Genetics and molecular pathology of gastric malignancy: Development of targeted therapies in the era of personalized medicine
Introduction
Personalized medicine is a medical model proposing the customization of healthcare, with decisions and practices being tailored to the individual patient by use of genetic or other information. Under the present paradigm personalized medicine offers a glimpse at the future of medicine. As a result, a new issue arises: The best for some or for all? Will this new model of medicine be an instrument for the few or the many? Molecular pathology, an initially expensive yet powerful tool in the post-genomic medical armatorium, lies at the crux of this issue. It offers physicians the ability to customize therapy for the individual patient based on his or her unique molecular pathological process. Defining the unique subsets of patients that can gain benefit from specific and expensive therapuetic agents is critical in both providing high quality care and cost-effective medicine. Globally, gastric cancer is the second most common cause of cancer-related death, with the majority of the health burden borne by economically less-developed countries (1). Here we review the prospects from genetics and beyond in regard to targeted molecular therapies for three common gastric malignancies.
Molecular pathology of gastric adenocarcinoma
Gastric cancer is the second most common cause of cancer death worldwide (2). The incidence of gastric adenocarcinoma has been declining for decades; however its prognosis remains poor (3). Epidemiological studies have shown that environmental factors such as Helicobacter pylori, diet, and smoking play a significant role in gastric carcinogenesis (4). However, host genetics are thought to contribute as well. For example, although H. pylori infection is known to be associated with an increased risk of gastric cancer, the risk is much higher in subgroups of infected patients who have atrophic gastritis and extensive intestinal metaplasia, suggesting that host genetics influence how often precancerous lesions appear in H. pylori-infected individuals (5).
There are two distinct types of gastric adenocarcinoma, intestinal (well-differentiated) and diffuse (undifferentiated), which have distinct morphologic appearance, epidemiology, pathogenesis, and genetic profiles (6,7). A molecular basis for this difference is now apparent (6). The morphologic differences are attributable to intercellular adhesion molecules, which are well preserved in intestinal-type tumors and defective in diffuse carcinomas.
The main carcinogenic event in diffuse carcinomas is loss of expression of E-cadherin, a key cell surface protein for establishing intercellular connections and maintaining the organization of epithelial tissues. Biallelic inactivation of the gene encoding E-cadherin, CDH1, can occur through germline or somatic mutation, allelic imbalance events (e.g., loss of heterozygosity), or epigenetic silencing of gene transcription through aberrant methylation of the CDH1 promoter. Approximately 10-15% of gastric cancers are familial. Hereditary diffuse gastric cancer, a highly penetrant autosomal dominant condition, is caused by germline mutations in the epithelial cadherin gene and is characterized by an increased risk for diffuse gastric cancer and lobular breast cancer (2). Approximately one third of families have inactivating mutations in the epithelial cadherin gene (2). Other cancer syndromes also display an increased risk in gastric cancer, such as, hereditary nonpolyposis colon cancer (HNPCC), familial adenomatous polyposis (FAP), Peutz-Jegher’s syndrome and BRAC2 mutation carriers (Figure 1) (2).
HER2 gene amplification and overexpression has been well recognized as a strong driver of carcinogenesis, especially in breast cancer. Increasing evidence has shown that HER2 amplification is also involved in a substantial number of gastric cancers, up to 34% (1). Moreover, treatment with tratuzumab increased survival benefits in patients with cancers that had high HER2-expression (8). HER2 testing in gastric cancer differs from HER2 testing in breast cancer (1). Gastric cancer more often display heterogeneous incomplete focal membrane staining. Histological differences between gastric and breast cancers necessitate modifications to the HER2 scoring system for gastric cancer. Gastric cancer-specific HER2 testing protocols have been developed and standardized. Immunohistochemistry is the initial testing methodology followed by fluorescence in-situ hybridization or silver in-situ hybridization in immunohistochemically 2+ equivocal cases. Using the scoring criteria for HER2 established in breast cancer on gastric cancer cases may underscore tumors by as much as 50% compared with the cases scored in the trastuzumab for gastric cancer trial; thus, preventing eligible patients access to effective therapy (9). Biopsies are the preferred specimen for optimal results. The scoring criteria for HER2 immunohistochemical testing in gastric cancer are summarized (Table 1, Figures 2,3).
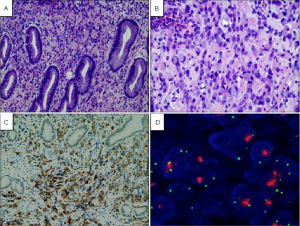
HER2 testing in gastric carcinoma opens a new promising therapeutic option for patients. The progress in molecular pathology enables understanding the biology of gastric and GEJ cancer and in discovering possible novel molecular therapy targets. These therapeutic strategies include epidermal growth factor receptor inhibitors, antiangiogenic agents, cell cycle inhibitors, apoptosis promoters, and matrix metalloproteinases inhibitors. The agents targeting the human epidermal growth factor receptor HER 2 and epidermal growth factor receptor 1 (EGFR1), vascular endothelial growth factor (VEGF), MET and regulators of cell cycle are being integrated into therapeutic studies with the goal of improving therapeutic options for this disease (10).
Molecular pathology of gastrointestinal stromaltumors
Gastrointestinal stromal tumor (GIST) is one of the most common mesenchymal tumors of the gastrointestinal tract, accounting for 80% of gastrointestinal mesenchymal tumors (10). However, they are rare with respect to all GI malignancies, as they constitute only 1-3% (10). At presentation, nearly half of malignant GISTs are metastatic, however less than a third of GISTs are classified as malignant (10).
Prior to 1998, GISTs were diagnostically problematic, being mistaken for smooth muscle tumors such as leiomyoblastomas, leiomyomas and leiomyosarcomas (11).Electron microscopy studies in the 1970s and immunohistochemical studies in the late 1980s revealed that these tumors were in fact not derived from smooth muscle (11).
Rather, these studies pointed to the interstitial cells of Cajal as the cell of origin of GISTs. The interstitial cells of Cajal are the pacemaker cells of the gastrointestinal track. They regulate intestinal motility and peristalsis and are found in-between the autonomic nervous system and the muscular wall of the GI tract (11). These cells have immunophenotypic and ultrastructural features of smooth muscle and neuronal cells similar to GISTs (11). Like GISTs they stain positive by IHC for CD34, CD117, and DOG1 (Figure 4).
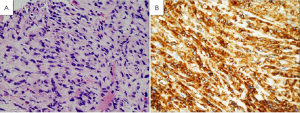
In 1998 Hirota and colleagues published a sentinel paper showing that most GISTs harbored mutations in the c-kit gene which results in ligand-independent activation of KIT protein (12). They also showed that GISTs usually express the KIT protein, using an immunohistochemistry stain c-kit or CD117, providing pathologists with a critical diagnostic test (12). This sentinel discovery changed the paradigm of GISTs pathogenesis, diagnosis, and treatment. Further studies showed that a subset of GISTs contain mutations in another tyrosine kinase receptor gene called platelet-derived growth factor receptor (PDGFRA).
Regardless of site of involvement, most GISTs express the CD34 antigen (70-80%) and the CD117 antigen (72-94%). A relatively new immunohistochemistry marker, DOG1, which was discovered using gene expression profiling (13), is highly specific for GISTs. Negativity for both DOG1 and KIT has been observed in only 2.6% of GISTs of the gastrointestinal tract (13).
The term GIST is now generally used to specify a mesenchymal tumor of the gastrointestinal tract that contains either a KIT or PDGFRA driver mutation and displays a characteristic histology which includes spindle, epithelioid, and rarely pleomorphic cells (14).
KIT is a transmembrane tyrosine kinase receptor that plays an important role in the maturation of hematopoetic cells, melanocytes, and interstitial cells of Cajal (11). The binding of stem cell factor to the extracellular domain of the receptor results in autophosphorylation of several tyrosine residues and activation. Once activated KIT phosphorylates other proteins and transcription factors leading to activation of signal transduction cascades, such as the Ras/MAP kinase pathway (15). These activated pathways ultimately lead to several cellular modifications including changes in cell adhesion, migration, and differentiation.
KIT mutations are seen in 85% to 95% of GISTs, almost always resulting in ligand-independent activation (11). The mutations tend to cluster in 4 exons: exon 9 (extracellular domain), exon 11 (intracellular juxtamembrane domain), exon 13 (split kinase domain), and exon 17 (kinase activation loop) (11). Exon 11 mutations are the most common, representing 60% to 70% of the cases. Exon 9 mutations are present in 10% of cases and are associated with small-bowel location and a more aggressive clinical behavior. Exon 13 and 17 mutations are rare, each representing approximately 1% of GIST cases (11) (Figure 5).
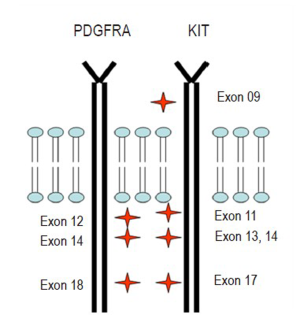
Thus far KIT and PDGFRA mutations are thought to be mutually exclusive (11). Approximately 5% to 10% of GISTs harbor PDGFRA mutations involving exons 12, 14, and 18 (11). Akin to KIT mutations, PDGFRA mutations result in ligand-independent activation (11). Almost all PDGFRA-mutant GISTs have an epithelioid morphology and are found in the stomach. CD117 expression in PDGFRA-mutant tumors is often weak and focal or entirely negative (11). Approximately 5% of GISTs do not harbor either KIT or PDGFRA mutations and yet, can still be positive for CD117 by immunohistochemistry (11). These are known as ‘‘wild-type’’ GISTs.
Most GISTs are sporadic, however, small percentages (less than 5%) do occur in the rare GIST associated tumor syndromes: neurofibromatosis type 1 (NF1), Carney triad, and familial GIST syndrome (14). From the Armed Forces Institute of Pathology (AFIP) series, 6% of duodenal GISTs belong to patients with NF1 (14). Although NF1 patients can have GISTs elsewhere, the great majority occur in the small bowel in this population. The tumors are frequently multiple, small, and indolent with a low mitotic activity. However, NF1 patients can go on to develop malignant GISTs, which can be confused with malignant schwannomas if immunohistochemical studies are not carried out. Interestingly, GISTs in NF1 patients likely have a different pathogenic pathway, since they rarely if ever have the c-kit and PDGFRA mutations as seen in sporadic GISTs (16) (Table 2).
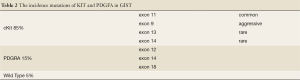
Full table
The Carney triad includes gastric GIST, paraganglioma, and pulmonary chondroma. These GISTs are usually epithelioid. They often occur in children and have a strong female predominance (85%) and the majority are indolent, even in the setting of metastatic disease (14).
Rare cases of familial GIST syndrome have been reported (14). Usually, they show autosomal dominant transmission of activating KIT or PDGFRA mutations. Patients with germline KIT or PDGFRA mutations have shown Cajal cell hyperplasia and progression to discrete GISTs (17). Tumors are typically multiple with biological behavior that varies from indolent to malignant. These individuals also develop cutaneous hyperpigmentation and mastocytosis (18). A study using PCR for clonality analysis showed that diffuse Cajal cell proliferations seen in these patients are polyclonal, whereas the GIST tumors are monoclonal (18). This suggests that additional genetic alterations are required before clonal expansion and malignant transformation can occur (14).
The therapeutic drug of choice for unresectable, metastatic, or recurrent GISTs is imatinib, a competitive antagonist of the ATP binding site of tyrosine kinases such as KIT, platelet growth factor receptors alpha and beta, ABL, and ABL-related gene product. It causes interruption of the downstream signaling process that leads to cellular proliferation. Ten to twenty percent of GISTs exhibit resistance to imatinib (10). This resistance has been associated with selection of mutations that in some cases interrupt the binding site of imatinib (19). Patients with the Kit exon 9 mutations often require a higher dose of imatinib, often double the starting dose recommended for exon 11 mutants (10). Resistance is also thought to result from secondary mutations in the KIT and/or PDGFRA kinase domain. Several other inhibitors are being developed for resistant tumors. Surgery however, remains the only curative treatment for GISTs.
Molecular pathology of gastric neuroendocrinetumors
Gastric neuroendocrine tumors are being diagnosed with higher and higher frequency than previously reported (20). Some have ascribed this to more frequent endoscopies and more accurate diagnosis with immunohistochemistry markers (21). Gastric endocrine tumors make up to 20% of all gastroentero-pancreatic neuroendocrine tumors and 1% of gastric neoplasms (22). Gastric neuroendocrine tumors are thought to be local endodermally derived cells and not neural crest derived based on studies of chick-quail chimeras (23,24). Gastric carcinoids have often been classified in a tripartite system as follows: tumors associated with chronic atrophic gastritis; tumors associated with MEN type 1, and Zollinger-Ellison syndrome; and sporadic tumors (25).
There are many classifications of the neuroendocrine tumors. An older classification scheme, divided these tumors into foregut (stomach and first part of the duodenum), midgut (small intestine: second portion of duodenum, jejunum, ileum, appendix and ascending colon) and hindgut (transverse and descending colon and rectum) (26). Molecular studies actually show that NETs of foregut, midgut, and hindgut display different genetically distinct abnormalities (27).
Foregut NETs (stomach and duodenum) show frequent loss of heterozygosity (LOH) for the MEN1 gene and is currently thought to play an initial role in gastric neuorendocrine tumor genesis in both familial and sporadic cases (26). The protein product menin, a 610-amino acid protein, is predominately nuclear and involved in transcription regulation, genome stability and cell division (Figure 6) (28).
The WHO classification of endocrine tumors has divided NET into well differentiated endocrine tumors (benign or uncertain behavior), well differentiated endocrine carcinomas (low-grade malignant behavior) and poorly differentiated endocrine carcinoma (high-grade malignant behavior) (29). Studies have shown that malignant progression of NET is associated with complex allelotypes and chromosomal instability (30).
Interestingly, one study showed that 8 of 11 diffuse gastric cancer cases with signet ring cells express one or more neuroendocrine markers, a finding previously thought to be rare, showing that the greater proportion of signet ring cancer cells express specific general neuroendocrine markers, indicating a neuroendocrine origin (31). More extensive research into the genes involved in gastrointestinal NET tumorigenesis and the cellular roles of their protein products is still under investigation.
Surgery remains the primary method of cure in limited disease (28). Multiple therapeutic options are available for metastatic disease including, surgery, ablation, and chemotherapy. However, cure is less likely and the therapeutic goal changes to extending survival, relieving symptoms, and improving quality of life. Approximately 80% of gastric NETs express somatostatin receptors, which can be targeted by octreotide and other somatostatin analogues (32). Although somatostatin analogues perform well with regards to symptom relief, their anti-neoplastic activity is thought to be minimal (28). A recent small study in patients with gastric neuroendocrine carcinoma reported promising results using the combination cisplatin and irinotecan (33).
Several receptors such as EGF, PDGF, IGF-1, and VEGF and downstream kinases like mTOR are known to be up-regulated in gastric and pancreatic NETs providing potential targets for personalized therapy (28). Clinical trials are already underway; unfortunately, most of these are in pancreatic NETs, which are known to have a different biology. Based on phase III evidence, mTOR inhibitor (Everolimus) has been approved by FDA for patients with metastatic pancreatic neuroendocrine tumors. More studies will be needed to know if the same results can be expected in gastric NETs.
Conclusions
The more we understand the different molecular pathways of tumorigenesis and progression to metastatic disease, the more accurate and effective we will become in tailoring targeted therapies. In the scope of new targeted cancer therapy approaches, molecular tests and new technologies that can analyze many genes simultaneously with high quality and cost-effectiveness are required to identify patients who will benefit from these therapies. The role of molecular pathology will only increase as clinicians and patients demand more novel diagnostic and prognostic information from the pathologist, which will ultimately allow for more personalized and effective therapy.
Acknowledgments
We acknowledge the support provided by the UC Davis Health System National Board of Advisors Vision grant awarded to M.C.
Disclosure: The authors declare no confict of interest.
References
- Rüschoff J, Hanna W, Bilous M, et al. HER2 testing in gastric cancer: a practical approach. Mod Pathol 2012;25:637-50. [PubMed]
- Kaurah P, MacMillan A, Boyd N, et al. Founder and recurrent CDH1 mutations in families with hereditary diffuse gastric cancer. JAMA 2007;297:2360-72. [PubMed]
- Khan FA, Shukla AN. Pathogenesis and treatment of gastric carcinoma: “an up-date with brief review J Cancer Res Ther 2006;2:196-9. [PubMed]
- Mbulaiteye SM, Hisada M, El-Omar EM. Helicobacter Pylori associated global gastric cancer burden. Front Biosci 2009;14:1490-504. [PubMed]
- Valle J, Gisbert JP. Helicobacter pylori infection and precancerous lesions of the stomach. Hepatogastroenterology 2001;48:1548-51. [PubMed]
- Shah MA, Khanin R, Tang L, et al. Molecular classification of gastric cancer: a new paradigm. Clin Cancer Res 2011;17:2693-701. [PubMed]
- Giaginis CT, Vgenopoulou S, Tsourouflis GS, et al. Expression and clinical significance of focal adhesion kinase in the two distinct histological types, intestinal and diffuse, of human gastric adenocarcinoma. Pathol Oncol Res 2009;15:173-81. [PubMed]
- Bang YJ, Van Cutsem E, Feyereislova A, et al. Trastuzumab in combination with chemotherapy versus chemotherapy alone for treatment of HER2-positive advanced gastric or gastro-oesophageal junction cancer (ToGA): a phase 3, open-label, randomised controlled trial. Lancet 2010;376:687-97. [PubMed]
- Rüschoff J, Dietel M, Baretton G, et al. HER2 diagnostics in gastric cancer-guideline validation and development of standardized immunohistochemical testing. Virchows Arch 2010;457:299-307. [PubMed]
- Cervera P, Fléjou JF. Changing pathology with changing drugs: tumors of the gastrointestinal tract. Pathobiology 2011;78:76-89. [PubMed]
- Laurini JA, Carter JE. Gastrointestinal stromal tumors: a review of the literature. Arch Pathol Lab Med 2010;134:134-41. [PubMed]
- Hirota S, Isozaki K, Moriyama Y, et al. Gain-of-function mutations of c-kit in human gastrointestinal stromal tumors. Science 1998;279:577-80. [PubMed]
- Miettinen M, Wang ZF, Lasota J. DOG1 antibody in the differential diagnosis of gastrointestinal stromal tumors: a study of 1840 cases. Am J Surg Pathol 2009;33:1401-8. [PubMed]
- Miettinen M, Lasota J. Gastrointestinal stromal tumors: review on morphology, molecular pathology, prognosis, and differential diagnosis. Arch Pathol Lab Med 2006;130:1466-78. [PubMed]
- Lennartsson J, Blume-Jensen P, Hermanson M, et al. Phosphorylation of Shc by Src family kinases is necessary for stem cell factor receptor/c-kit mediated activation of the Ras/MAP kinase pathway and c-fos induction. Oncogene 1999;18:5546-53. [PubMed]
- Miettinen M, Fetsch JF, Sobin LH, et al. Gastrointestinal stromal tumors in patients with neurofibromatosis 1: a clinicopathologic and molecular genetic study of 45 cases. Am J Surg Pathol 2006;30:90-6. [PubMed]
- Lasota J, Jasinski M, Sarlomo-Rikala M, et al. Mutations in exon 11 of c-kit occur preferentially in malignant versus benign gastrointestinal stromal tumors and do not occur in leiomyomas or leiomyosarcomas. Am J Pathol 1999;154:53-60. [PubMed]
- Chen H, Hirota S, Isozaki K, et al. Polyclonal nature of diffuse proliferation of interstitial cells of Cajal in patients with familial and multiple gastrointestinal stromal tumours. Gut 2002;51:793-6. [PubMed]
- Blay JY. Pharmacological management of gastrointestinal stromal tumours: an update on the role of sunitinib. Ann Oncol 2010;21:208-15. [PubMed]
- Christopoulos C, Balatsos V, Rotas E, et al. The syndrome of gastric carcinoid and hyperparathyroidism: a family study and literature review. Eur J Endocrinol 2009;160:689-94. [PubMed]
- Modlin IM, Lye KD, Kidd M. Carcinoid tumors of the stomach. Surg Oncol 2003;12:153-72. [PubMed]
- Wunderlich A, Arndt T, Fischer M, et al. Targeting the proteasome as a promising therapeutic strategy in thyroid cancer. J Surg Oncol 2012;105:357-64. [PubMed]
- Rosai J. The origin of neuroendocrine tumors and the neural crest saga. Mod Pathol 2011;24:S53-7. [PubMed]
- Fontaine J, Le Douarin NM. Analysis of endoderm formation in the avian blastoderm by the use of quail-chick chimaeras.The problem of the neurectodermal origin of the cells of the APUD series. J Embryol Exp Morphol 1977;41:209-22. [PubMed]
- Modlin IM, Kidd M, Lye KD. Biology and management of gastric carcinoid tumours: a review. Eur J Surg 2002;168:669-83. [PubMed]
- Oberg K. Genetics and molecular pathology of neuroendocrine gastrointestinal and pancreatic tumors (gastroenteropancreatic neuroendocrine tumors). Curr Opin Endocrinol Diabetes Obes 2009;16:72-8. [PubMed]
- Leotlela PD, Jauch A, Holtgreve-Grez H, et al. Genetics of neuroendocrine and carcinoid tumours. Endocr Relat Cancer 2003;10:437-50. [PubMed]
- Modlin IM, Oberg K, Chung DC, et al. Gastroenteropancreatic neuroendocrine tumours. Lancet Oncol 2008;9:61-72. [PubMed]
- Bosman FT, World Health Organization. International Agency for Research on Cancer.WHO classification of tumours of the digestive system. 4th ed. Lyon: International Agency for Research on Cancer, 2010:417p.
- Furlan D, Cerutti R, Uccella S, et al. Different molecular profiles characterize well-differentiated endocrine tumors and poorly differentiated endocrine carcinomas of the gastroenteropancreatic tract. Clin Cancer Res 2004;10:947-57. [PubMed]
- Bakkelund K, Fossmark R, Nordrum I, et al. Signet ring cells in gastric carcinomas are derived from neuroendocrine cells. J Histochem Cytochem 2006;54:615-21. [PubMed]
- Appetecchia M, Baldelli R. Somatostatin analogues in the treatment of gastroenteropancreatic neuroendocrine tumours, current aspects and new perspectives. J Exp Clin Cancer Res 2010;29:19. [PubMed]
- Okita NT, Kato K, Takahari D, et al. Neuroendocrine tumors of the stomach: chemotherapy with cisplatin plus irinotecan is effective for gastric poorly-differentiated neuroendocrine carcinoma. Gastric Cancer 2011;14:161-5. [PubMed]