Knockdown of tankyrase 1 inhibits the progression of gastric adenocarcinoma via regulating human telomerase reverse transcriptase and telomeric repeat binding factor 1
Introduction
Gastric cancer is a leading cause of mortality from digestive tract cancer, which is particularly prevalent in Asian populations (1,2). The incidence of gastric cancer has increased annually worldwide. Following the development of the electronic gastroscope, the detection rate of early gastric cancer has greatly increased, but its pathogenesis is still obscure.
Telomere and telomerase are hot topics in basic tumor research in recent years. A telomere is a specific DNA-protein complex in eukaryotic linear chromosomes, which contributes to the stability of chromosome structure and functions. Telomerase is an RNA-containing reverse transcriptase composed of protein and RNA in the vast majority of tumors (3), which is crucial for the survival of cancer cells. Given that the activation of telomerase and the maintenance of telomere length are the bases of cell immortalization and unlimited proliferation (4), they have become new tumor markers and anti-cancer targets. Human telomerase reverse transcriptase (h-TERT) is the main component of human telomerase, and recurrent mutations in the promoter of h-TERT (5,6) is closely associated with telomerase activity. Telomeric repeat binding factor 1 (TRF1) is a telomeres double-stranded DNA binding protein in mammals, which has negative regulation effects on telomere length (7,8). A previous study has shown that tankyrase (TANK), a member of the poly (ADP-ribose) polymerase (PARP) superfamily, is identified to bind with the telomeric protein TRF1 and regulate telomere function (9). It is known that TANK induces ADP ribosylation of TRF1 and inhibits its binding to telomeres, suggesting that TANK is a positive regulator of telomere length and may be a target of tumor gene therapy. Recently, researchers have confirmed that TANK1 is highly expressed in neuroblastoma and bladder cancer (10,11).
Telomere, telomerase, and telomere binding proteins partaking in the regulation of DNA damage repair, cell cycle, mitosis, and cell apoptosis, are in close association with aging and tumors. It has been reported that the ablation of h-TERT induces cellular senescence and inhibits the growth of gastric cancer cells (12). Down-regulation of TRF1 and TRF2 is important for the maintenance of telomeric DNA (13). In addition, co-inhibition of TANK1 and telomerase activity exerts a synergistic effect on telomere length shortening in gastric cancer cells (14). A considerable body of evidence indicates that human telomerase antisense oligonucleotides (ASON) coupled with oligoadenylic acid has significant inhibitory effects on the growth of prostate cancer, glioma and liver cancer (15-17). These findings suggest that inhibition of telomerase activity by ASON technology can inhibit tumor cell growth, indicating that if TANK1 is inhibited by ASON, tumor cell growth may also be suppressed. Ji et al constructed antisense human TANK1 RNA retroviral vector and revealed its inhibition on tongue cancer cells (18). A previous study has reported that TANK1 was up-regulated in osteosarcoma cells and TANK1-ASODN could suppress the proliferation, migration and invasion through Hippo/YAP pathway in human osteosarcoma cells (19). It was previously identified that TANK1 is significantly upregulated in gastric cancer tissues (20); however, another study presented that TANK is not increased in gastric cancer (13). These controversial results caught our attention. Thus, the purpose of this study was to investigate the expression of TANK1 in cancer and adjacent cancer tissues in gastric adenocarcinoma patients. The effects of TANK1 ASODN on the tumor formation and cell apoptosis were analyzed both in SGC-7901 tumor-bearing mice and SGC-7901 cells to further elucidate the underlying mechanism. We present the following article in accordance with the ARRIVE reporting checklist (available at https://jgo.amegroups.com/article/view/10.21037/jgo-22-82/rc).
Methods
Patients and clinical specimens
This study was approved by the institutional ethics committee board of Suqian First Hospital (No. 20200093). All involved patients (n=15) were informed of the study and signed consent forms. The study was conducted in accordance with the Declaration of Helsinki (as revised in 2013). The anonymous medical records of the patients were reviewed, and the exclusion criteria were as follows: non-adenocarcinomas, radiotherapy or chemotherapy prior to surgery, and incomplete clinical data. Paired gastric cancer and adjacent non-tumor tissues were collected from 15 patients diagnosed with gastric adenocarcinoma at Suqian First Hospital between January 2021 and June 2021, and then preserved in liquid nitrogen.
Cell lines
We cultured SGC-7901 cells (Procell, Wuhan, China) in Roswell Park Memorial Institute (RPMI) 1640 (Procell) containing 10% fetal bovine serum (FBS; Procell) in a humidified incubator with 5% CO2 at 37 ℃.
Tumor-bearing model
A total of 15 male BALC/C nude mice (5–6 weeks old; weighing 20±2 g, ~18–22 g) were randomly divided into 3 groups (n=5 in each group): vehicle group, TANK1 sense oligonucleotides (TANK1 SODN) group, and TANK1 ASODN group. Random numbers were generated using the standard = RAND () function in Microsoft Excel (Microsoft Corp., Redmond, WA, USA). All animals were housed under a standardized condition with a light-dark cycle at 22±2 ℃ and 55%±5% humidity. Animal experiments were performed under a project license (No. IACUC-20200922-01) granted by institutional ethics committee board of Zhaofenghua Biotechnology (Nanjing) Co., Ltd., in compliance with the National Institutes of Health guidelines for the care and use of animals. And the animal experiments were done in the Zhaofenghua Biotechnology (Nanjing) Co., Ltd. A protocol was prepared before the study without registration.
TANK1 SODN and TANK1 ASODN were synthesized by General Biol (General Biosystems, Anhui, China) according to the initial codon of the protein translation site of TANK1 complementary DNA (cDNA) sequence. The SGC-7901 cells in logarithmic growth phase were collected and re-suspended in normal saline. We then injected 100 µL suspension (1 million cells) subcutaneously at the right inguinal site of each nude mouse. Liposomes-encased TANK1 SODN and TANK1 ASODN were diluted with normal saline to a final concentration of 0.5 µg/µL. Each mouse was injected with 50 µL mixture for 3 times every 5 days until a tumor diameter of 0.5 cm was achieved. The vehicle group, injected with equivalent normal saline containing liposomes, was used as the negative control group. Tumor volumes were recorded every 3 days. At 24 days later, mice were sacrificed, and tumor weight was measured. Tumor volume was calculated based on the formula: tumor volume (mm3) = (width) × (height)2/2. All animals were sacrificed with an intraperitoneal injection of 200 mg/kg sodium pentobarbital (body weight). After death verification by cessation of the heartbeat, the tumor tissues were obtained for further investigation.
TUNEL staining
Formaldehyde was utilized to fix tumor tissues, and part of the tissues were embedded in paraffin. Tissue slices were prepared and stained with terminal deoxynucleotidyl transferase dUTP nick end labeling (TUNEL) mixture (Roche, Shanghai, China) following deparaffinization, hydration, and permeation. Subsequently, slices were washed with phosphate-buffered saline (PBS), mixed with 3,3’-diaminobenzidine (DAB) solution, redyed with hematoxylin, and washed with flowing water. After dehydration and vitrification, the apoptotic cells were observed under microscope (Leica, Heidelberg, Germany; magnification, ×100).
Immunohistochemistry
Briefly, tissue slices were subjected to incubation with primary antibodies against Ki67 and h-TERT (Abcam, Cambridge, UK) at 4 ℃ following deparaffinization, antigen retrieval, and blockade. Then, slices were incubated with corresponding secondary antibody (Abcam). Pictures were taken under a microscope (Leica; magnification, ×100).
Real-time quantitative polymerase chain reaction
We used TRIzol (Invitrogen, Carlsbad, CA, USA) to extract the total RNA, followed by reverse transcription using tRevertAid™ H Minus First Strand cDNA Synthesis Kit (Fermantas, St. Leon-Rot, Germany). The polymerase chain reaction (PCR) was carried out using a PCR 7500 System and Power SYBR Green PCR master mix (both Applied Biosystems; Thermo Fisher Scientific Inc., Waltham, MA, USA) according to the manufacturer’s instructions. The following thermocycling conditions were used: 30 s at 95 ℃ for 1 cycle; 3 s at 95 ℃, 30 s at 60 ℃ and 30 s at 72 ℃ for 40 cycles; and 5 min at 72 ℃ for 1 cycle. Glyceraldehyde 3-phosphate dehydrogenase (GAPDH) was used as the internal reference gene. Relative gene expression levels were calculated using the 2−ΔΔCq method (21).
Cell proliferation assay
The SGC-7901 cells in logarithmic growth phase were seeded in a 96-well culture plate. Cell proliferation ability was assessed by cell counting kit-8 (CCK-8; KeyGen, Nanjing, China) at 24, 48, and 72 h. Then, 10 µL CCK-8 solution was added to the medium and incubated for an additional 3 h. The absorbance at 450 nm was measured using a microplate reader (BioRad Laboratories, Hercules, CA, USA).
Flow cytometry analysis
Cells in each group were collected by centrifugation, washed twice with pre-cooled PBS, and then re-suspended in 500 µL buffer solution. We mixed 100 µL buffer solution with 5 µL Annexin V-APC (KeyGen), and subsequently, 5 µL propidium iodide (PI; KeyGen) was added for incubation of 15 min at room temperature (avoiding light). Next, 400 µL PBS was added to re-suspend cells and filtered with a 400-mesh sieve. Flow cytometry (Becton Dickinson and Co., Franklin Lakes, NJ, USA) was conducted to analyze apoptotic cells.
Statistical analysis
Data was represented as mean ± standard deviation and analyzed by Student’s t-test or one-way analysis of variance (ANOVA) followed by Tukey’s post hoc test with GraphPad Prism 8.0 (GraphPad Software, Inc., La Jolla, CA, USA). Each experiment was performed at least 3 times. Statistical significance was considered when P<0.05.
Results
The expression levels of TANK1, h-TERT, and TRF1 in gastric adenocarcinoma
In order to clarify the expression levels of TANK1, h-TERT, and TRF1, 15 pairs of gastric adenocarcinoma and adjacent non-tumor tissues were prepared for reverse transcription quantitative polymerase chain reaction (RT-qPCR) analysis. The results showed that the expression of TANK1 was significantly increased in tumor tissues compared with non-tumor tissues (Figure 1A). Likewise, h-TERT was obviously elevated in tumor tissues in comparison to the adjacent non-tumor tissues (Figure 1B). However, it was observed that the mRNA level of TRF1 was decreased in tumor tissues compared with non-tumor tissues (Figure 1C). These results indicated that the high levels TANK1 and h-TERT, along with low expression of TRF1, were associated with the development of gastric adenocarcinoma.
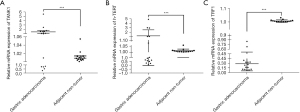
TANK1 ASODN delays tumor formation and promotes cell apoptosis in SGC-7901 tumor-bearing mice
Subsequently, tumor-bearing mice were established to validate the role of TANK1 in vivo. We injected TANK1 SODN and TANK1 ASODN into SGC-7901 tumor-bearing mice to observe the function of TANK1 in vivo. Following 24 days-treatment of TANK1 SODN or TANK1 ASODN, tumor tissues from nude mice were harvested and photographed. It was obvious that TANK1 ASODN markedly lessened the tumor diameter compared to vehicle group; whereas, there were no significant distinctions between TANK1 SODN group and vehicle group (Figure 2A). In addition, TUNEL staining was employed to investigate the cell apoptosis in tumor tissues. The results exhibited that TANK1 ASODN prominently promoted cell apoptosis when compared with the vehicle group. Contrastingly, limited inhibition of cell apoptosis was presented in the TANK1 SODN group (Figure 2B). In brief, compared with TANK1 SODN, TANK1 ASODN appeared to be more effective in reducing tumor formation and enhancing cell apoptosis.
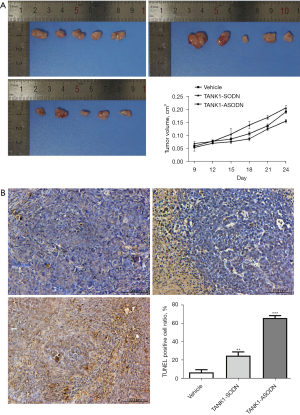
TANK1 ASODN decreases the expression levels of Ki67 and h-TERT in SGC 7901 cells tumor-bearing mice
The marker Ki67 serves as a proliferation marker, the function of which is strongly related to mitosis, and it is indispensable to tumor cell proliferation and growth (22). The expression of Ki67 is widely used as a predictive indicator for the assessment of tumor outcomes (23,24). Immunohistochemistry (IHC) assay suggested that the Ki67 positive area in the TANK1 ASODN group was dramatically reduced compared to the vehicle group and TANK1 SODN groups (Figure 3A). Of note, TANK1 SODN also decreased the expression of Ki67 (Figure 3A). Moreover, the level of h-TERT was estimated, which indicated that TANK1 ASODN remarkably restrained the expression of h-TERT relative to the vehicle group and TANK1 SODN group (Figure 3B). These results illustrated that TANK1 ASODN could limit tumor growth and decrease the expression of h-TERT.
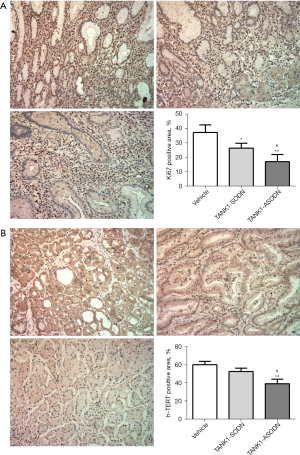
Silencing TANK1 inhibits the proliferation and promotes the apoptosis of SGC-7901 cells
To investigate the function of TANK1 in gastric adenocarcinoma in vitro, TANK1 ASODN was transfected into SGC-7901 cells. As shown in Figure 4A, TANK1 ASODN inhibited the expression of TANK1 as compared to the vehicle group. The CCK-8 assay suggested that TANK1 ASODN remarkably inhibited proliferation of SGC-7901 cells compared with the vehicle group (Figure 4B). To further investigate the effect of TANK1 ASODN on the apoptosis of SGC-7901 cells, flow cytometry analysis was conducted. The results revealed that the apoptotic rate of cells transfected with TANK1 ASODN was apparently higher than control group or vehicle group (Figure 4C,4D). Collectively, TANK1 ASODN exhibited a powerfully suppressive effect on cell proliferation and a significantly promotive effect on cell apoptosis.
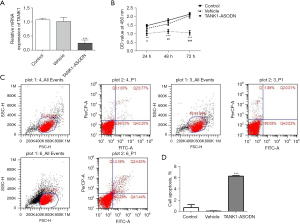
Knockdown of TANK1 affects the expression levels of h-TERT and TRF1 in SGC-7901 cells
After the above exploration, the possible mechanism was further probed. The TANK1 gene could weaken the binding of TRF1 to telomeres, which provides a premise for the activation of telomerase and its binding with telomere (9). The TRF1 gene is an inhibitor of telomere elongation and related to the negative feedback mechanism of stabilizing telomere length. When compared to the vehicle group, the expression of h-TERT was decreased in the TANK1 ASODN group (Figure 5A). Moreover, it was noted that TANK1 ASODN simultaneously elevated the mRNA level of TRF1 compared with the vehicle group (Figure 5B). Taken together, these results indicated that TANK1 ASODN could downregulate but upregulate the mRNA levels of h-TERT and TRF1, respectively.
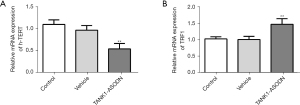
Discussion
Telomere, telomerase, and telomere binding proteins play pivotal roles in cellular immortality and tumorigenesis. The gene TANK1 is a regulator of telomerase activation and telomere prolongation (25). The level of TANK negatively correlates with poor survival of lung cancer patients (26). Recent studies have demonstrated that inhibition of TANK significantly reduces prostate cancer cell proliferation and inhibits the growth of human osteosarcoma xenograft (27,28). Besides, TANK promotes proliferation of ovarian cancer through activation of Wnt/β-catenin signaling (29). However, the expression of TANK1 in gastric cancer is still controversial. A previous study suggested that TANK1 is significantly upregulated in gastric cancer tissues (20), while contrary findings were revealed by Yamada et al. (13). The most common type of gastric cancer is adenocarcinoma. In the present study, 15 matched gastric adenocarcinoma and adjacent non-tumor tissues were collected according to the exclusion criteria. After detection, we disclosed that the mRNA levels of TANK1 and h-TERT were elevated while TRF1 was downregulated in gastric adenocarcinoma tissues, together indicating that the overexpression of TANK1 may be strongly correlated with gastric carcinogenesis.
To further evaluate the specific functions of TANK1 on gastric adenocarcinoma, a tumor-bearing model was established by injection with TANK1 ASODN. It was observed that TANK1 ASODN inhibited the progression of gastric adenocarcinoma and affected the expression of h-TERT. Furthermore, SGC-7901 cells were cultured in vivo and transfected with TANK1 ASODN. The cell proliferation was markedly inhibited, but the cell apoptosis was significantly increased by TANK1 ASODN. It has been reported that TANK knockdown using small interfering RNA (siRNA) could suppress the proliferation of the hepatocellular cancer (HCC) cell lines (30). The proliferation of human tumor cells is antagonized by TANK inhibitors via stabilization of angiomotin (31). In addition, TANK inhibition impairs directional migration and invasion of lung cancer cells (32). These findings highlight an anti-tumor effect of TANK inhibitors, consistent with the results presented in the current study.
In conclusion, the present study illustrated that TANK1 was increased in gastric adenocarcinoma, and TANK1 ASODN developed an anti-tumor role possibly through manipulating the expressions of h-TERT and TRF1. However, telomerase activity and telomere length were not included in this study, and to improve the research, further investigation is indispensable.
Acknowledgments
Funding: This study was supported by 2016 Jiangsu Provincial Key Medical Talents Funding Project for Youth (No. QNRC2016486).
Footnote
Reporting Checklist: The authors have completed the ARRIVE reporting checklist. Available at https://jgo.amegroups.com/article/view/10.21037/jgo-22-82/rc
Data Sharing Statement: Available at https://jgo.amegroups.com/article/view/10.21037/jgo-22-82/dss
Conflicts of Interest: All authors have completed the ICMJE uniform disclosure form (available at https://jgo.amegroups.com/article/view/10.21037/jgo-22-82/coif). The authors have no conflicts of interest to declare.
Ethical Statement: The authors are accountable for all aspects of the work in ensuring that questions related to the accuracy or integrity of any part of the work are appropriately investigated and resolved. This study was approved by the institutional ethics committee board of Suqian First Hospital (No. 20200093). All involved patients (n=15) were informed of the study and signed consent forms. The study was conducted in accordance with the Declaration of Helsinki (as revised in 2013). Animal experiments were performed under a project license (No. IACUC-20200922-01) granted by institutional ethics committee board of Zhaofenghua Biotechnology (Nanjing) Co., Ltd., in compliance with the National Institutes of Health guidelines for the care and use of animals.
Open Access Statement: This is an Open Access article distributed in accordance with the Creative Commons Attribution-NonCommercial-NoDerivs 4.0 International License (CC BY-NC-ND 4.0), which permits the non-commercial replication and distribution of the article with the strict proviso that no changes or edits are made and the original work is properly cited (including links to both the formal publication through the relevant DOI and the license). See: https://creativecommons.org/licenses/by-nc-nd/4.0/.
References
- Katoh H, Ishikawa S. Lifestyles, genetics, and future perspectives on gastric cancer in east Asian populations. J Hum Genet 2021;66:887-99. [Crossref] [PubMed]
- Sekiguchi M, Oda I, Matsuda T, et al. Epidemiological Trends and Future Perspectives of Gastric Cancer in Eastern Asia. Digestion 2022;103:22-8. [Crossref] [PubMed]
- Blackburn EH, Collins K. Telomerase: an RNP enzyme synthesizes DNA. Cold Spring Harb Perspect Biol 2011;3:a003558. [Crossref] [PubMed]
- Jafri MA, Ansari SA, Alqahtani MH, et al. Roles of telomeres and telomerase in cancer, and advances in telomerase-targeted therapies. Genome Med 2016;8:69. [Crossref] [PubMed]
- Horn S, Figl A, Rachakonda PS, et al. TERT promoter mutations in familial and sporadic melanoma. Science 2013;339:959-61. [Crossref] [PubMed]
- Huang FW, Hodis E, Xu MJ, et al. Highly recurrent TERT promoter mutations in human melanoma. Science 2013;339:957-9. [Crossref] [PubMed]
- d'Alcontres MS, Palacios JA, Mejias D, et al. TopoIIα prevents telomere fragility and formation of ultra thin DNA bridges during mitosis through TRF1-dependent binding to telomeres. Cell Cycle 2014;13:1463-81. [Crossref] [PubMed]
- van Steensel B, de Lange T. Control of telomere length by the human telomeric protein TRF1. Nature 1997;385:740-3. [Crossref] [PubMed]
- Yang L, Sun L, Teng Y, et al. Tankyrase1-mediated poly(ADP-ribosyl)ation of TRF1 maintains cell survival after telomeric DNA damage. Nucleic Acids Res 2017;45:3906-21. [Crossref] [PubMed]
- Tian XH, Hou WJ, Fang Y, et al. XAV939, a tankyrase 1 inhibitior, promotes cell apoptosis in neuroblastoma cell lines by inhibiting Wnt/β-catenin signaling pathway. J Exp Clin Cancer Res 2013;32:100. [Crossref] [PubMed]
- Gelmini S, Quattrone S, Malentacchi F, et al. Tankyrase-1 mRNA expression in bladder cancer and paired urine sediment: preliminary experience. Clin Chem Lab Med 2007;45:862-6. [Crossref] [PubMed]
- La SH, Kim SJ, Kang HG, et al. Ablation of human telomerase reverse transcriptase (hTERT) induces cellular senescence in gastric cancer through a galectin-3 dependent mechanism. Oncotarget 2016;7:57117-30. [Crossref] [PubMed]
- Yamada M, Tsuji N, Nakamura M, et al. Down-regulation of TRF1, TRF2 and TIN2 genes is important to maintain telomeric DNA for gastric cancers. Anticancer Res 2002;22:3303-7. [PubMed]
- Zhang H, Yang MH, Zhao JJ, et al. Inhibition of tankyrase 1 in human gastric cancer cells enhances telomere shortening by telomerase inhibitors. Oncol Rep 2010;24:1059-65. [PubMed]
- Li Y, Malaeb BS, Li ZZ, et al. Telomerase enzyme inhibition (TEI) and cytolytic therapy in the management of androgen independent osseous metastatic prostate cancer. Prostate 2010;70:616-29. [Crossref] [PubMed]
- Iwado E, Daido S, Kondo Y, et al. Combined effect of 2-5A-linked antisense against telomerase RNA and conventional therapies on human malignant glioma cells in vitro and in vivo. Int J Oncol 2007;31:1087-95. [PubMed]
- Yang B, Yu RL, Tuo S, et al. Antisense oligonucleotide against hTERT (Cantide) inhibits tumor growth in an orthotopic primary hepatic lymphoma mouse model. PLoS One 2012;7:e41467. [Crossref] [PubMed]
- Ji YX, Zhang P, Chen WM, et al. Construction of antisense human tankyrase-1 RNA retroviral vector and its inhibition on tongue cancer cells. Zhonghua Kou Qiang Yi Xue Za Zhi 2007;42:180-3. [PubMed]
- Zhou Y, Jin Q, Xiao W, et al. Tankyrase1 antisense oligodeoxynucleotides suppress the proliferation, migration and invasion through Hippo/YAP pathway in human osteosarcoma cells. Pathol Res Pract 2019;215:152381. [Crossref] [PubMed]
- Gao J, Zhang J, Long Y, et al. Expression of tankyrase 1 in gastric cancer and its correlation with telomerase activity. Pathol Oncol Res 2011;17:685-90. [Crossref] [PubMed]
- Livak KJ, Schmittgen TD. Analysis of relative gene expression data using real-time quantitative PCR and the 2(-Delta Delta C(T)) Method. Methods 2001;25:402-8. [Crossref] [PubMed]
- Li LT, Jiang G, Chen Q, et al. Ki67 is a promising molecular target in the diagnosis of cancer Mol Med Rep 2015;11:1566-72. (review). [Crossref] [PubMed]
- Jacobsen F, Kohsar J, Gebauer F, et al. Loss of p16 and high Ki67 labeling index is associated with poor outcome in esophageal carcinoma. Oncotarget 2020;11:1007-16. [Crossref] [PubMed]
- Muzashvili T, Tutisani A, Chabradze G, et al. The study of the expression of CDH1, KI67, P53 and HER2 in diffuse gastric carcinoma. Georgian Med News 2020;147-50. [PubMed]
- Muramatsu Y, Ohishi T, Sakamoto M, et al. Cross-species difference in telomeric function of tankyrase 1. Cancer Sci 2007;98:850-7. [Crossref] [PubMed]
- Li N, Wang Y, Neri S, et al. Tankyrase disrupts metabolic homeostasis and promotes tumorigenesis by inhibiting LKB1-AMPK signalling. Nat Commun 2019;10:4363. [Crossref] [PubMed]
- Cheng H, Li X, Wang C, et al. Inhibition of tankyrase by a novel small molecule significantly attenuates prostate cancer cell proliferation. Cancer Lett 2019;443:80-90. [Crossref] [PubMed]
- Martins-Neves SR, Paiva-Oliveira DI, Fontes-Ribeiro C, et al. IWR-1, a tankyrase inhibitor, attenuates Wnt/β-catenin signaling in cancer stem-like cells and inhibits in vivo the growth of a subcutaneous human osteosarcoma xenograft. Cancer Lett 2018;414:1-15. [Crossref] [PubMed]
- Yang HY, Shen JX, Wang Y, et al. Tankyrase Promotes Aerobic Glycolysis and Proliferation of Ovarian Cancer through Activation of Wnt/β-Catenin Signaling. Biomed Res Int 2019;2019:2686340. [Crossref] [PubMed]
- Huang J, Qu Q, Guo Y, et al. Tankyrases/β-catenin Signaling Pathway as an Anti-proliferation and Anti-metastatic Target in Hepatocarcinoma Cell Lines. J Cancer 2020;11:432-40. [Crossref] [PubMed]
- Troilo A, Benson EK, Esposito D, et al. Angiomotin stabilization by tankyrase inhibitors antagonizes constitutive TEAD-dependent transcription and proliferation of human tumor cells with Hippo pathway core component mutations. Oncotarget 2016;7:28765-82. [Crossref] [PubMed]
- Lupo B, Vialard J, Sassi F, et al. Tankyrase inhibition impairs directional migration and invasion of lung cancer cells by affecting microtubule dynamics and polarity signals. BMC Biol 2016;14:5. [Crossref] [PubMed]
(English Language Editor: J. Jones)