Asiaticoside inhibits epithelial-mesenchymal transition and stem cell-like properties of pancreatic cancer PANC-1 cells by blocking the activation of p65 and p38MAPK
Introduction
Pancreatic cancer is a common tumor of the digestive system with a high degree of malignancy and a poor prognosis. The main clinical approach is surgery, supplemented by comprehensive treatment measures of radiotherapy and chemotherapy (1). Patients with pancreatic cancer often do not show typical symptoms in the early stage, so the detection rate is low, and they have often developed to the middle and late stages when they go to the doctor. The rate of surgical resection is low, and the rate of surgical recurrence and metastasis is high. Novel therapies such as FOLFIRINOX, a therapy combination including folinic acid, 5-fluoruracil, irinotecan and oxaliplatin, have significantly improved the treatment. However, median survival for advanced or metastatic patients was less than one and a half years even with such therapies (2). It is of great clinical significance to analyze the mechanism of the malignant progression of pancreatic cancer and find new effective drugs to assist clinical treatment. The epithelial-mesenchymal transition (EMT) is the basis of tumor cell invasion and migration, and plays an important role in the occurrence and metastasis of pancreatic cancer (3). Recent studies have shown that EMT is related to cancer stem cell-like characteristics, participates in the self-renewal and differentiation of cancer stem cell-like cells, and plays an important role in tumor occurrence, invasion, metastasis, and recurrence (4). Asiaticoside (ATS) is extracted from the Apiaceae plant Centella asiatica and has antioxidative, antifibrotic, and antineoplastic actions (5). Studies have shown that ATS can inhibit the proliferation and invasion of multiple myeloma cells, and induce tumor cell apoptosis (6), but its effect on the stem-like characteristics of pancreatic cancer cells is still unclear. Therefore, this study explored the effect of ATS on EMT and stem cell-like characteristics of pancreatic cancer cell line (PANC-1) cells, aiming to provide a reference for the clinical treatment of pancreatic cancer. We present the following article in accordance with the ARRIVE reporting checklist (available at
Methods
Instruments and reagents
The CO2 incubator and automatic microplate reader were purchased from Thermo Scientific; flow cytometer was purchased from FASCAN Becton Dickison, USA; vernier calipers (precision 0.1 mm, EC-200) were purchased from Chengdu Measuring Tool & Cutting Tool Co., Ltd.; pancreatic cancer PANC-1 cells were purchased from the cell bank of the Shanghai Chinese Academy of Sciences; ATS was purchased from Guangxi Changzhou Natural Products Development Co., Ltd., with a purity of >95%; RPMI 1640 medium and mycoplasma-free fetal bovine serum were purchased from Zhejiang Tianhang Biotechnology Co., Ltd.; CCK-8 detection kit and TUNEL tissue apoptosis detection kit were purchased from Shenyang Wanlei Biotechnology Co., Ltd.; β-actin monoclonal antibody was purchased from Beijing Soleibao Technology Co., Ltd.; CD133-PE and CD44-FITC antibodies were purchased from Miltenyi Biotec, Germany; rabbit anti-human E-cadherin, N-cadherin, vimentin, and p65, p-p65, p38 mitogen-activated protein kinase (p38MAPK), p-p38MAPK monoclonal antibodies, were purchased from Santa Cruz, USA; horseradish peroxidase (HRP) labeled goat anti-rabbit IgG was purchased from DAKO, Denmark.
We also purchased 40 male BALB/C nude mice, aged 4–6 weeks, weighing 17–22 g from Beijing Weitong Lihua Laboratory Animal Technology Co., Ltd. [animal license number SCXK (Beijing) 2016-0006]. The in vivo experiments were carried out in the Weitong Lihua Laboratory Animal Technology Co., Ltd according to Chinese National Guidelines (GB/T 35892-20181).
Cell culture and drug treatment
PANC-1 cells (about 1.5×104 in a flask) were cultured in RPMI 1640 medium containing 10% fetal bovine serum, incubated overnight at 37 °C in 5% CO2; the medium was changed the next day, and subculture was started after cell fusion reached ≥80%. Cells in the logarithmic growth phase were used to make a single-cell suspension (5×104/mL), and cultured overnight. Next, 100 µL of different concentrations of ATS (final concentration 0, 0.5, 1, 2.5, 5, 10, 25, 50, 100, 200, 300, and 400 µmol/L) was added, followed by incubation for 24 h. The culture medium was discarded, 10 µL of CCK-8 solution was added to each well, and there was a further 4 h of incubation. Finally, absorbance at 450 nm wavelength was detected, and the cell survival rate was calculated. Setting the ATS low-dose group as 10 µmol/L, the medium-dose (25 µmol/L), high-dose (50 µmol/L), and control (0 µmol/L) groups were established. For the wound enclosure test, cells of 80% confluence were scratched with a 200 µL tip, and observed at 0 and 24 h respectively. For transwell, 50,000 cells were seeded in the 6 mg/L Matrigel pretreated upper chamber of the Transwell, with DMEM containing 0.1% FBS. The lower chamber was added with normal culture medium. After 24 h the cells were stained with hematoxylin and observed under a microscope.
RT-PCR detection of proliferation markers Ki67 and PCNA mRNA levels
Each group of cells incubated with the corresponding concentration of ATS for 24 h was used to extract total RNA by the Trizol method. Next, the RNA concentration and purity were determined by electrophoresis, and the cDNA was synthesized by reverse transcription (RT), which was then used to perform RT-PCR reaction, and detect Ki67 mRNA (forward primer: 5'-CTTTGGGTGCGACTTGACGA-3', reverse primer: 5'-TTCTGCCATTACGTCCAGCG-3') and Proliferating Cell Nuclear Antigen (PCNA) (forward primer: 5'-AGGGCTGAAGATAATGCTGATA-3', reverse primer: 5'- CTCATTCATCTCTATGGTCACAG-3'). Glyceraldehyde-3-phosphate dehydrogenase (GAPDH) was used as the internal reference, and the relative expression level was calculated using 2−∆∆Ct.
Flow cytometric detection of CD133+ and CD44+ positive cells
Each group of cells incubated with the corresponding concentration of ATS for 24 h was suspended with trypsin, and the cells were collected by centrifugation (400 g for 5 min). The supernatant was discarded, the cells were resuspended in phosphate-buffered saline (PBS), and their concentration adjusted to 1×106 mL. Thereafter, CD133-PE and CD44-FITC (diluted 1:100) antibodies were added, before incubation for 30 min at 4 °C, followed by washing twice with PBS, centrifugation (1,000 rpm, 5 min), resuspension in 200 µL PBS, and analyzed by flow cytometry.
Western blot detection of phosphorylated protein expression of E-cadherin, N-cadherin, vimentin, SOX2, OCT4, p65 and p38MAPK
Each group of cells incubated with the corresponding concentration of ATS for 24 h was used to extract the total cell protein with cell lysate, and the protein concentration was determined by the quinolinic acid method. The protein was used for SDS-PAGE gel electrophoresis, and the membrane was electro-transferred to a polyvinylidene fluoride membrane, which was blocked for 2 h at room temperature, washed with membrane washing buffer, before adding the primary antibodies (E-cadherin, N-cadherin, Vimentin, SOX2, OCT4, p65, p-p65, p38MAPK, and p-p38MAPK and beta-actin), and incubating overnight at 4 °C. The membrane was washed with membrane washing buffer and the secondary antibody was added to incubate for 2 h at room temperature. Finally, electrochemiluminescence was used for imaging, with β-actin as the internal reference, and a gel imaging system was used to analyze the strength of the contrast band.
Establishment of PANC-1 nude mouse xenograft tumor model
After 7 days of acclimatization, the nude mice were randomly divided into a control group, and ATS low-dose, medium-dose, and high-dose groups. All nude mice were injected subcutaneously with 0.1 mL PANC-1 cells (1×106–1×108/mL) to establish a nude mouse xenograft tumor model. A nude mouse with visible subcutaneous tumor formation was regarded as a successful model. When the tumor volume reached ≈100 mm3, the ATS low-, medium-, and high-dose groups were intraperitoneally injected with 2.5, 5, and 10 mg/kg ATS, and the control group was injected with the same volume of normal saline. At 30 days after the injection, the nude mice were killed humanely, the tumor tissue was removed, and the volume of tumor in each group was measured.
TUNEL method to detect cell apoptosis in tumor tissue
The resected tumor tissue was placed in a 4% paraformaldehyde solution for fixation, routine sectioning, and then deparaffinizing to water. Proteinase K working solution was added for antigen retrieval, and samples were fixed at room temperature for 15–30 min. Next, 50 µL of 3% H2O2 was added, incubated at room temperature for 10 min, then 50 µL TUNEL reaction solution was added, further incubated at 37 °C in the dark for 60 mins, and finally rinsed three times with PBS for 5 min each time. Then, 50 µL peroxidase buffer solution was added, reacted at 37 °C for 30 min, rinsed three times with PBS, developed with DAB, and staining was performed in strict accordance with the instructions of the TUNEL kit.
Immunohistochemical method to detect the expression of VIMENTIN and SOX2 protein in transplanted tumor tissue
Tumor tissue sections were deparaffinized to water in turn, then treated with 1.5% H2O2–CH3OH solution at 37 °C for 30 min, blocked, and incubated at 37 °C for 30 min. Primary antibodies (vimentin and SOX2) were added respectively for 4 different sections and incubated overnight at 4 °C. Next, a biotin-labeled secondary antibody was added and incubated at 37 °C for another 30 min, color developed with DAB and controlled under the microscope so that the secondary antibody was properly bonded. Finally, sections were stained with hematoxylin and cleared with xylene after sealing with neutral gum. The number of positive cells was counted under the microscope.
Statistical analysis
SPSS 17.0 software was used to analyze the obtained data, and the measurement data that met normality are expressed as mean ± standard deviation (
Results
Effects of ATS on the survival rate of PANC-1 cells
The results of CCK-8 addition (Figure 1) showed that the inhibitory effect of ATS on PANC-1 cells was drug concentration-dependent. As the ATS concentration increased, when it reached 25 µmol/L the cell survival rate decreased significantly (P<0.05).
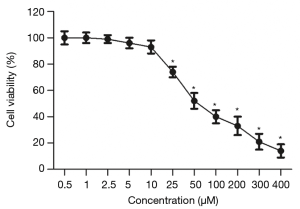
Effect of ATS on the expression of PANC-1 cell proliferation-related genes and EMT-related proteins
The RT-PCR results (Figure 2A,B) showed that with increasing ATS concentration when it was 25 µmol/L, the cells’ Ki67 and PCNA mRNA levels decreased significantly (P<0.05). Western blot results (Figure 2C,D) showed that when the ATS concentration was 25 µmol/L, the cells’ E-cadherin protein expression increased significantly (P<0.05), while that of N-cadherin and vimentin decreased significantly (P<0.05).
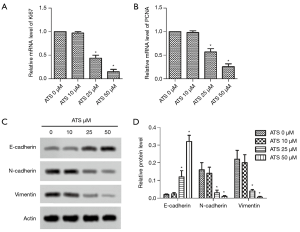
Effect of ATS on CD44+ and CD133+ positive cells, and SOX2, OCT4 protein expression
The results of flow cytometry (Figure 3A,B) showed that when the ATS concentration was 25 µmol/L, the proportions of CD44+ and CD133+ positive cells decreased significantly (P<0.05). Western blot results (Figure 3C,D) showed that when the ATS concentration was 25 µmol/L, the cells’ SOX2 and OCT4 protein expressions decreased significantly (P<0.05).
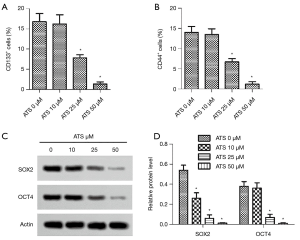
Effect of ATS on the phosphorylation of p65 and p38MAPK in PANC-1 cells
Western blot results (Figure 4A) showed that when the ATS concentration was 25 µmol/L, the cells’ p-p65/p65 and p-p38MAPK/p38MAPK protein expressions decreased significantly (Figure 4B,C, P<0.05). Then activators for nuclear factor-κB (NF-κB) and P38 MAPK were employed to show the involvement of NF-κB and P38 MAPK. Both NF-κB activator RANKL (10 ng/mL) and P38 MAPK activator Asiatic acid (4.6 mM) did not invert the decreased cell viability (Figure 4D). Then MAPK and EMT related proteins were tested (Figure 4E). RANKL and Asiatic acid activated P65 and P38 respectively (Figure 4F,G, P<0.05). E-cadherin was increased with ATS while decreased with both activators (Figure 4H, P<0.05). N-cadherin and Vimentin reduced with ATS was reverted with RANKL and Asiatic acid (Figure 4I,J, P<0.05). Similarly, ATS decreased SOX2 and OCT4 were also reverted with RANKL and Asiatic acid (Figure 4K,L, P<0.05). ATS treatment inhibited wound healing of PANC-1 cells, which was partly reverted with RANKL and Asiatic acid (Figure 4M, P<0.05). A similar trend was observed in invasive cells tested with transwell (Figure 4N, P<0.05). The results collectively indicated that ATS inhibited proliferation mobility and stemness of PANC-1 cells possibly through inactivating NF-κB and P38 MAPK.
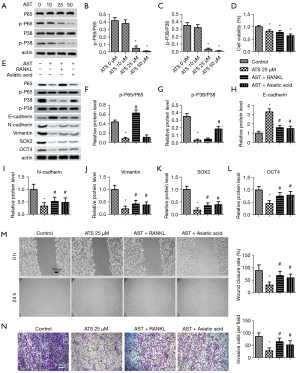
Effects of ATS on the growth, apoptosis, and expression of vimentin and SOX2 protein of transplanted tumor cells in nude mice
The results of tumor formation in nude mice (Figure 5A,B) showed that when the ATS concentration was 5 mg/kg and 10 mg/kg, the tumor’s weight and volume decreased significantly (P<0.05). The TUNEL results (Figure 5C,D) showed that when the ATS concentration was 5 mg/kg, the cell apoptosis rate of the tumor tissue increased significantly (P<0.05). The results of immunohistochemistry (Figure 5C,E,F) showed that the positive expression rates of vimentin and SOX2 proteins in tumor tissues significantly decreased (P<0.05).
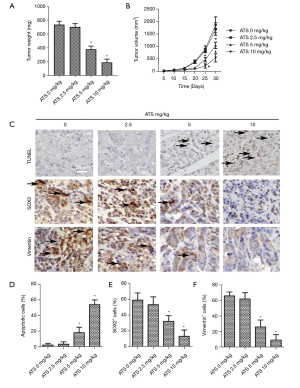
Discussion
Pancreatic cancer is a highly aggressive malignant tumor with a poor prognosis, and no clinically effective treatment. ATS is a biologically effective active ingredient extracted from Centella asiatica that can inhibit the malignant behavior of some tumor cells (7), but its effect on pancreatic cancer cells is still unclear. Therefore, this study analyzed the effects of ATS on the EMT and stem cell-like characteristics of PANC-1 pancreatic cancer cells. In this study, the inhibitory effect of ATS on PANC-1 cells was concentration-dependent, and when the ATS concentration was ≥25 µmol/L, not only the cell survival rate decreased significantly, but also the levels of Ki67 and PCNA mRNA decreased significantly. Both Ki67 and PCNA are tumor cell proliferation-related proteins, and their levels change with cell mitosis. They are ideal markers of cell proliferation (8), and our results suggested that ATS can inhibit the proliferation of PANC-1 cells by decreasing the expression of proteins related to cell proliferation in a concentration-dependent manner.
EMT program is the key to malignant transformation across tumors. The survival of metastatic patients would be dramatically reduced (2), which was enabled with EMT. EMT promotes stemness, drug resistance and immune escape of tumor cells, and make the tumors impossible to be surgically removed. Targeting of EMT to prevent malignant transforming was pivotal for pancreatic cancer patients (3). AST was reported to inhibit TGF-β-induced EMT in in the human peritoneal mesothelial cell line HMrSV5 (9). On the other hand, AST inhibits Matrix metalloproteinase, hyaluronidase and elastase (10), all of which could degrade extracellular matrix to promote EMT. In this study, when the ATS concentration reached 25 µmol/L, the cells’ E-cadherin protein expression increased significantly, while that of N-cadherin and vimentin decreased significantly. E-cadherin is a calcium-dependent epithelial marker protein that exists in almost all normal epithelial cells. It mediates adhesion between cells and plays an important role in tumor cell invasion and migration (11). N-cadherin, a calcium-dependent cell surface adhesion molecule, also mediated the adhesion and migration of cancer cells, and promotes the malignant biological behavior of tumor cells (12). Vimentin is mainly expressed in mesenchymal cells and as a component of the cytoskeleton, it can regulate cell adhesion and promote tumor cell invasion and migration (13). Bulzico et al. showed that in adrenocortical carcinomas specimens the expression of E-cadherin protein is reduced or even absent, and the expression of N-Cadherin and Vimentin protein is upregulated (14), which are signs of EMT in cancer cells, suggesting that ATS can decrease the expression of PANC-1 EMT-related proteins in a concentration-dependent manner to inhibit cell invasion and metastasis.
Cancer cells that undergo EMT could get stemness (4). Cancer stem cells were widely accepted as the main force in tumor growth, drug resistance and metastasis (15). In the adult pancreas, the differentiated pancreas cells show a high degree of plasticity, which makes it more important to inhibit cancer stem cells in pancreatic cancer treatment (16). In this study, when the ATS concentration was 25 µmol/L, the proportion of CD44+ and CD133 positive cells decreased significantly, and the expression of SOX2 and OCT4 proteins also decreased significantly. Both CD44 and CD133 are surface markers of cancer stem cells, and have been used to identify pancreatic cancer stem cells (17). Gzil et al. showed that the expression of stem cell markers such as CD44 and CD133 is a risk factor affecting the prognosis of patients with pancreatic cancer (18). Our results suggested that ATS can reduce CD44+ and CD133+ positive cells in a concentration-dependent manner, reduce tumor stem cells, and thus improve patient prognosis. SOX2 belongs to the Y-related HMG protein family in the SOX region, which can regulate embryonic development and is an important protein for maintaining stem cell self-renewal and pluripotency (19). OCT4 belongs to the POU transcription factor family and plays an extremely important role in maintaining the multidifferentiation potential and self-renewal of embryonic stem cells (20). Studies have shown that SOX2 and OCT4 are initial factors for inducing cancer stem cells. Their abnormal expression is related to the occurrence of colorectal cancer, pancreatic cancer, and other tumors (21,22). Our results suggested that ATS can inhibit the stem cell-like properties of PANC-1 cells in a concentration-dependent manner.
Inflammation promotes progression of tumors (23). It is suggested that inflammation in pre-malignant transformation and subsequent oncogene activation was the pivotal cause for pancreatic cancer. Then inflammation supported the formation of immune-tolerate microenvironment (24). AST was widely characterized as an anti-inflammatory agent. For example, Asiaticoside protects against cerebral ischemia-reperfusion injury in rats via inhibiting the NOD2/MAPK/NF-κB signaling pathway (25). Also, Asiaticoside can alleviate inflammation bronchopulmonary injury induced by hypoxia in neonatal rats (26). In this study, when the ATS concentration was 25 µmol/L, the cell’s p-p65/p65 and p-p38MAPK/p38MAPK protein expression decreased significantly. p65 belongs to the NF-κB family, and after its activation by phosphorylation it can participate in the regulation of tumor cell proliferation, invasion, migration, and other biological behaviors (27). Marquardt et al. showed that blocking the phosphorylation of NF-κB/p65 can upregulate the expression of E-cadherin, downregulate the expression of N-Cadherin and vimentin, and inhibit EMT of liver cancer cells (28). Ma et al. showed that blocking the NF-κB/p65 signaling pathway can also inhibit CD133+ positive stem cells (29), with our results suggesting that ATS may inhibit the phosphorylation of NF-κB/p65 in PANC-1 cells in a concentration-dependent manner, thus inhibiting the occurrence of EMT and the stem cell-like properties of PANC-1 cells. p38MAPK belongs to the MAPK signal pathway, and its phosphorylation can promote tumor cell proliferation, invasion, and migration, thus playing an important regulatory role (28). Studies have shown that p38MAPK can regulate the phosphorylation of NF-κB/p65 and the biological behavior of tumor cells (30), with our results suggesting that ATS may inhibit the phosphorylation of PANC-1 cells’ p38MAPK and NF-κB/p65 in a concentration-dependent manner, and also inhibit the malignant biological behavior of pancreatic cancer cells.
Inflammation, EMT and stemness were mutually affecting processes in tumors (3,15). Inflammation activates extracellular matrix degrading enzymes to admit immune screening, which promotes tumor growth, phenotypic switching and chemotherapy resistance. EMT, on the other hand, could activate inflammation and promote stemness. In this study, the results of tumor formation in nude mice showed that when the ATS concentration was 5 and 10 mg/kg, tumor volume decreased significantly, the apoptosis rate increased significantly, and the positive expression rates of vimentin and SOX2 proteins decreased significantly, suggesting that ATS can inhibit the growth of pancreatic cancer xenografts and induce cell apoptosis in a concentration-dependent manner. Our results implicated that ATS could be a multi-targeting agent in treating pancreatic carcinoma.
In summary, ATS may block the phosphorylation of p38MAPK and NF-κB/p65 in PANC-1 cells in a concentration-dependent manner, inhibiting the proliferation, EMT, and stem cell-like properties of pancreatic cancer cells and the growth of transplanted tumor cells.
Acknowledgments
Funding: None.
Footnote
Reporting Checklist: The authors have completed the ARRIVE reporting checklist. Available at http://dx.doi.org/10.21037/jgo-20-533
Data Sharing Statement: Available at http://dx.doi.org/10.21037/jgo-20-533
Conflicts of Interest: All authors have completed the ICMJE uniform disclosure form (available at http://dx.doi.org/10.21037/jgo-20-533). The authors have no conflicts of interest to declare.
Ethical Statement: The authors are accountable for all aspects of the work in ensuring that questions related to the accuracy or integrity of any part of the work are appropriately investigated and resolved. The in vivo experiments were carried out in Beijing Weitong Lihua Laboratory Animal Technology Co., Ltd. [SCXK (Beijing) 2016-0006] according to Chinese National Guidelines (GB/T 35892-20181).
Open Access Statement: This is an Open Access article distributed in accordance with the Creative Commons Attribution-NonCommercial-NoDerivs 4.0 International License (CC BY-NC-ND 4.0), which permits the non-commercial replication and distribution of the article with the strict proviso that no changes or edits are made and the original work is properly cited (including links to both the formal publication through the relevant DOI and the license). See: https://creativecommons.org/licenses/by-nc-nd/4.0/.
References
- Halbrook CJ, Lyssiotis CA. Employing Metabolism to Improve the Diagnosis and Treatment of Pancreatic Cancer. Cancer Cell 2017;31:5-19. [Crossref] [PubMed]
- Neoptolemos JP, Kleeff J, Michl P, et al. Therapeutic developments in pancreatic cancer: current and future perspectives. Nat Rev Gastroenterol Hepatol 2018;15:333-48. [Crossref] [PubMed]
- Dongre A, Weinberg RA. New insights into the mechanisms of epithelial-mesenchymal transition and implications for cancer. Nat Rev Mol Cell Biol 2019;20:69-84. [Crossref] [PubMed]
- Wang H, Unternaehrer JJ. Epithelial-mesenchymal Transition and Cancer Stem Cells: At the Crossroads of Differentiation and Dedifferentiation. Dev Dyn 2019;248:10-20. [Crossref] [PubMed]
- Zhou J, Jiang YY, Wang HP, et al. Natural compound Tan-I enhances the efficacy of Paclitaxel chemotherapy in ovarian cancer. Ann Transl Med 2020;8:752. [Crossref] [PubMed]
- Yingchun L, Huihan W, Rong Z, et al. Antitumor Activity of Asiaticoside Against Multiple Myeloma Drug-Resistant Cancer Cells Is Mediated by Autophagy Induction, Activation of Effector Caspases, and Inhibition of Cell Migration, Invasion, and STAT-3 Signaling Pathway. Med Sci Monit 2019;25:1355-61. [Crossref] [PubMed]
- Fitri AR, Pavasant P, Chamni S, et al. Asiaticoside induces osteogenic differentiation of human periodontal ligament cells through the Wnt pathway. J Periodontol 2018;89:596-605. [Crossref] [PubMed]
- Juríková M, Danihel Ľ, Polák Š, et al. Ki67, PCNA, and MCM proteins: Markers of proliferation in the diagnosis of breast cancer. Acta Histochem 2016;118:544-52. [Crossref] [PubMed]
- Zhao J, Shi J, Shan Y, et al. Asiaticoside inhibits TGF-β1-induced mesothelial-mesenchymal transition and oxidative stress via the Nrf2/HO-1 signaling pathway in the human peritoneal mesothelial cell line HMrSV5. Cell Mol Biol Lett 2020;25:33. [Crossref] [PubMed]
- Nema NK, Maity N, Sarkar BK, et al. Matrix metalloproteinase, hyaluronidase and elastase inhibitory potential of standardized extract of Centella asiatica. Pharm Biol 2013;51:1182-7. [Crossref] [PubMed]
- Coopman P, Djiane A. Adherens Junction and E-Cadherin complex regulation by epithelial polarity. Cell Mol Life Sci 2016;73:3535-53. [Crossref] [PubMed]
- Aytac Vuruskan B, Ozturk Nazlioglu H, Vuruskan H. N-cadherin expression in primary and metastatic testicular germ cell tumors. J buon 2018;23:1125-9. [PubMed]
- Richardson AM, Havel LS, Koyen AE, et al. Vimentin Is Required for Lung Adenocarcinoma Metastasis via Heterotypic Tumor Cell-Cancer-Associated Fibroblast Interactions during Collective Invasion. Clin Cancer Res 2018;24:420-32. [Crossref] [PubMed]
- Bulzico D, Pires BRB. Twist1 Correlates With Epithelial-Mesenchymal Transition Markers Fibronectin and Vimentin in Adrenocortical Tumors. Anticancer Res 2019;39:173-5. [Crossref] [PubMed]
- Razi E, Radak M, Mahjoubin-Tehran M, et al. Cancer stem cells as therapeutic targets of pancreatic cancer. Fundam Clin Pharmacol 2020;34:202-12. [Crossref] [PubMed]
- Rodriguez-Aznar E, Wiesmüller L, Sainz B Jr, et al. EMT and Stemness-Key Players in Pancreatic Cancer Stem Cells. Cancers (Basel) 2019;11:1136. [Crossref] [PubMed]
- Ishiwata T, Matsuda Y, Yoshimura H, et al. Pancreatic cancer stem cells: features and detection methods. Pathol Oncol Res 2018;24:797-805. [Crossref] [PubMed]
- Gzil A, Zarębska I, Bursiewicz W, et al. Markers of pancreatic cancer stem cells and their clinical and therapeutic implications. Mol Biol Rep 2019;46:6629-45. [Crossref] [PubMed]
- Huang C, Lu H, Li J, et al. SOX2 regulates radioresistance in cervical cancer via the hedgehog signaling pathway. Gynecol Oncol 2018;151:533-41. [Crossref] [PubMed]
- Cho Y, Kang HG, Kim SJ, et al. Post-translational modification of OCT4 in breast cancer tumorigenesis. Cell Death Differ 2018;25:1781-95. [Crossref] [PubMed]
- Wahab SMR, Islam F, Gopalan V, et al. The Identifications and Clinical Implications of Cancer Stem Cells in Colorectal Cancer. Clin Colorectal Cancer 2017;16:93-102. [Crossref] [PubMed]
- Sharma NS, Gupta VK, Dauer P, et al. O-GlcNAc modification of Sox2 regulates self-renewal in pancreatic cancer by promoting its stability. Theranostics 2019;9:3410-24. [Crossref] [PubMed]
- Greten FR, Grivennikov SI. Inflammation and Cancer: Triggers, Mechanisms, and Consequences. Immunity 2019;51:27-41. [Crossref] [PubMed]
- Stone ML, Beatty GL. Cellular determinants and therapeutic implications of inflammation in pancreatic cancer. Pharmacology & therapeutics 2019;201:202-13. [Crossref] [PubMed]
- Zhang C, Chen S, Zhang Z, et al. Asiaticoside Alleviates Cerebral Ischemia-Reperfusion Injury via NOD2/Mitogen-Activated Protein Kinase (MAPK)/Nuclear Factor kappa B (NF-κB) Signaling Pathway. Med Sci Monit 2020;26:e920325. [Crossref] [PubMed]
- Mai LJ, Fu XX, He G, et al. Effect of asiaticoside on hyperoxia-induced bronchopulmonary dysplasia in neonatal rats and related mechanism. Zhongguo Dang Dai Er Ke Za Zhi 2020;22:71-6. [PubMed]
- He F, Fan M, Jin Y, et al. Sphingosine kinase 1 inhibition decreases the epithelial-mesenchymal transition and ameliorates renal fibrosis via modulating NF-κB signaling. Am J Transl Res 2019;11:5879-87. [PubMed]
- Marquardt JU, Gomez-Quiroz L, Arreguin Camacho LO, et al. Curcumin effectively inhibits oncogenic NF-κB signaling and restrains stemness features in liver cancer. J Hepatol 2015;63:661-9. [Crossref] [PubMed]
- Ma DQ, Zhang YH, Ding DP, et al. Effect of Bmi-1-mediated NF-κB signaling pathway on the stem-like properties of CD133+ human liver cancer cells. Cancer Biomark 2018;22:575-85. [Crossref] [PubMed]
- Zhang N, Jiang Y, Mu F, et al. Gentiopicrin exerts anti-rheumatic effect in human fibroblast-like synoviocytes via inhibition of p38MAPK/NF-κB pathway. Cell Mol Biol (Noisy-le-grand) 2019;65:85-90. [Crossref] [PubMed]
(English Language Editor: K. Brown)