Establishing a standardized murine orthotopic intra-rectal model for the study of colorectal adenocarcinoma
Highlight box
Key findings
• The intra-rectal injection method yielded a 92% success rate in males and 95% in females, proving effective for growing colorectal adenocarcinoma. Tumor growth reached nearly 90% within two weeks.
• Elevating the pelvis using a novel 45° wedge, increased the visibility of the injection field leading to more successful intra-rectal injections, while minimizing respiratory distress in the mice.
• A comparative analysis between male and female mice showed no significant differences in tumor growth.
• Immunohistochemistry revealed vascular networks at the tumor periphery, along with apoptotic centers.
What is known and what is new?
• The use of Matrigel facilitated successful tumor growth without the need for acetic acid. Matrigel prevented cell leakage and provided nutrition for tumor proliferation, even with smaller Matrigel volumes than those used in previous studies.
• Magnetic resonance imaging (MRI) allowed a thorough assessment of tumor growth and identify cases of misplaced injections, highlighting the precision and non-invasiveness of this imaging modality.
What is the implication, and what should change now?
• The novel 45° wedge technique could become a new standard for researchers, as it reduces technical failures related to injection accuracy and increase overall success rates in tumor growth.
• Acetic acid is unnecessary for successful tumor uptake. Shifting away from using acetic acid will reduce animal discomfort, the risk of complications such as infections or disruption of fecal excretion.
Introduction
Colorectal cancer (CRC), along with lung, breast, and prostate, are expected to account for about 46% of all cancers diagnosed in 2023. Even though the CRC incidence rates are declining, approximately 24,100 Canadians are expected to be diagnosed with CRC in 2023 (1). These statistics highlight the need for developing novel and efficient treatment protocols and modalities.
Small animal models are indispensable tools in cancer research, offering reproducible, modifiable, and dependable platforms for studying tumor growth and therapeutic interventions. To understand CRC biology, one must consider its local growth dynamics, cellular and molecular microenvironment, and vascularization patterns. Such characteristics are often lost in traditional subcutaneous models, highlighting the value of orthotopic models in recapitulating the complexity of human CRC.
A review from Stribbling et al. [2024] (2) underlines the differences and importance of completing orthotopic animal models for various types of cancers, further emphasizing the significance of establishing an orthotopic intra-rectal injection model for CRC. Among orthotopic models, murine intra-rectal injection has captured significant attention for its ability to replicate the natural progression of CRC. By inoculating colorectal adenocarcinoma cells directly into the mucosal layer of the rectum, these models replicate human CRC with high fidelity (3). Many studies have reported orthotopic murine models such as the intra-rectal injection (4-11) and the intra-cecal injection (3,12-14). Furthermore, several variations of intra-rectal models exist, each offering unique advantages and insights into CRC biology (2). Cell-derived xenografts (CDX) are models that involve the transplantation of established CRC cell lines into immune-compromised mice, allowing for the study of tumor growth and response to therapy in a controlled environment. Additionally, the syngeneic models utilize immune-competent mice in which CRC cells derived from the same genetic background as the host are injected, facilitating the investigation of tumor-immune interactions and immunotherapy. Moreover, patient-derived orthotopic xenografts (PDOX) are models encompassing the direct implantation of patient-derived CRC tissues into recipient mice, preserving the heterogeneity and microenvironment of human tumors, thereby enabling personalized medical approaches (2). Despite the plethora of existing models, a comprehensive understanding of their comparative advantages and limitations is important for selecting the most appropriate model for specific research objectives (15).
This study aimed to establish a workflow for an orthotopic intra-rectal model for human colorectal adenocarcinoma growth in male and female mice. Through detailed methodology optimization and rigorous validation, we aimed to contribute to the expanding repertoire of preclinical models available for CRC research. This model will later investigate a treatment modality called diffusing alpha-emitter radiation therapy for treating CRC. We present this article in accordance with the ARRIVE reporting checklist (available at https://jgo.amegroups.com/article/view/10.21037/jgo-24-515/rc).
Methods
This study established an intra-rectal injection of HT-29 colorectal adenocarcinoma cells in a murine model. The methods are further described in the sections below.
Cell culture
The human colorectal adenocarcinoma HT-29 cell line was purchased from the American type culture collection (ATCC). Cells were grown in McCoy’s 5a Medium Modified (cat. # 317-010-CL, Wisent, Montreal, Canada), in 5% CO2 humidified incubator at 37 ℃. All media were supplemented with 10% fetal bovine serum (Wisent, cat. # 080-150), penicillin (100 U/mL) and streptomycin (100 µg/mL) (cat. # 15140122, ThermoFisher, Ottawa, Canada). When the cells reached 80% confluence, they were trypsinized and neutralized by adding media. The cell suspensions from each flask were combined into a sterile 50 mL tube. Cell counting was then performed to determine the required media/Matrigel volumes, aiming for a final cell concentration of 1×106 cells per 25 µL Matrigel/media. Matrigel Matrix (Corning, New York, USA) was used for this purpose. The pooled cell suspension was centrifuged for 500 ×g for 5 minutes, after which the media was discarded, leaving the cell pellet. Based on the cell count, the cell pellet was resuspended in media without fetal bovine serum and gently mixed 1 mL pipette tip to break up any clumps. The cells were then chilled on ice for 5 minutes. The desired number of aliquots of Matrigel was thawed overnight in a 4 ℃ cold room. A final suspension with a 50% Matrigel to 50% media ratio was prepared, kept on ice to prevent the Matrigel from solidifying. All syringes and needle were also chilled on ice before inoculation, ensuring that each syringe contained 25 µL of the 1×106 cells in Matrigel/media.
Mouse model
Forty-seven NSG mice (8–12 weeks old), both female (n=21) and male (n=26), were obtained from the Animal Resources Division at the Research Institute of the McGill University Health Center (RI-MUHC), Montreal, Canada. Environmental enrichment such as cardboard huts were provided to the mice if fighting occurred. All surgical and invasive procedures were completed under anesthesia by inhalation of isoflurane (between 2% to 2.5%) and oxygen (between 0.4 to 0.8 L/min). The mice received analgesia of carprofen (20 mg/kg) subcutaneously for the first 72 hours post-surgery. The mice were euthanized by CO2 asphyxiation as the primary method of euthanasia. A secondary method of cervical dislocation was administered. Experiments were performed under a project license (No. 8254-2021) granted by the institutional ethics board of The Research Institute at McGill University Health Center, in compliance with institutional guidelines (in which follow the Canadian Council on Animal Care guidelines) for the care and use of animals.
Intra-rectal injection
The mice were anesthetized and placed over a wedge of ~45° to allow for better visualization of the anal canal as illustrated in Figure 1. The tails were taped so the mice would stay stretched along the wedge. A light-emitting diode (LED) magnifying surgical lamp was clipped to the surgical bench for optimal surgical field visibility. Prior to sterilization of the field, feces were expelled by gently palpating the lower abdomen of the anesthetized mice to clear the intestinal tract and aid in an accurate injection. The anus was washed three times with chlorhexidine. The use of 70% alcohol was avoided due to the potential risk of causing anal prolapse. Fine point high precision forceps and/or curved, very fine precision tip forceps were used to gently enlarge the anus enough to have access to the rectum of the mice without over-extending the anus and causing damage to the external anal sphincter as shown in Figure 2. The reflexes were tested every two to three minutes to ensure the mice were fully anesthetized. Cells were injected at a concentration of 1×106 cells/25 µL Matrigel/media using a 28-G needle tip into the distal mucosal layer of the rectum, 3–5 mm proximal to the anal canal. The injection was completed with care to avoid injecting into the dorsal pelvic cavity. Local tumor growth was monitored weekly with the 7-T magnetic resonance imaging (MRI) at the RI-MUHC Montreal, Canada, using a rapid acquisition with relaxation echo (RARE)-acquisition sequence. Three mutually orthogonal tumor dimensions were measured using the 3D Slicer Software (Harvard University, National Institutes of Health) (16).
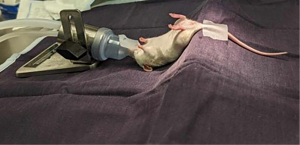
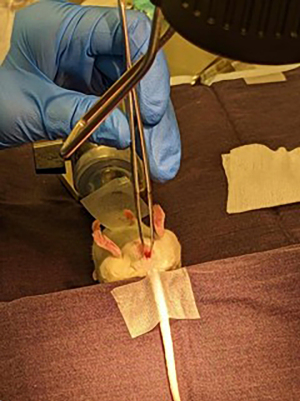
The volume of the tumors was calculated using the well-established (17) tumor volume estimation hemi-ellipsoid shape as presented in Eq. [1] below:
Where V is the volume (mm3), l is the length (mm) from the horizontal x-direction of the axial slice of the magnetic resonance (MR) image, w is the width (mm) and is the measurement perpendicular to the length on the axial slice of the MR image, and h is the height (mm) and is the measured vertical length from the sagittal slice of the MR image (Figure 3).
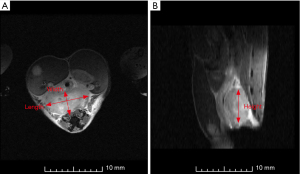
Histopathology
At the end of the study, tumors were extracted from the mice. The samples were flash-frozen in dry ice and then halved with a surgical scalpel. Following standard procedures, the specimens were embedded in Tissue-Tek® O.C.T Compound (cat. #14-373-65, ThermoFisher, Ottawa, Canada). Histological sections with a thickness of 10 µm were obtained using a Leica CM1950 cryostat (Leica, Nussloch, Germany) and fixed on positively charged glass slides using 10% formalin. Subsequently, the slides underwent staining by the Histopathology Core at the RI-MUHC (Montreal, Canada). Stains included hematoxylin-eosin (H&E) for overall morphology and necrotic tissue identification, cluster of differentiation 31 (CD-31) for hypoxia/vascularity detection and cleaved caspase-3 for apoptosis detection. The stained slides were scanned at 20× magnification using a Leica Aperio AT Turbo digital pathology scanner (Leica, Nussloch, Germany). The stained slides were evaluated by a board-certified veterinary pathologist.
Statistical analysis
Data presented are representative of two similar experiments. To assess statistical significance of differences, an unpaired Student’s t-test (OriginPro 2024, OriginLab, Northampton, USA) was used. A P value <0.05 was considered significant, and P<0.01 was considered very significant.
Results
The subsequent sections present the results of the orthotopic intra-rectal model, focusing on tumor growth cellular characteristics, and a comparison between the genders of the mice.
Tumor growth
This study aimed to induce tumor growth of approximately 5–8 mm in diameter within the submucosal layer of the rectum and establish a foundation for future investigations into CRC treatment. This orthotopic intra-rectal model demonstrated a tumor growth success rate of up to 95%. Tumors reached their desired size within ~20 days. Some tumors remained small, possibly due to injection method failures on the day of cell injection. Only 18 mice exhibited visible tumor growth on the first day of imaging. By the end of the second week, visible tumors were observed in 26 mice, increasing to 28 mice by the third week. The tumor incidence is depicted in Table 1. While male mice exhibited a higher incidence rate than females, no statistically significant difference was observed between the two groups. Figure 4 illustrates the progression of tumor size over time. Noticeable variation in average tumor volumes may be attributed to differences in the success of cell injection, with six requiring a second attempt. The cell injections that required a second attempt was determined due to the solution coming out of the anus instead of going into the tissue. The second attempt did not influence the mispositioned tumor growth and did not have delays in tumor growth. The mice that received a second injection did not have larger tumors than the others. Male mice displayed lower variability in tumor volume compared to females. Additionally, male mice tended to have larger tumor volumes, although these differences were not statistically significant between male and female groups.
Table 1
Gender | Week 1 | Week 2 | Week 3 |
---|---|---|---|
Male | 69% | 88% | 92% |
Female | 71% | 86% | 95% |
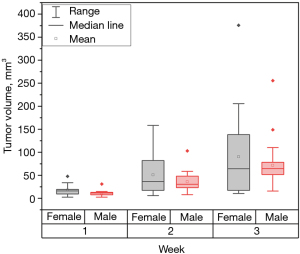
Administering HT-29 cell injections demands a precise technique, particularly due to the challenge of injecting into the delicate submucosal layer of the rectum. Consequently, some tumors failed to develop within the mucosa, instead growing beyond the muscular layer (Figure 5). Despite this, they were not omitted from the study because these tumors established a vascular network near the rectum, and the microenvironments at both injection sites remained comparable.
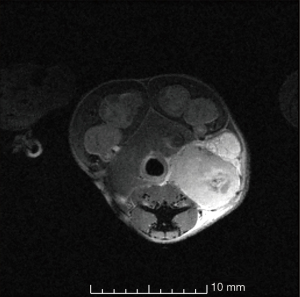
Animal care and monitoring were performed daily. Pain indications such as animal weight, mouse grimace scales, ability to urinate and defecate, hydration status, movement, body conditions (hunched/ruffled), were monitored daily. The stomachs of the mice were scruffed, and their abdomen and pelvis were gently tickled to activate urination and defecation. If there were any doubts or concerns of animal welfare, veterinary technicians ensured the health of the mice. No pain indications were observed in the mice throughout the study. The growth of the tumors did not disrupt their fecal excretion. However, some mice exhibited elongation of the external anal sphincter, resulting in a straightened rather than round anus. This was considered a normal response as it did not affect fecal excretion. Towards the end of the study, palpable tumors near the anus were noted, although imaging proved to be a more accurate method for measuring tumor sizes. At the time of euthanasia, the tumors were firmly attached to the rectum of the mice, as shown in Figure 6.
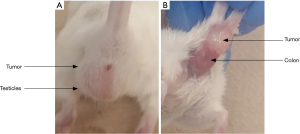
Histopathology
Histopathology was consistent with poorly differentiated adenocarcinoma. Clear areas of necrosis were noted in the center of the tumors, whereas increased vascular activity was noted at the periphery. Apoptosis was observed within the central regions of the tumors, alongside the necrosis. Due to the lack of vascularity in these regions, the increased apoptosis was interpreted as probable hypoxia-related cell death. This can be observed in the Figure 7 below. The tumor growth was both tangentially and circumferentially, as indicated by the histology slides.
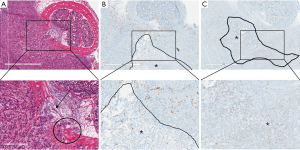
Discussion
The intra-rectal injection proved to be an efficient method of growing HT-29 colorectal adenocarcinoma with a 92% and 95% success rate in males and females, respectively. There were three instances where the tumors accidentally grew in the muscularis due to a suspected misplaced injection. These were assessed by thoroughly investigating the MRIs taken for each mouse during the tumor growth process. Despite these occasional occurrences, the tumor growth rate reached nearly 90% after two weeks. Based on these results, we anticipate that the lack of visible tumors after two weeks would be consistent with a potential technical failure. An additional recommendation using this model would be to increase the overall number of animals injected by at least 10%, if ethically possible, to ensure that the required sample size is achieved for research purposes, especially when re-injection of new animals is not feasible for single-trial studies.
This CRC intra-rectal approach is particularly valuable given that the microenvironment closely mimics human CRC (2), thus providing a relevant platform for assessing treatment efficacy. We pioneered the utilization of a novel 45° wedge technique in our experimental set-up, whereby the anus of the mice is elevated to enhance visibility during the intra-rectal injections. This innovative approach allows for clear visualization of the anus opening and precise administration of cell/Matrigel clusters into the rectal mucosa. In contrast, traditional methods involving mice in a supine position would compromise visibility, impeding accurate injection targeting. Furthermore, the wedge is set at an angle of 45° to ensure optimal injection visibility without compromising the respiratory function of the mice. A larger angle would risk interference with breathing by causing organ displacement and exerting pressure on the diaphragm, impeding respiratory mechanics and potentially compromising the well-being of the animals.
A similar study by Hite et al. [2018] (5) has also investigated the growth of HT-29 cells using the intra-rectal and intra-cecal methods and compared the two methods. They have reported a 100% primary tumor growth success rate for both the intra-rectal and intra-cecal methods. However, they also discuss that the intra-rectal model is the safest, most reproducible, and most successful orthotopic CRC mouse model for primary tumor growth. The intra-cecal model requires an abdominal incision and suture closure of the abdominal wall, whereas the intra-rectal model is a simple, less invasive and quick trans-anal injection (5).
Other studies, such as Lee et al. (18) [2012], Hite et al. (5) [2018] and others (6,7) have employed strong acids to induce significant inflammation of the colonic mucosa, thereby facilitating increased tumor deposition during tumor cell enemas. While this approach has effectively promoted tumor uptake, it is notable for its invasiveness. The method poses a heightened risk of infection, inflammation, increased pain for the mice, and potential disruptions to fecal excretion.
Additionally, Kishimoto et al. (7) [2013], demonstrated tumor growth using CT26-GFP CRC cells mixed with Matrigel, following disruption of the rectal lumen with 4% acetic acid, and subsequent injection into the rectal mucosa using a 28-G needle. However, their attempts to induce tumor growth using solely cancer cells or acetic acid disruption alone were unsuccessful. In contrast, our study achieved successful tumor growth using cancer cells combined with Matrigel without the use of acetic acid, indicating that acetic acid may not be essential for CRC cell injection. Our study used the cancer cells alone with Matrigel and grew successful tumors. Therefore, this notes that the acetic acid method is unnecessary for injecting the CRC cells. Our approach involved a single injection into the submucosal layer of the rectum, resulting in sufficient tumor growth that closely mimics the microenvironment of human CRC.
Moreover, suspending the cell concentration in Matrigel yielded several advantages. Matrigel facilitated the injection process by solidifying at temperatures exceeding 10 ℃, thus forming a robust cluster of cells upon injection into the tissue layer. This solidification mechanism prevented leakage of cells into the anal canal from the injection site. Additionally, Matrigel provides a rich source of nutrients, ensuring adequate nutrition for cell proliferation and subsequent tumor formation. Previous studies have also used Matrigel to enhance tumor growth (6,7). Notably, Takahashi et al. (6) [2004] and Kishimoto et al. (7) [2013] used volumes of 100 and 50 µL of Matrigel, respectively. In contrast, our study employed a smaller volume of 25 µL of Matrigel/media, a decision influenced by recommendations from the veterinary staff at the animal facility of the RI-MUHC. Although the volume of Matrigel differed, the cell concentration remained consistent with previous studies. This reduction in Matrigel volume minimized the risk of rupturing the rectal mucosa during injection and minimized cell leakage from the injection site by facilitating the rapid formation of the Matrigel pellet. Additionally, a study by Schölch et al. (19) [2016] observed successful tumor growth with a similar volume of 20 µL of HT-29 cells suspended in Matrigel, further supporting our approach.
To date, Kashtan et al. (4) [1992] is the only study that has included the use of female and male mice. However, a direct comparison of tumor sizes was precluded due to their use of different cell lines for each gender. Historically, male mice have predominantly been used for studies focusing on the growth of orthotopic tumors via intra-rectal and intra-cecal approaches (6,8,9,12,14). Nonetheless, a handful of studies have used female mice for similar purposes (13,18). Our investigation marks the first instance of a comparative analysis of tumor uptake in female and male mice. As presented in Figure 4, no discernible correlation exists between the gender of the animal and tumor growth. Rather, success in tumor growth hinges upon the efficacy of cell injection into the rectal mucosa.
Histopathology sections exhibit morphological characteristics consistent with those documented in previous studies (5-7,14,20,21). The tumors demonstrate invasion into the normal mucosa and submucosa of the rectum. H&E staining reveals that the tumors exhibit self-induced necrosis three weeks after cell injection, a feature also noted in the colorectal adenocarcinoma cell line HCT-116 by Céspedes et al. (14) [2007]. This study is the first to present immunohistochemistry results for an orthotopic intra-rectal model for CD-31 and cleaved caspase-3 staining. Although, Yokoi et al. (20) [2005] previously observed CD-31 positively stained cells in orthotopic intra-cecal injections of HT-29 tumor models. Their findings are consistent with ours, indicating vascular networks at the tumor periphery, leading to an apoptotic center as indicated by the cleaved caspase-3 stain.
Our study establishes the groundwork for future investigations into CRC treatment using the orthotopic intra-rectal model. While our focus was on tumor growth dynamics and the efficacy of cell injection techniques, there are numerous avenues for further exploration. For instance, future research could explore the molecular mechanisms underlying tumor response for various treatments, including brachytherapy. Ultimately, the versatility and fidelity of the orthotopic intra-rectal model are promising for advancing our understanding of CRC pathogenesis and developing novel therapeutic strategies.
Conclusions
This study investigated the development of an orthotopic intra-rectal injection model using an NSG murine animal model. Employing this method, we examined the tumor growth of HT-29 colorectal adenocarcinoma cells by comparing growth rates, cellular structures, and experimental techniques with existing literature. Our efforts successfully established the orthotopic intra-rectal model, laying the foundation for future investigations.
Acknowledgments
Funding: This work was supported by part of
Footnote
Reporting Checklist: The authors have completed the ARRIVE reporting checklist. Available at https://jgo.amegroups.com/article/view/10.21037/jgo-24-515/rc
Data Sharing Statement: Available at https://jgo.amegroups.com/article/view/10.21037/jgo-24-515/dss
Peer Review File: Available at https://jgo.amegroups.com/article/view/10.21037/jgo-24-515/prf
Conflicts of Interest: All authors have completed the ICMJE uniform disclosure form (available at https://jgo.amegroups.com/article/view/10.21037/jgo-24-515/coif). The authors have no conflicts of interest to declare.
Ethical Statement: The authors are accountable for all aspects of the work in ensuring that questions related to the accuracy or integrity of any part of the work are appropriately investigated and resolved. Experiments were performed under a project license (No. 8254-2021) granted by the institutional ethics board of The Research Institute at McGill University Health Center, in compliance with institutional guidelines (in which follow the Canadian Council on Animal Care guidelines) for the care and use of animals.
Open Access Statement: This is an Open Access article distributed in accordance with the Creative Commons Attribution-NonCommercial-NoDerivs 4.0 International License (CC BY-NC-ND 4.0), which permits the non-commercial replication and distribution of the article with the strict proviso that no changes or edits are made and the original work is properly cited (including links to both the formal publication through the relevant DOI and the license). See: https://creativecommons.org/licenses/by-nc-nd/4.0/.
References
- Canadian Cancer Statistics Advisory in collaboration with the Canadian Cancer Society and the Public Health Agency of Canada. Canadian Cancer Statistics; 2023.
- Stribbling SM, Beach C, Ryan AJ. Orthotopic and metastatic tumour models in preclinical cancer research. Pharmacol Ther 2024;257:108631. [Crossref] [PubMed]
- Tseng W, Leong X, Engleman E. Orthotopic mouse model of colorectal cancer. J Vis Exp 2007;484. [PubMed]
- Kashtan H, Rabau M, Mullen JB, et al. Intra-rectal injection of tumour cells: a novel animal model of rectal cancer. Surg Oncol 1992;1:251-6. [Crossref] [PubMed]
- Hite N, Klinger A, Hellmers L, et al. An Optimal Orthotopic Mouse Model for Human Colorectal Cancer Primary Tumor Growth and Spontaneous Metastasis. Dis Colon Rectum 2018;61:698-705. [Crossref] [PubMed]
- Takahashi T, Morotomi M, Nomoto K. A novel mouse model of rectal cancer established by orthotopic implantation of colon cancer cells. Cancer Sci 2004;95:514-9. [Crossref] [PubMed]
- Kishimoto H, Momiyama M, Aki R, et al. Development of a clinically-precise mouse model of rectal cancer. PLoS One 2013;8:e79453. [Crossref] [PubMed]
- Donigan M, Norcross LS, Aversa J, et al. Novel murine model for colon cancer: non-operative trans-anal rectal injection. J Surg Res 2009;154:299-303. [Crossref] [PubMed]
- Zigmond E, Halpern Z, Elinav E, et al. Utilization of murine colonoscopy for orthotopic implantation of colorectal cancer. PLoS One 2011;6:e28858. [Crossref] [PubMed]
- Kasashima H, Duran A, Cid-Diaz T, et al. Mouse model of colorectal cancer: orthotopic co-implantation of tumor and stroma cells in cecum and rectum. STAR Protoc 2021;2:100297. [Crossref] [PubMed]
- Kasashima H, Duran A, Martinez-Ordoñez A, et al. Stromal SOX2 Upregulation Promotes Tumorigenesis through the Generation of a SFRP1/2-Expressing Cancer-Associated Fibroblast Population. Dev Cell 2021;56:95-110.e10. [Crossref] [PubMed]
- Ogata Y, Hara Y, Akagi Y, et al. Metastatic model of human colon cancer constructed using orthotopic implantation in nude mice. Kurume Med J 1998;45:121-5. [Crossref] [PubMed]
- Hackl C, Man S, Francia G, et al. Metronomic oral topotecan prolongs survival and reduces liver metastasis in improved preclinical orthotopic and adjuvant therapy colon cancer models. Gut 2013;62:259-71. [Crossref] [PubMed]
- Céspedes MV, Espina C, García-Cabezas MA, et al. Orthotopic microinjection of human colon cancer cells in nude mice induces tumor foci in all clinically relevant metastatic sites. Am J Pathol 2007;170:1077-85. [Crossref] [PubMed]
- Mittal VK, Bhullar JS, Jayant K. Animal models of human colorectal cancer: Current status, uses and limitations. World J Gastroenterol 2015;21:11854-61. [Crossref] [PubMed]
- Fedorov A, Beichel R, Kalpathy-Cramer J, et al. 3D Slicer as an imaging computing platform for the quantitative imaging network. Magnetic Resonance Imaging 2012;30:1323-41. [Crossref] [PubMed]
- Sápi J, Kovács L, Drexler DA, et al. Tumor Volume Estimation and Quasi-Continuous Administration for Most Effective Bevacizumab Therapy. PLoS One 2015;10:e0142190. [Crossref] [PubMed]
- Lee JS, Bae MH, Choi SH, et al. Tumor establishment features of orthotopic murine bladder cancer models. Korean J Urol 2012;53:396-400. [Crossref] [PubMed]
- Schölch S, García S, Iwata N, et al. Circulating tumor cells exhibit stem cell character-istics in an orthotopic mouse model of colorectal cancer. Oncotarget 2016;7:27232-42. [Crossref] [PubMed]
- Yokoi K, Thaker PH, Yazici S, et al. Dual inhibition of epidermal growth factor receptor and vascular endothelial growth factor receptor phosphorylation by AEE788 reduces growth and metastasis of human colon carcinoma in an orthotopic nude mouse model. Cancer Res 2005;65:3716-25. [Crossref] [PubMed]
- Uccello TP, Kintzel SA, Mills BN, et al. Development of an Orthotopic Murine Model of Rectal Cancer in Conjunction With Targeted Short-Course Radiation Therapy. Adv Radiat Oncol 2021;7:100867. [Crossref] [PubMed]