth Factor (NGF) and Pro-NGF in Breast Cancer: A Systematic Review. Curr Oncol 2022;29:8103-20. [Crossref] [PubMed]
- Vera DB, Fredes AN, Garrido MP, et al. Role of Mitochondria in Interplay between NGF/TRKA, miR-145 and Possible Therapeutic Strategies for Epithelial Ovarian Cancer. Life (Basel) 2021;12:8. [Crossref] [PubMed]
- Hayakawa Y, Sakitani K, Konishi M, et al. Nerve Growth Factor Promotes Gastric Tumorigenesis through Aberrant Cholinergic Signaling. Cancer Cell 2017;31:21-34. [Crossref] [PubMed]
- Lei Y, Tang L, Xie Y, et al. Gold nanoclusters-assisted delivery of NGF siRNA for effective treatment of pancreatic cancer. Nat Commun 2017;8:15130. [Crossref] [PubMed]
- Wang Y, Lai X, Wu D, et al. Umbilical mesenchymal stem cell-derived exosomes facilitate spinal cord functional recovery through the miR-199a-3p/145-5p-mediated NGF/TrkA signaling pathway in rats. Stem Cell Res Ther 2021;12:117. [Crossref] [PubMed]
- Tomellini E, Touil Y, Lagadec C, et al. Nerve growth factor and proNGF simultaneously promote symmetric self-renewal, quiescence, and epithelial to mesenchymal transition to enlarge the breast cancer stem cell compartment. Stem Cells 2015;33:342-53. [Crossref] [PubMed]
- Di Donato M, Cernera G, Migliaccio A, et al. Nerve Growth Factor Induces Proliferation and Aggressiveness In Prostate Cancer Cells. Cancers (Basel) 2019;11:784. [Crossref] [PubMed]
- Isaac R, Reis FCG, Ying W, et al. Exosomes as mediators of intercellular crosstalk in metabolism. Cell Metab 2021;33:1744-62. [Crossref] [PubMed]
- Yu W, Zhu K, Wang Y, et al. Overexpression of miR-21-5p promotes proliferation and invasion of colon adenocarcinoma cells through targeting CHL1. Mol Med 2018;24:36. [Crossref] [PubMed]
- Zou M, Sheng J, Ruan M, et al. Perineural invasion confers poorer clinical outcomes in patients with T1/T2 intrahepatic cholangiocarcinoma: a single center, retrospective cohort study. J Gastrointest Oncol 2023;14:2500-10. [Crossref] [PubMed]