Systematic review and meta-analysis of the prognostic role of fibroblast-activation protein in gastrointestinal cancers
Highlight box
Key findings
• Fibroblast-activation protein (FAP) overexpression in gastrointestinal (GI) cancers is associated with poorer overall survival (OS), as shown in 31 cohort studies involving 3,976 patients. This association was consistent across several GI cancers, including esophageal, pancreatic, colorectal, and gastric cancers. Furthermore, patients with FAP overexpression exhibited a higher frequency of lymph node metastases compared to those without FAP overexpression.
What is known and what is new?
• FAP is a marker predominantly observed in cancer-associated fibroblasts (CAFs) within the tumor microenvironment of GI cancers. Its expression is linked to adverse outcomes, including poorer OS and increased lymph node metastases.
• The findings highlight the need for standardized definitions and cutoff values for FAP expression in research.
What is the implication, and what should change now?
• The study underscores the potential of FAP as a prognostic marker and a therapeutic target in GI cancers. The variability in FAP expression and its impact on survival across different GI cancers calls for further investigation into its role and the development of selective FAP inhibitors or targeted therapies. Future research should focus on identifying and targeting specific FAP-positive CAF subtypes that contribute to tumor progression. Standardization in measuring FAP expression and controlling for confounding factors in studies are critical steps to improve the reliability of research in this area.
Introduction
Cancers of the gastrointestinal (GI) tract include esophageal, pancreatic, colorectal, gastric, and liver cancer. These five major types of GI cancer represent 26% of the global cancer incidence and 35% of all cancer related deaths in 2018 (1). A hallmark of GI cancers, especially pancreatic cancer, is the dense and complex stromal tumor microenvironment where cancer-associated fibroblasts (CAFs) are the predominant stromal cell type (2). They comprise highly heterogeneous subpopulations with diverse and sometimes opposing functions, ranging from tumor-promoting to tumor-restraining roles (3). CAFs contribute to the reprogramming of the immune microenvironment, thereby facilitating and promoting cancer proliferation, migration, invasion, as well as metastasis (4,5). Given their influence on tumor progression, CAFs have been proposed as therapeutic target in GI cancers, especially in pancreatic cancer. However, the depletion of α-smooth muscle actin [(α-SMA), a major CAF marker]-positive cells in pre-clinical models of pancreatic cancer has paradoxically led to invasive, undifferentiated tumors and reduces animal survival, highlighting the complexity of targeting CAFs due to their functional heterogeneity (6). As a result, identification and specific targeting of tumor-promoting CAF subtypes and markers are emerging strategies (7). To identify and characterize CAF subtypes, a number of markers have been identified, such as desmin, fibroblast-activation protein (FAP), fibroblast-specific protein (FSP), podoplanin (PDPN), α-SMA, and vimentin (8,9).
FAP, a type II trans-membrane serine protease, which shares high sequential similarity with the dipeptidyl peptidase (DPP) 4, is particular interest (10). Although expression of FAP is typically low to undetectable in most adult tissues, it becomes markedly upregulated in multiple types of cancer, and it is predominantly observed in CAFs (11). In a pre-clinical study, it has been shown that FAP activates macrophages and exacerbates liver inflammation and fibrosis (12). FAP plays a key role in promoting tumor progression and metastasis, further shaping the immunosuppressive tumor microenvironment (13). Inhibition or deletion of FAP-positive CAFs in pancreatic cancer models has been associated with reduced tumor growth and improved survival (14,15). Recent findings further differentiate the roles of tumor-promoting FAP-positive CAFs from tumor-restraining α-SMA-positive CAFs, emphasizing the need for precise targeting strategies (15).
To assess the currently available evidence on the impact of FAP expression on survival and clinical characteristics in GI cancers, we performed a systematic review and meta-analysis. We present this article in accordance with the PRISMA reporting checklist (available at https://jgo.amegroups.com/article/view/10.21037/jgo-24-374/rc) (16).
Methods
The study has been registered in the PROSPERO (International Prospective Register of Systematic Reviews) database (CRD42022372194) (17). The protocol of this meta-analysis was published a priori (18).
The inclusion criteria were defined according to the Population, Intervention, Comparison, Outcomes and Study (PICOS) principles. The population consisted of adults with GI cancers exhibiting FAP overexpression. The intervention was defined as diagnostic methods utilizing FAP. The comparator were patients without FAP overexpression. The outcome measures included postoperative survival, histological differentiation, local tumor invasion, lymph node metastases, and distant metastases. The included study designs were randomized controlled trials (RCTs), cohort studies, case-control studies, and cross-sectional studies relevant to FAP in GI cancers.
Search strategy
The databases PubMed/MEDLINE, Web of Science Core Collection, Cochrane Library, and ClinicalTrials.gov were searched via their respective online search engines. Citavi 6 (Swiss Academic Software GmbH) was used as an automatic deduplication system for the studies retrieved from the several databases (Figure 1). The search was performed on studies published until December 29, 2022. The search strategies used in each database are displayed in Appendix 1. Titles and abstracts were evaluated independently in a standardized manner by two authors (A.R. and Y.S.) to assess eligibility for inclusion. All the potential studies identified from the search were coded as either “retrieve” (eligible, potentially eligible, or unclear) or “do not retrieve”. For studies coded “retrieve”, two reviewers (A.R. and Y.S.) independently screened the full text and recommended inclusion or exclusion. Disagreements between the reviewers was resolved by consensus; if no agreement was reached, a third reviewer (R.B.) decided whether to include the respective study. The reference lists of the included studies were manually searched to find additional relevant articles.
Inclusion and exclusion criteria
Only articles in English were considered. Studies comparing patients with and without FAP overexpression (regardless of the specific cutoff value used) in GI tumors and reporting on at least one of the following a priori defined outcomes were included: postoperative survival (overall and median survival, 1-, 2-, 3-, and 5-year survival rates), histological differentiation (grading), local tumor invasion (as defined in the included studies), lymph node metastases, and distant metastases. Review articles, case reports, case series with less than five patients, commentaries, and letters were not included. Details of the study selection process were summarized in a flowchart according to the recommendations of the PRISMA 2020 statement.
Data collection
Data from the included studies were extracted separately by two authors (Y.S. and A.R.) and stored in a dedicated database. The following descriptive data were documented for each selected study: first author, year of publication, inclusion period, country/region and city where the study was conducted, sample size, and mean or median follow-up time. The distribution of the following patient characteristics was documented: tumor type, histopathological tumor stage [using the Union for International Cancer Control (UICC) tumor, node, metastasis, grade (TNMG) classification system], presence and type of neoadjuvant therapy, presence and type of adjuvant therapy, FAP detection method, FAP antibody, FAP location, number of FAP-positive cases, and cutoff for overexpression. The following predefined outcomes were extracted: postoperative survival (overall and median survival, 1-, 2-, 3-, and 5-year survival rates), histological differentiation (grading), local tumor invasion (as defined in the included studies), lymph node metastases, and distant metastases. Subgroup analysis was performed for location of FAP expression (tumor stroma or tumor cells or both) and tumor type (esophageal cancer, pancreatic cancer, gastric cancer, colorectal cancer, hepatocellular carcinoma, and cholangiocellular carcinoma).
For each observational study, the risk of bias was assessed using the risk of bias in non-randomized studies of exposure (ROBINS-E) tool (19).
Statistical analysis
A meta-analysis comparing patients with and without FAP overexpression with the following outcomes was conducted: postoperative survival (overall and median survival, 1-, 2-, 3-, and 5-year survival rates), histological differentiation (grading), local tumor invasion (as defined in the included studies), lymph node metastases, and distant metastases. Time-to-event outcomes were analyzed using hazard ratios (HRs) with 95% confidence intervals (CIs). For estimating pooled overall effects, we used both random- and common-effect models. The Review Manager (RevMan) software (version 5.4; Cochrane Collaboration) was used. The magnitude of the effect estimate was visualized by forest plots. Odds ratios (ORs) were calculated for binary data, and weighted mean differences and relative standard deviation were determined for continuous data. For calculating ORs, random-effect models were used. The 95% CI, heterogeneity, and statistical significance were reported for each outcome. The Chi-squared test and degrees of freedom (df) were used to evaluate heterogeneity and statistical significance. A P value of <0.05 was considered statistically significant. Heterogeneity was presented as I2. I2>75% was defined as “considerable heterogeneity” (https://training.cochrane.org/handbook/current/chapter-10). If the study reported overall survival (OS) in the form of a Kaplan-Meier estimate and no specific data were available, then the graphically presented data were extracted using the Enguage Digitizer 12.1 (https://github.com/markummitchell/engauge-digitizer), and the HR and standard error (SE) was calculated using a spreadsheet designed by Tierney (20). For sensitivity analyses, all studies with a high or serious risk of bias were excluded, and the analyses of the outcomes, as described above, were conducted.
Results
Thirty-one cohort studies [4 studies on esophageal cancer (21-24), 8 studies on pancreatic cancer (25-32), 9 studies on colorectal cancer (33-41), 7 studies on gastric cancer (42-48), 2 studies on hepatocellular carcinoma (49,50), and 1 study on cholangiocarcinoma (51)] from 6 countries published between 2007 and 2022, were included in the meta-analysis (Figure 1). The enrolment periods of these studies ranged from 1981 to 2018. In these studies, a total of 3,976 patients (2,114 with FAP overexpression and 1,862 without overexpression) were included. The study features, patient characteristics, follow-up and outcomes are presented in table available at https://cdn.amegroups.cn/static/public/jgo-24-374-1.xlsx.
No subgroup analysis was conducted regarding the location of FAP expression due to insufficient information provided. Additionally, a meta-analysis could not be performed for histological differentiation (grading) and local tumor invasion due to inadequate information or heterogeneous definitions provided. Regarding OS, patients with FAP overexpression experienced a poorer outcome than those without overexpression (HR =1.74; 95% CI: 1.51–2.01; heterogeneity χ230=79.94; P<0.001; I2=62%) (Figure 2). This result persisted within specific cancer subgroups: esophageal cancer (HR =2.06; 95% CI: 1.53–2.77; heterogeneity χ23=6.41; P=0.09; I2=53%), pancreatic cancer (HR =2.06; 95% CI: 1.45–2.92; heterogeneity χ27=12.76; P=0.08; I2=45%), colorectal cancer (HR =1.53; 95% CI: 1.18–1.97; heterogeneity χ28=17.25; P=0.03; I2=54%), and gastric cancer (HR =1.85; 95% CI: 1.48–2.32; heterogeneity χ26=2.4; P=0.88; I2=0%). For the subgroup hepatocellular carcinoma there was no significant association between FAP overexpression and survival. In addition, the data was highly heterogeneous (HR =1.32; 95% CI: 0.33–5.22; heterogeneity χ21=7.01; P=0.008; I2=86%). We did not conduct a sensitivity analysis for the subgroup hepatocellular carcinoma, since we identified only two studies. Notably, only the study conducted by Byrling in 2020 investigated cholangiocarcinoma, reporting that patients without FAP overexpression exhibited shorter survival (HR =0.74; 95% CI: 0.40–1.36) (Figure 3). The range of median overall survival (mOS) for patients with overexpression and without overexpression was 10.0 to 125.9 months and 16.6 to 81.5 months, respectively. Analyzing the available dichotomous survival data, identical 1-year survival rates were noted in patients with esophageal cancer between the overexpression and no overexpression groups (data from 4 studies, 78% vs. 80%, OR =0.93; 95% CI: 0.56–1.53; P=0.76). In the subgroup of pancreatic cancer, lower 1-year survival rates were evident in the overexpression group (data from five studies, 59% vs. 73%, OR =0.66; 95% CI: 0.45–0.97; P=0.03). Similarly, lower 2- and 3-year survival rates were noted in the overexpression group for both esophageal and pancreatic cancer (41% vs. 60%, OR =0.62, 95% CI: 0.47–0.81, P<0.05; 25% vs. 57%, OR =0.25, 95% CI: 0.18–0.35, P<0.05). It is noteworthy that 5-year survival rates were higher in the FAP overexpression group for both subgroups (39% vs. 34%, OR =1.71; 95% CI: 1.26–2.32, P<0.05) (Figures S1-S4).
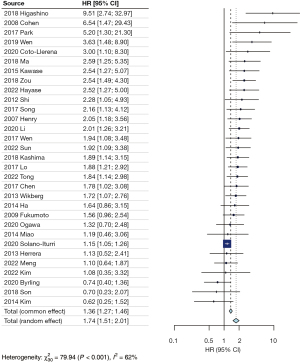
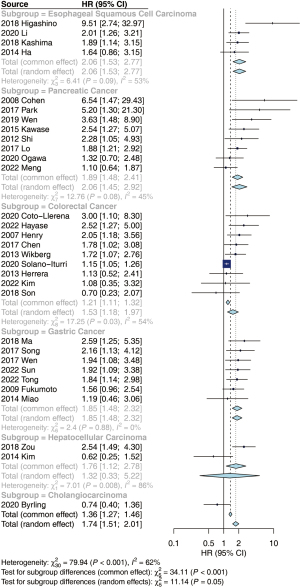
Regarding lymph node metastases, a higher frequency was evident in the FAP overexpression group compared to the non-overexpression group (56% vs. 44%, OR =1.98; 95% CI: 1.59–2.46; P<0.05) (Figure 4). In terms of distant metastases, there was no difference between the two groups (11% vs. 12%, OR =0.92; 95% CI: 0.51–1.64; P=0.77).
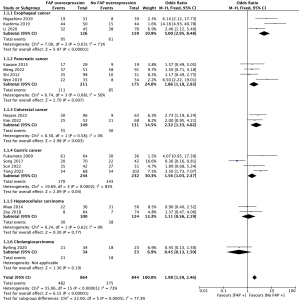
ROBINS-E bias analysis revealed that none of 31 studies systematically controlled confounding and adjusted data via e.g., propensity score matching, so that all studies were categorized as “high risk of bias” for the domain “risk of bias due to confounding” (Figure 5). Currently, there is no evidence identifying potential confounding factors for FAP expression. As all 31 studies are observational and not RCTs, acquiring comprehensive data on confounding, including all unknown factors, is unattainable. Yet, several studies stratified FAP expression status and analyzed several potential confounders such as age or gender, and no study detected a notable difference in age or gender between FAP overexpression and no overexpression groups (21-24,26-28,31,32,34,35,38,41-43,45-47,49-51). These studies were categorized as “low risk of bias” for the domain “risk of bias due to missing data”, and the other studies were categorized as “some concerns”. All 31 studies were categorized “some concerns” for the domain “risk of bias in selection of participants into the study”. General selection bias is discussed in the discussion section. For domains “risk of bias arising from measurement of exposure”, “risk of bias due to post-exposure interventions”, “risk of bias arising from measurement of outcomes”, and “risk of bias in selection of the reported result”, all studies were categorized as “low risk of bias” (Figure 5).
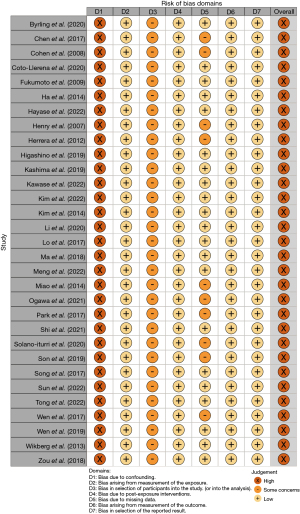
Discussion
Increasing evidence suggests that the tumor microenvironment and CAFs, a pivotal component of the tumor stroma, play critical roles in facilitating cancer progression, metastasis, drug resistance, and immunosuppression in many types of cancer including GI cancer (52). CAFs can originate from diverse types of cells such as adipocytes and mesenchymal stem cells (3). CAFs are a highly heterogeneous group of cells and exhibit high cellular plasticity (3). Therefore, identification of tumor-promoting CAF subtypes and specific markers are emerging to develop precise targeting strategies. FAP has been considered to label tumor-promoting CAF subtypes and to play a key role in promoting tumor progression and metastasis, further shaping the immunosuppressive tumor microenvironment (13). In the current study, we conducted a systematic review and meta-analysis concerning FAP overexpression in GI cancers and observed that patients without FAP overexpression experienced a favorable outcome regarding OS compared to patients with FAP overexpression. Several studies on this topic have been published and the last meta-analysis published in 2015 included 15 studies with various cancer types. Eight studies involving 1,277 patients with GI cancers were included in this meta-analysis (2 esophageal, 1 gastric, 2 pancreatic, and 3 colorectal cancer). It reported an association between FAP overexpression and shorter OS in pancreatic cancer (HR =3.18; 95% CI: 1.42–7.12) and colorectal cancer (HR =1.72; 95% CI: 1.14–2.60) (53). In our meta-analysis, we identified 8 pancreatic and 9 colorectal cancer cohort studies, for which we demonstrated an association between FAP overexpression and shorter OS. We further observed that patients with esophageal cancer and gastric cancer who showed FAP overexpression experienced a shorter survival compared to those without overexpression. We identified only one study, in patients with cholangiocarcinoma, which yielded inconclusive results.
FAP has been considered one of the most relevant CAF markers. The question of whether FAP can be a viable target in the treatment of GI cancers is still not fully understood and requires further investigation. A pre-clinical study showed that stromal FAP promotes intrahepatic cholangiocarcinoma growth via chemokine CCL2 expression and recruitment of monocyte-derived suppressor cells (MDSCs) (54). Consistently, the chemokine-mediated immunosuppressive and tumor-promoting role of FAP-positive CAFs has been demonstrated in pancreatic cancer and liver cancer (55,56). These data suggest a tumor-promoting role of FAP-positive CAFs by expressing chemokines and establishing an immunosuppressive tumor microenvironment. On the other hand, several studies have shown the organ-specific variations of transcriptional fingerprints in CAFs, especially for hepatic and pancreatic fibroblasts/CAFs (57,58). Therefore, we cannot exclude the possibility that FAP-positive CAFs in addition co-express organ-specific CAF markers, leading to functional diversity and disparity of FAP-positive CAFs between different GI tumor entities. To clarify this issue, it is necessary to conduct single nuclear RNA sequencing and compare gene expression profiling in CAFs from different GI tumor entities in the future.
The present study has some limitations. First, the cutoff values to define FAP overexpression and the antibodies employed for immunohistochemistry were heterogeneous between the single studies. This can increase the variation in results of the individual studies and make their interpretation difficult. Second, the studies recruited only GI cancer patients and no control cohort. The inclusion of all GI cancer subtypes might have caused clinical heterogeneity, which we aimed to reduce by also conducting meta-analyses in subgroups of tumor subtype. Third, all included studies are observational and retrospective, and selection bias is substantial. The research has primarily focused on individuals who underwent surgical procedures, potentially skewing the participant pool. Moreover, a noteworthy imbalance is evident in the geographical distribution of the studies, with 23 out of 31 conducted in Asian countries. This disparity introduces the possibility of additional selection bias, as cultural and societal variations could influence the outcomes. Furthermore, a critical observation arises from the fact that none of the 31 studies systematically addressed confounding variables or adjusted data using established methods such as propensity score matching. The absence of rigorous control measures raises concerns about the reliability and validity of the findings, emphasizing the need for future research to employ more robust methodologies. Pre-clinical studies have demonstrated that pharmacological inhibition or deletion of FAP-positive CAFs results in attenuation of tumor growth and increased survival in pancreatic cancer models (14,15). However, further studies are needed to prove whether FAP overexpression has causal effects in GI cancers in general.
Our study re-evaluates and highlights the possible importance of FAP and CAFs in GI tumors. In the future, it is important to design and establish studies to evaluate the clinical significance of FAP targeting strategies with e.g., novel selective FAP inhibitors, such as FAPI-04, FAPI-46 (59), FAPI-74 (60) as well as 3BP-3940 (61), molecular imaging with 68Ga-FAPI-positron emission tomography/computed tomography (PET/CT) (59), and chimeric antigen receptor (CAR) T cells therapy (62).
Conclusions
In the current study, we showed that patients without FAP overexpression exhibited a favorable OS. Subgroup analyses revealed consistent results across esophageal, pancreatic, colorectal, and gastric cancers. This meta-analysis underscores the potential adverse prognostic significance of FAP expression in GI cancers. The variability in FAP expression and its impact on survival across different GI cancers calls for further investigation into its role and the development of selective FAP inhibitors or targeted therapies. Future research should focus on delineating the precise roles and clinical implications of FAP in GI cancers. Standardization in measuring FAP expression and controlling for confounding factors in studies are critical steps to improve the reliability of research in this area.
Acknowledgments
The study was in part presented at the 42nd Congress of the German Pancreatic Club (March 4, 2023, Munich, Germany), at the 16th Congress of the International Hepato-Pancreato-Biliary Association (May 16, 2024, Cape Town, South Africa), and at the 56th Congress of the European Pancreatic Club (June 27, 2024, Santiago de Compostela, Spain).
Funding: None.
Footnote
Reporting Checklist: The authors have completed the PRISMA reporting checklist. Available at https://jgo.amegroups.com/article/view/10.21037/jgo-24-374/rc
Peer Review File: Available at https://jgo.amegroups.com/article/view/10.21037/jgo-24-374/prf
Conflicts of Interest: All authors have completed the ICMJE uniform disclosure form (available at https://jgo.amegroups.com/article/view/10.21037/jgo-24-374/coif). The authors have no conflicts of interest to declare.
Ethical Statement: The authors are accountable for all aspects of the work in ensuring that questions related to the accuracy or integrity of any part of the work are appropriately investigated and resolved.
Open Access Statement: This is an Open Access article distributed in accordance with the Creative Commons Attribution-NonCommercial-NoDerivs 4.0 International License (CC BY-NC-ND 4.0), which permits the non-commercial replication and distribution of the article with the strict proviso that no changes or edits are made and the original work is properly cited (including links to both the formal publication through the relevant DOI and the license). See: https://creativecommons.org/licenses/by-nc-nd/4.0/.
References
- Arnold M, Abnet CC, Neale RE, et al. Global Burden of 5 Major Types of Gastrointestinal Cancer. Gastroenterology 2020;159:335-349.e15. [Crossref] [PubMed]
- Sunami Y, Häußler J, Zourelidis A, et al. Cancer-Associated Fibroblasts and Tumor Cells in Pancreatic Cancer Microenvironment and Metastasis: Paracrine Regulators, Reciprocation and Exosomes. Cancers (Basel) 2022;14:744. [Crossref] [PubMed]
- Sunami Y, Häußler J, Kleeff J. Cellular Heterogeneity of Pancreatic Stellate Cells, Mesenchymal Stem Cells, and Cancer-Associated Fibroblasts in Pancreatic Cancer. Cancers (Basel) 2020;12:3770. [Crossref] [PubMed]
- Sun Y, Wang R, Qiao M, et al. Cancer associated fibroblasts tailored tumor microenvironment of therapy resistance in gastrointestinal cancers. J Cell Physiol 2018;233:6359-69. [Crossref] [PubMed]
- Mao X, Xu J, Wang W, et al. Crosstalk between cancer-associated fibroblasts and immune cells in the tumor microenvironment: new findings and future perspectives. Mol Cancer 2021;20:131. [Crossref] [PubMed]
- Özdemir BC, Pentcheva-Hoang T, Carstens JL, et al. Depletion of carcinoma-associated fibroblasts and fibrosis induces immunosuppression and accelerates pancreas cancer with reduced survival. Cancer Cell 2014;25:719-34. [Crossref] [PubMed]
- Sunami Y, Böker V, Kleeff J. Targeting and Reprograming Cancer-Associated Fibroblasts and the Tumor Microenvironment in Pancreatic Cancer. Cancers (Basel) 2021;13:697. [Crossref] [PubMed]
- Kalluri R. The biology and function of fibroblasts in cancer. Nat Rev Cancer 2016;16:582-98. [Crossref] [PubMed]
- Pereira BA, Vennin C, Papanicolaou M, et al. CAF Subpopulations: A New Reservoir of Stromal Targets in Pancreatic Cancer. Trends Cancer 2019;5:724-41. [Crossref] [PubMed]
- Fitzgerald AA, Weiner LM. The role of fibroblast activation protein in health and malignancy. Cancer Metastasis Rev 2020;39:783-803. [Crossref] [PubMed]
- Puré E, Blomberg R. Pro-tumorigenic roles of fibroblast activation protein in cancer: back to the basics. Oncogene 2018;37:4343-57. [Crossref] [PubMed]
- Yang AT, Kim YO, Yan XZ, et al. Fibroblast Activation Protein Activates Macrophages and Promotes Parenchymal Liver Inflammation and Fibrosis. Cell Mol Gastroenterol Hepatol 2023;15:841-67. [Crossref] [PubMed]
- Han C, Liu T, Yin R. Biomarkers for cancer-associated fibroblasts. Biomark Res 2020;8:64. [Crossref] [PubMed]
- Santos AM, Jung J, Aziz N, et al. Targeting fibroblast activation protein inhibits tumor stromagenesis and growth in mice. J Clin Invest 2009;119:3613-25. [Crossref] [PubMed]
- McAndrews KM, Chen Y, Darpolor JK, et al. Identification of Functional Heterogeneity of Carcinoma-Associated Fibroblasts with Distinct IL6-Mediated Therapy Resistance in Pancreatic Cancer. Cancer Discov 2022;12:1580-97. [Crossref] [PubMed]
- Page MJ, McKenzie JE, Bossuyt PM, et al. The PRISMA 2020 statement: an updated guideline for reporting systematic reviews. BMJ 2021;372: [PubMed]
. Available online: https://www.crd.york.ac.uk/prospero/PROSPERO - Sunami Y, Ronellenfitsch U, Kleeff J, et al. Fibroblast Activation Protein Overexpression in Gastrointestinal Tumors: Protocol for a Systematic Review and Meta-analysis. JMIR Res Protoc 2023;12:e45176. [Crossref] [PubMed]
- ROBINS-E Development Group. Risk Of Bias In Non-randomized Studies - of Exposure (ROBINS-E). Launch version, 20 June 2023. Available online: https://www.riskofbias.info/welcome/robins-e-tool
- Tierney JF, Stewart LA, Ghersi D, et al. Practical methods for incorporating summary time-to-event data into meta-analysis. Trials 2007;8:16. [Crossref] [PubMed]
- Ha SY, Yeo SY, Xuan YH, et al. The prognostic significance of cancer-associated fibroblasts in esophageal squamous cell carcinoma. PLoS One 2014;9:e99955. [Crossref] [PubMed]
- Higashino N, Koma YI, Hosono M, et al. Fibroblast activation protein-positive fibroblasts promote tumor progression through secretion of CCL2 and interleukin-6 in esophageal squamous cell carcinoma. Lab Invest 2019;99:777-92. [Crossref] [PubMed]
- Kashima H, Noma K, Ohara T, et al. Cancer-associated fibroblasts (CAFs) promote the lymph node metastasis of esophageal squamous cell carcinoma. Int J Cancer 2019;144:828-40. [Crossref] [PubMed]
- Li F, Wu X, Sun Z, et al. Fibroblast Activation Protein-α Expressing Fibroblasts Promote Lymph Node Metastasis in Esophageal Squamous Cell Carcinoma. Onco Targets Ther 2020;13:8141-8. [Crossref] [PubMed]
- Cohen SJ, Alpaugh RK, Palazzo I, et al. Fibroblast activation protein and its relationship to clinical outcome in pancreatic adenocarcinoma. Pancreas 2008;37:154-8. [Crossref] [PubMed]
- Kawase T, Yasui Y, Nishina S, et al. Fibroblast activation protein-α-expressing fibroblasts promote the progression of pancreatic ductal adenocarcinoma. BMC Gastroenterol 2015;15:109. [Crossref] [PubMed]
- Lo A, Li CP, Buza EL, et al. Fibroblast activation protein augments progression and metastasis of pancreatic ductal adenocarcinoma. JCI Insight 2017;2:e92232. [Crossref] [PubMed]
- Meng Y, Yu J, Zhu M, et al. CT radiomics signature: a potential biomarker for fibroblast activation protein expression in patients with pancreatic ductal adenocarcinoma. Abdom Radiol (NY) 2022;47:2822-34. [Crossref] [PubMed]
- Ogawa Y, Masugi Y, Abe T, et al. Three Distinct Stroma Types in Human Pancreatic Cancer Identified by Image Analysis of Fibroblast Subpopulations and Collagen. Clin Cancer Res 2021;27:107-19. [Crossref] [PubMed]
- Park H, Lee Y, Lee H, et al. The prognostic significance of cancer-associated fibroblasts in pancreatic ductal adenocarcinoma. Tumour Biol 2017;39:1010428317718403. [Crossref] [PubMed]
- Shi M, Yu DH, Chen Y, et al. Expression of fibroblast activation protein in human pancreatic adenocarcinoma and its clinicopathological significance. World J Gastroenterol 2012;18:840-6. [Crossref] [PubMed]
- Wen Z, Liu Q, Wu J, et al. Fibroblast activation protein α-positive pancreatic stellate cells promote the migration and invasion of pancreatic cancer by CXCL1-mediated Akt phosphorylation. Ann Transl Med 2019;7:532. [Crossref] [PubMed]
- Chen L, Qiu X, Wang X, et al. FAP positive fibroblasts induce immune checkpoint blockade resistance in colorectal cancer via promoting immunosuppression. Biochem Biophys Res Commun 2017;487:8-14. [Crossref] [PubMed]
- Coto-Llerena M, Ercan C, Kancherla V, et al. High Expression of FAP in Colorectal Cancer Is Associated With Angiogenesis and Immunoregulation Processes. Front Oncol 2020;10:979. [Crossref] [PubMed]
- Hayase S, Miyoshi N, Fujino S, et al. Fibroblast Activation Protein and Tertiary Lymphoid Structure in Colorectal Cancer Recurrence. Anticancer Res 2022;42:5897-907. [Crossref] [PubMed]
- Henry LR, Lee HO, Lee JS, et al. Clinical implications of fibroblast activation protein in patients with colon cancer. Clin Cancer Res 2007;13:1736-41. [Crossref] [PubMed]
- Herrera M, Herrera A, Domínguez G, et al. Cancer-associated fibroblast and M2 macrophage markers together predict outcome in colorectal cancer patients. Cancer Sci 2013;104:437-44. [Crossref] [PubMed]
- Kim S, Yeo MK, Kim JS, et al. Elevated CXCL12 in the plasma membrane of locally advanced rectal cancer after neoadjuvant chemoradiotherapy: a potential prognostic marker. J Cancer 2022;13:162-73. [Crossref] [PubMed]
- Solano-Iturri JD, Beitia M, Errarte P, et al. Altered expression of fibroblast activation protein-α (FAP) in colorectal adenoma-carcinoma sequence and in lymph node and liver metastases. Aging (Albany NY) 2020;12:10337-58. [Crossref] [PubMed]
- Son GM, Kwon MS, Shin DH, et al. Comparisons of cancer-associated fibroblasts in the intratumoral stroma and invasive front in colorectal cancer. Medicine (Baltimore) 2019;98:e15164. [Crossref] [PubMed]
- Wikberg ML, Edin S, Lundberg IV, et al. High intratumoral expression of fibroblast activation protein (FAP) in colon cancer is associated with poorer patient prognosis. Tumour Biol 2013;34:1013-20. [Crossref] [PubMed]
- Fukumoto Y, Yamada Y, Fukuda K, et al. Clinical Findings on Fibroblast Activation Protein in Patients with Gastric Cancer. Yonago Acta Medica 2009;52:21-5.
- Ma Y, Zhu J, Chen S, et al. Activated gastric cancer-associated fibroblasts contribute to the malignant phenotype and 5-FU resistance via paracrine action in gastric cancer. Cancer Cell Int 2018;18:104. [Crossref] [PubMed]
- Miao ZF, Zhao TT, Wang ZN, et al. Tumor-associated mesothelial cells are negative prognostic factors in gastric cancer and promote peritoneal dissemination of adherent gastric cancer cells by chemotaxis. Tumour Biol 2014;35:6105-11. [Crossref] [PubMed]
- Song H, Liu QY, Huang ZW. High expression of fibroblast activation protein is an adverse prognosticator in gastric cancer. Biomedical Research 2017;28:7779-83.
- Sun H, Wang X, Zhang X, et al. Multiplexed immunofluorescence analysis of CAF-markers, EZH2 and FOXM1 in gastric tissue: associations with clinicopathological parameters and clinical outcomes. BMC Cancer 2022;22:1188. [Crossref] [PubMed]
- Tong Y, Zhao Z, Zhang J, et al. High expressions of CD10, FAP and GPR77 in CAFs are associated with chemoresistance and worse prognosis in gastric cancer. Front Oncol 2022;12:984817. [Crossref] [PubMed]
- Wen X, He X, Jiao F, et al. Fibroblast Activation Protein-α-Positive Fibroblasts Promote Gastric Cancer Progression and Resistance to Immune Checkpoint Blockade. Oncol Res 2017;25:629-40. [Crossref] [PubMed]
- Kim GJ, Rhee H, Yoo JE, et al. Increased expression of CCN2, epithelial membrane antigen, and fibroblast activation protein in hepatocellular carcinoma with fibrous stroma showing aggressive behavior. PLoS One 2014;9:e105094. [Crossref] [PubMed]
- Zou B, Liu X, Zhang B, et al. The Expression of FAP in Hepatocellular Carcinoma Cells is Induced by Hypoxia and Correlates with Poor Clinical Outcomes. J Cancer 2018;9:3278-86. [Crossref] [PubMed]
- Byrling J, Sasor A, Nilsson J, et al. Expression of fibroblast activation protein and the clinicopathological relevance in distal cholangiocarcinoma. Scand J Gastroenterol 2020;55:82-9. [Crossref] [PubMed]
- Asif PJ, Longobardi C, Hahne M, et al. The Role of Cancer-Associated Fibroblasts in Cancer Invasion and Metastasis. Cancers (Basel) 2021;13:4720. [Crossref] [PubMed]
- Liu F, Qi L, Liu B, et al. Fibroblast activation protein overexpression and clinical implications in solid tumors: a meta-analysis. PLoS One 2015;10:e0116683. [Crossref] [PubMed]
- Lin Y, Li B, Yang X, et al. Fibroblastic FAP promotes intrahepatic cholangiocarcinoma growth via MDSCs recruitment. Neoplasia 2019;21:1133-42. [Crossref] [PubMed]
- Feig C, Jones JO, Kraman M, et al. Targeting CXCL12 from FAP-expressing carcinoma-associated fibroblasts synergizes with anti-PD-L1 immunotherapy in pancreatic cancer. Proc Natl Acad Sci U S A 2013;110:20212-7. [Crossref] [PubMed]
- Yang X, Lin Y, Shi Y, et al. FAP Promotes Immunosuppression by Cancer-Associated Fibroblasts in the Tumor Microenvironment via STAT3-CCL2 Signaling. Cancer Res 2016;76:4124-35. [Crossref] [PubMed]
- Buchholz M, Kestler HA, Holzmann K, et al. Transcriptome analysis of human hepatic and pancreatic stellate cells: organ-specific variations of a common transcriptional phenotype. J Mol Med (Berl) 2005;83:795-805. [Crossref] [PubMed]
- Erkan M, Weis N, Pan Z, et al. Organ-, inflammation- and cancer specific transcriptional fingerprints of pancreatic and hepatic stellate cells. Mol Cancer 2010;9:88. [Crossref] [PubMed]
- Chandekar KR, Prashanth A, Vinjamuri S, et al. FAPI PET/CT Imaging-An Updated Review. Diagnostics (Basel) 2023;13:2018. [Crossref] [PubMed]
- Lang M, Spektor AM, Hielscher T, et al. Static and Dynamic 68Ga-FAPI PET/CT for the Detection of Malignant Transformation of Intraductal Papillary Mucinous Neoplasia of the Pancreas. J Nucl Med 2023;64:244-51. [Crossref] [PubMed]
- Greifenstein L, Gunkel A, Hoehne A, et al. 3BP-3940, a highly potent FAP-targeting peptide for theranostics - production, validation and first in human experience with Ga-68 and Lu-177. iScience 2023;26:108541. [Crossref] [PubMed]
- Xiao Z, Todd L, Huang L, et al. Desmoplastic stroma restricts T cell extravasation and mediates immune exclusion and immunosuppression in solid tumors. Nat Commun 2023;14:5110. [Crossref] [PubMed]