Regulation of survivin and caspase/Bcl-2/Cyto-C signaling by TDB-6 induces apoptosis of colorectal carcinoma LoVo cells
Introduction
Colorectal carcinoma (CRC) is one of the most common tumors of the digestive system, and its morbidity and mortality rank third and fourth among all malignancies, respectively (1,2). CRC mostly occurs in the rectum and the junction of the rectum and sigmoid colon. The etiology and pathogenesis of CRC are complex. CRC is caused by a variety of factors such as genetic and environmental factors and functional molecular regulation abnormalities (3,4). A combination of surgery and radiotherapy, chemotherapy, immunotherapy, or targeted therapy is the main treatment strategy for CRC. Medication is critical for advanced CRC patients since they cannot be treated surgically because of metastasis (5-7). Erbitux, which targets epidermal growth factor receptor (EGFR), and the multiple tyrosine kinase inhibitor regorafenib have been proven to improve the condition and prolong the survival of CRC patients. However, the 5-year survival rate is still low and the treatment for advanced CRC remains severe (8-10). It is of great significance to further study the pathological features of CRC and develop new anti-CRC drugs.
Apoptosis reduction is a pivotal feature of tumors and induction of apoptosis has gradually become a promising anti-tumor treatment (11,12). Abnormal regulation of mitochondrial-mediated endogenous apoptotic pathways plays an important role in tumorigenesis. The induction of mitochondrial-mediated apoptosis will not only lead to the rapid decrease of mitochondrial membrane potential and electron transport decoupling, but also cause the increase of mitochondrial membrane permeability, which further induces the release of cytochrome-C (Cyto-C) (13-15). In the cytoplasm, Cyto-C can bind to non-activated caspase-9 to produce apoptotic bodies and activate caspase-9, thus promoting the apoptosis of tumor cells (16). Inhibitor of apoptosis protein (IAP) is a natural caspase inhibitor, and survivin is a member of the IAP family, which directly or indirectly suppresses the activity of caspase-3 and caspase-7. Survivin can also interfere with the activity of caspase-9 and block mitochondria-mediated apoptosis (17,18). Previous studies indicated that survivin was widely expressed in gastric, liver, lung, breast, and prostate cancers, but it was not expressed or showed low expression in adjacent tissues, making survivin a potential tumor marker (19). Specific inhibition of survivin could induce apoptosis and suppress the proliferation of hepatocellular carcinoma (HCC), and similar effects were observed in CRC, leukemia, and breast cancer, among others. Therefore, survivin might be a promising target for tumor inhibition (20,21).
Natural products are a kind of valuable compound library, containing rich medicinal resources. Many natural products and their chemical derivatives have been found to be effective in tumor suppression (22). Taspine, isolated from the medicinal plant Radix Aconitum, is a natural product with extensive pharmacological activities, including the inhibition of bacteria, angiogenesis, and malignant tumors (23,24). TDB-6 is a new taspine derivative synthesized in our laboratory, the lipid solubility and bioavailability of which were significantly improved compared to that of taspine. In this study, the anti-CRC effect of TDB-6 (Figure 1A) was investigated in vitro and in vivo. We mainly studied the inhibitory effect of TDB-6 on the proliferation and apoptosis of CRC cells, and preliminarily investigated the underlying mechanism. We present the following article in accordance with the ARRIVE reporting checklist (available at https://jgo.amegroups.com/article/view/10.21037/jgo-22-780/rc).
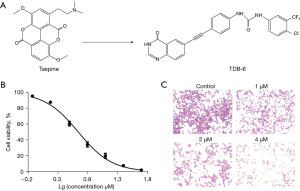
Methods
Chemicals and reagents
TDB-6 was obtained from the Research and Engineering Center for Natural Medicine, Xi’an Jiaotong University (Xi’an, China). Dulbecco’s modified Eagle’s medium (DMEM) was purchased from HyClone (Logan, UT, USA). Phosphate-buffered saline (PBS) and fetal bovine serum (FBS) were obtained from ExCell Bio (Shanghai, China). Trypsin and antibiotics were obtained from Beijing Solarbio Science & Technology Co., Ltd. (Beijing, China). Dimethyl sulfoxide (DMSO), Rhodamine 123, and 3-(4,5-dimethylthiazol-2-yl)-2.5-diphenyl-2H-tetrazolium bromide (MTT) powder were obtained from Sigma-Aldrich (St. Louis, MO, USA). The Annexin V FITC/PI cell apoptosis reagent kit was purchased from Beyotime Institute of Biotechnology (Shanghai, China). The BCA protein assay reagent kit was obtained from Pierce Biotechnology (Waltham, MA, USA). RIPA lysis buffer was purchased from Pioneer Biotechnology Co., Ltd. (Shaanxi, China). Protease and phosphatase inhibitors were obtained from Roche Tech. (Basel, Switzerland). Tween-20 was obtained from Amresco (Pennsylvania, USA). Sodium carboxylmethyl cellulose (CMC-Na) was purchased from Jinyi Biology Co., Ltd. (Henan, China). Bcl-2, survivin, Bid, cleaved caspase-3, cleaved caspase-7, cleaved caspase-9, cleaved caspase-PARP, and Cyto-C rabbit mAb were from Protein Technology Group (Chicago, Illinois, USA). HRP-conjugated goat anti-rabbit IgG and β-actin rabbit mAb were obtained from ABclonal (Boston, MA, USA).
Cell line and cell culture
The human CRC cell line LoVo was obtained from the Shanghai Institute of Cell Biology at the Chinese Academy of Sciences. The cells were cultured in DMEM supplemented with 10% FBS and 5% antibiotics. The cells were incubated in 95% air and 5% CO2 in a humidified atmosphere at 37 ℃.
Animals and xenograft model
Male BALB/c nude mice (4 to 6 weeks old, 18–22 g) were housed at the Laboratory Animal Center of Xi’an Jiaotong University with a 12-h light-dark cycle. The animals were permitted free consumption of a standard chow diet and water. All the animal studies were conducted according to regional authority guidelines. To establish the xenograft model of colon cancer, 12 mice received 200 µL LoVo cell suspension (2×107 cells/mL) per mouse by subcutaneous inoculation. When the LoVo cells developed as a palpable tumor, the tumor volume was measured once every 2 days. The mice were randomly divided into 3 groups (4 mice per group) when the tumor volume reached 100 mm3. The grouped mice received TDB-6 (40 or 80 mg/kg) or 0.5% CMC-Na solution every day by intragastric administration. The dose of TDB-6 (40 or 80 mg/kg) was determined according to the pre-experiment result, including the acute toxicity study and pharmacodynamic investigate the body weight of each mouse was recorded daily. After 2 weeks, the mice were sacrificed by the cervical dislocation method. The tumors and spleens were removed and weighed. Animal experiments were performed under a project license (No. 2022-1434) granted by the Research Ethics Committee of Xi’an Jiaotong University, in compliance with the Chinese Academy of Sciences Animal Care and Use Committee guidelines for the care and use of animals. A protocol was prepared before the study without registration.
Cell viability assay
LoVo cells were trypsinized and re-plated into a 96-well plate with a total cell suspension volume of 180 µL per well. Following incubation for 24 h, an increasing gradient of TDB-6 (0.39–50 µM) was added into the plate, with a total volume of 20 µL per well. After 48 h, the medium with TDB-6 was replaced by MTT solution dissolved in serum free medium. Following incubation for 4–6 h, the supernatant was removed and 150 µL DMSO was added per well. The plate was subsequently shaken 15 min for thorough dissolution and measured at 490 nm.
Cell apoptosis assay
The cells were seeded in a 6-well plate at proper density for 24 h. For the TDB-6 treatment experiment, cells were subsequently exposed to TDB-6 (0, 1, 2, 4 µM). For YM-155 and TDB-6 treatment experiments, the cells were exposed to YM-155 (100 nM), TDB-6 (4 µM), or both. At 48 h later, the treated cells were trypsinized and resuspended in binding buffer, followed by incubation with annexin V-FITC for 15 min and Propidium lodide (PI) for 5 min in the dark. Finally, the stained cells were detected by a NovoCyte 2040R flow cytometer (ACEA, San Diego, CA, USA).
Hoechst staining
LoVo cells were cultured in a 96-well plate at proper density for 24 h. For the TDB-6 treatment experiment, cells were subsequently exposed to TDB-6 (0, 1, 2, 4 µM). For YM-155 and TDB-6 treatment experiments, the cells were exposed to YM-155 (100 nM), TDB-6 (4 µM), or both. After 48 h incubation, the treated cells were washed 3 times with PBS and subsequently fixed with 4% paraformaldehyde for 15 min. Following washing again with PBS, the cells were incubated with hoechst 33258 for 15 min. Images were obtained using an inverted fluorescence microscope.
Mitochondrial membrane potential (Δψm) assay
LoVo cells were seeded in a 6-well plate at proper density for 24 h. After treatment with TDB-6 (0, 1, 2, 4 µM) for 48 h, the cells were rinsed with DMEM, followed by incubation with Rhodamine 123 (1 mM) for 30 min at 37 ℃ in the dark. The fluorescently labeled cells were rinsed again and analyzed using a NovoCyte 2040R flow cytometer (ACEA, San Diego, CA, USA).
Western blot assay
Treated cells or tumor tissues were placed at 4 ℃ in RIPA buffer supplemented with a protease and phosphatase inhibitor cocktail. After centrifuging at 12,000 r/min for 10 min, the precipitate was discarded and the supernatant was collected for western blotting. A BCA Protein Quantification kit was utilized to determine the protein concentrations of cells or tissues samples. In order to separate the proteins, equivalent amounts of samples were electrophoresed on SDS-polyacrylamide gels. Then the proteins were transferred to polyvinylidene difluoride membranes, following by blocking with 5% non-fat milk for 2 h and incubating with primary antibodies overnight. After washing 4 times with TBST for 5 min each, the membranes were incubated with secondary antibodies (HRP-conjugated) at 37 ℃ for 1 h, after which they were washed 4 times with TBST for 5 min each. After detection with an ECL kit, protein bands were visualized by a Tanon 5200 automatic chemiluminescence image analysis system (Tanon, Shanghai, China).
Statistical analysis
All data are expressed as the mean ± SD. Statistical analysis was performed by SPSS (version 16.0; IBM, Armonk, NY, USA). Statistical differences between different treated groups was analyzed by ANOVA. A Student’s t-test was used to compare one group with the control group. Significance was set at P<0.05.
Results
TDB-6 suppressed the proliferation of LoVo cells
The effect of TDB-6 on the viability of LoVo cells was investigated by the MTT assay and the results are shown in Figure 1B. The viability of LoVo cells was significantly inhibited by TDB-6 in a dose-dependent manner, with an IC50 value of 4.49±0.33 µM. The crystal violet staining assay was conducted on LoVo cells treated with different concentrations of TDB-6 (1, 2, and 4 µM). The results in Figure 1C demonstrated that the proliferation of LoVo cells was obviously suppressed by TDB-6.
TDB-6 inhibited the tumor growth of the xenograft model in nude mice
A xenograft model was utilized to investigate the anti-tumor effect of TDB-6 on LoVo cells in vivo. The results indicated that TDB-6 significantly delayed tumor growth at the indicated concentrations (Figure 2A-2C). The inhibitory rate of TDB-6 on tumor growth was more than 50% (56.91%) at the concentration of 80 mg/kg (Figure 2D). Spleen index (spleen weight/body weight) is closely associated with immunity, and can reflect side effects of drugs. As shown in Figure 2E,2F, the spleen weight and body weight showed no significant difference between the TDB-6 treated groups and control group, indicating no visible damage was caused by TDB-6 on BALB/c nude mice.
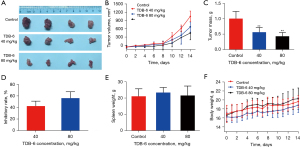
TDB-6 induced the apoptosis of LoVo cells by regulating survivin
The effect of TDB-6 on LoVo cell apoptosis was investigated by flow cytometry. The results of Annexin-V FITC/PI analysis in Figure 3A showed that TDB-6 increased the percent of apoptotic cells in a dose-dependent manner compared to the control group. Hoechst staining was also conducted on LoVo cells and the result is shown in Figure 3B. TDB-6 could induce condensed bright blue apoptotic nuclei in LoVo cells, demonstrating its apoptosis induction effect. The result of the Δψm assay indicated that the fluorescence intensity of Rhodamine 123 in LoVo cells decreased significantly after TDB-6 treatment, which indicated the decrease of mitochondrial membrane potential (Figure 3C). These results demonstrated that TDB-6 could induce mitochondrial-mediated apoptosis of LoVo cells in a dose-dependent manner.
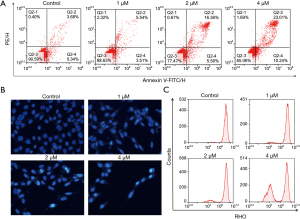
As a member of the IAP family, survivin can suppress the apoptosis of tumor cells. The Cancer Genome Atlas (TCGA) database was used to analyze the survivin mRNA levels in HCC and non-cancerous colon tissues, and the result in Figure 4A indicated that survivin mRNA levels were markedly higher in carcinoma tissues than in para-carcinoma tissues. Western blotting was conducted to investigate whether TDB-6 could suppress the expression of survivin. The result showed that TDB-6 obviously decreased the protein expression of survivin in a dose-dependent manner (Figure 4B,4C). YM-155 is a specific inhibitor of survivin and also decreased the survivin protein level at the indicated doses (Figure 4D,4E). Moreover, TDB-6 and YM-155 were used in combination on LoVo cells to investigate the targeted regulation of survivin by TDB-6. The results in Figure 4F,4G indicated that combined use of TDB-6 and YM-155 could suppress the protein expression of survivin more effectively compared to the single use of either reagent. These results preliminarily indicated that the induction of apoptosis in LoVo cells by TDB-6 correlated with its targeted suppression of survivin protein.
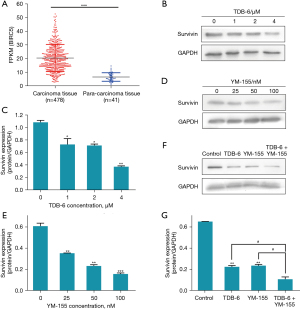
The apoptosis-induction effect of TDB-6 and YM-155 co-administration on LoVo cells was also investigated by Annexin-V FITC/PI analysis and hoechst staining. The result in Figure 5A showed that combined use of TDB-6 and YM-155 increased the percent of apoptotic cells more effectively than the single use of either reagent. A similar result was gained in the hoechst staining assay in Figure 5B. More condensed bright blue dots could be observed in the TDB-6 and YM-155 co-administration group compared to the single use of either reagent. These results indicated that TDB-6 could induce the apoptosis of LoVo cells by targeted regulation of survivin.
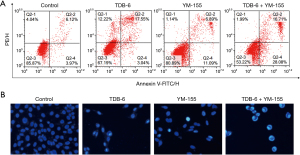
TDB-6 regulated the downstream signaling of survivin
The downstream signaling of survivin was further investigated by western blot analysis after TDB-6 treatment, and the results are shown in Figure 6. The expression of the anti-apoptotic protein Bcl-2 was significantly inhibited by TDB-6 in a dose-dependent manner (Figure 6A,6B). TDB-6 obviously increased the protein level of cleaved caspase-3, cleaved caspase-7, and cleaved caspase-9 (Figure 6A,6B,6D). The protein levels of cleaved-PARP and Cyto-C were also up-regulated by TDB-6.
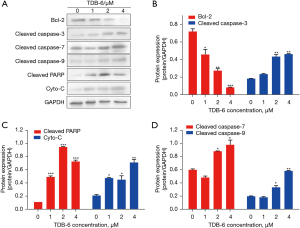
The in vivo regulation of TDB-6 on survivin and its downstream apoptosis related proteins was also evaluated by western blotting. TDB-6 could significantly inhibit the expression of survivin at the indicated doses (Figure 7A,7B). As an anti-apoptotic protein, Bcl-2 was also obviously suppressed by TDB-6 (Figure 7A,7B). After the inhibition of survivin by TDB-6, the protein levels of cleaved caspase-3, cleaved caspase-7, cleaved caspase-9, cleaved-PARP, and Cyto-C significantly increased (Figure 7A,7C,7D). These results indicated that TDB-6 could suppress the expression of survivin and its downstream anti-apoptotic protein and increase the expression of pro-apoptotic proteins in LoVo cells in vitro and in vivo.
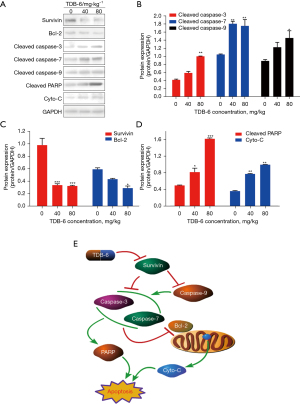
Discussion
In this study, we proved that TDB-6 could significantly suppress the proliferation and xenograft growth of LoVo cells in vitro and in vivo, and no visible damage was observed in BALB/c nude mice, suggesting that TDB-6 might be an effective and safe choice for the treatment of CRC.
Inducing apoptosis is an important part of anti-tumor research, and the effect of TDB-6 on LoVo cell apoptosis was investigated. Annexin-V FITC/PI analysis and hoechst staining revealed that TDB-6 could increase the percent of apoptotic LoVo cells in a dose-dependent manner. Moreover, the Δψm assay demonstrated that TDB-6 reduced the mitochondrial membrane potential of LoVo cells, indicating the occurrence of mitochondrial-mediated apoptosis.
Survivin is the smallest member of the IAP family and is overexpressed in many human cancers, including cancers of the lung, breast, stomach, esophagus, pancreas, prostate, liver, and ovary (25). The dramatic dysregulation of expression between normal adult tissues and cancers makes survivin an attractive and perhaps ideal target for cancer drug discovery. In this study, we found that the survivin mRNA level was almost 3-fold higher in carcinoma than in para-carcinoma tissues according to the analysis of TCGA database. Western blotting was further conducted to investigate the inhibitory effect of TDB-6 on survivin expression, and the results indicated that TDB-6 reduced the protein expression of survivin in a dose-dependent manner. Moreover, co-administration of TDB-6 and YM-155 could decrease the protein expression of survivin more effectively compared to the single use of either reagent, indicating a synergistic inhibitory effect. These results demonstrated targeted suppression of TDB-6 on survivin protein. In order to prove whether TDB-6 and YM-155 could induce the apoptosis of LoVo cells synergistically, Annexin-V FITC/PI analysis and hoechst staining were conducted, and the results showed that combined use of TDB-6 and YM-155 significantly enhanced the percent of apoptotic LoVo cells compared to the single use of either reagent. In conclusion, TDB-6 could induce the apoptosis of LoVo cells by targeted inhibition of survivin protein.
Caspases are a group of proteases with similar structures that participate in cell growth, differentiation, and apoptosis regulation. As a negative apoptosis regulator, survivin inhibits the activation of caspase molecules including caspase-3, caspase-7, and caspase-9 (26). Poly ADP-ribose polymerase (PARP) is a DNA repair enzyme and plays an important role in DNA damage repair and apoptosis. PARP can be cleaved by caspase-3 and caspase-7, leading to the loss of enzyme function and cell instability (27). Activation of caspase-3 and caspase-7 will suppress the anti-apoptotic protein Bcl-2 (18), which causes the increase of mitochondrial membrane permeability and the release of Cyto-C, promoting the apoptosis of tumor cells (Figure 7E). In this study, we proved that TDB-6 could suppress the expression of survivin protein and increase the protein level of cleaved caspase-3, cleaved caspase-7, and cleaved caspase-9 in LoVo cells. The protein level of Bcl-2 decreased significantly but the expression of cleaved-PARP and Cyto-C proteins increased obviously after TDB-6 treatment at the indicated doses in LoVo cells. Similar results were observed in the LoVo xenograft model. These results indicated that TDB-6 could regulate survivin downstream caspase/Bcl-2/Cyto-C signaling to induce mitochondria-mediated apoptosis of LoVo cells.
In conclusion, the obtained data indicated that TDB-6 could induce mitochondria-mediated apoptosis and inhibit the proliferation and growth of LoVo cells in vitro and in vivo via targeted regulation of survivin and its downstream caspase/Bcl-2/Cyto-C signaling. To sum up, TDB-6 might be a promising candidate for the inhibition of CRC.
Acknowledgments
Funding: This work was supported by the Key Scientific Research Project on Inheritance and Innovation of Traditional Chinese Medicine and Development of Qin Medicine (No. 2021-02-zz-015), the Natural Science Foundation of Shaanxi Province (No. 2022JM-605), and the Talent Support Program in Shaanxi Provincial People’s Hospital (No. 2021JY-29).
Footnote
Reporting Checklist: The authors have completed the ARRIVE reporting checklist. Available at https://jgo.amegroups.com/article/view/10.21037/jgo-22-780/rc
Data Sharing Statement: Available at https://jgo.amegroups.com/article/view/10.21037/jgo-22-780/dss
Conflicts of Interest: All authors have completed the ICMJE uniform disclosure form (available at https://jgo.amegroups.com/article/view/10.21037/jgo-22-780/coif). All authors report that this work was supported by the Key Scientific Research Project on Inheritance and Innovation of Traditional Chinese Medicine and Development of Qin Medicine (No. 2021-02-zz-015), the Natural Science Foundation of Shaanxi Province (No. 2022JM-605), and the Talent Support Program in Shaanxi Provincial People’s Hospital (No. 2021JY-29). The authors have no other conflicts of interest to declare.
Ethical Statement: The authors are accountable for all aspects of the work in ensuring that questions related to the accuracy or integrity of any part of the work are appropriately investigated and resolved. Animal experiments were performed under a project license (No. 2022-1434) granted by the Research Ethics Committee of Xi’an Jiaotong University, in compliance with the Chinese Academy of Sciences Animal Care and Use Committee guidelines for the care and use of animals.
Open Access Statement: This is an Open Access article distributed in accordance with the Creative Commons Attribution-NonCommercial-NoDerivs 4.0 International License (CC BY-NC-ND 4.0), which permits the non-commercial replication and distribution of the article with the strict proviso that no changes or edits are made and the original work is properly cited (including links to both the formal publication through the relevant DOI and the license). See: https://creativecommons.org/licenses/by-nc-nd/4.0/.
References
- Baidoun F, Elshiwy K, Elkeraie Y, et al. Colorectal Cancer Epidemiology: Recent Trends and Impact on Outcomes. Curr Drug Targets 2021;22:998-1009. [Crossref] [PubMed]
- Shi X, Zhu M, Gong Z, et al. Homoharringtonine suppresses LoVo cell growth by inhibiting EphB4 and the PI3K/AKT and MAPK/EKR1/2 signaling pathways. Food Chem Toxicol 2020;136:110960. [Crossref] [PubMed]
- Malki A, ElRuz RA, Gupta I, et al. Molecular Mechanisms of Colon Cancer Progression and Metastasis: Recent Insights and Advancements. Int J Mol Sci 2020;22:130. [Crossref] [PubMed]
- Kim M, Vogtmann E, Ahlquist DA, et al. Fecal Metabolomic Signatures in Colorectal Adenoma Patients Are Associated with Gut Microbiota and Early Events of Colorectal Cancer Pathogenesis. mBio. 2020;11:e03186-19. [Crossref] [PubMed]
- Ganesh K, Stadler ZK, Cercek A, et al. Immunotherapy in colorectal cancer: rationale, challenges and potential. Nat Rev Gastroenterol Hepatol 2019;16:361-75. [Crossref] [PubMed]
- Cheng X, Xu X, Chen D, et al. Therapeutic potential of targeting the Wnt/β-catenin signaling pathway in colorectal cancer. Biomed Pharmacother 2019;110:473-81. [Crossref] [PubMed]
- Biller LH, Schrag D. Diagnosis and Treatment of Metastatic Colorectal Cancer: A Review. JAMA 2021;325:669-85. [Crossref] [PubMed]
- Woolston A, Khan K, Spain G, et al. Genomic and Transcriptomic Determinants of Therapy Resistance and Immune Landscape Evolution during Anti-EGFR Treatment in Colorectal Cancer. Cancer Cell 2019;36:35-50.e9. [Crossref] [PubMed]
- Fukuoka S, Hara H, Takahashi N, et al. Regorafenib Plus Nivolumab in Patients With Advanced Gastric or Colorectal Cancer: An Open-Label, Dose-Escalation, and Dose-Expansion Phase Ib Trial (REGONIVO, EPOC1603). J Clin Oncol 2020;38:2053-61. [Crossref] [PubMed]
- Arai H, Battaglin F, Wang J, et al. Molecular insight of regorafenib treatment for colorectal cancer. Cancer Treat Rev 2019;81:101912. [Crossref] [PubMed]
- Pistritto G, Trisciuoglio D, Ceci C, et al. Apoptosis as anticancer mechanism: function and dysfunction of its modulators and targeted therapeutic strategies. Aging (Albany NY) 2016;8:603-19. [Crossref] [PubMed]
- Carneiro BA, El-Deiry WS. Targeting apoptosis in cancer therapy. Nat Rev Clin Oncol 2020;17:395-417. [Crossref] [PubMed]
- Wu Y, Chen M, Jiang J. Mitochondrial dysfunction in neurodegenerative diseases and drug targets via apoptotic signaling. Mitochondrion 2019;49:35-45. [Crossref] [PubMed]
- Rizwan H, Pal S, Sabnam S, et al. High glucose augments ROS generation regulates mitochondrial dysfunction and apoptosis via stress signalling cascades in keratinocytes. Life Sci 2020;241:117148. [Crossref] [PubMed]
- Li H, Xiao Y, Tang L, et al. Adipocyte Fatty Acid-Binding Protein Promotes Palmitate-Induced Mitochondrial Dysfunction and Apoptosis in Macrophages. Front Immunol 2018;9:81. [Crossref] [PubMed]
- Srivastav AK, Dubey D, Chopra D, et al. Oxidative stress-mediated photoactivation of carbazole inhibits human skin cell physiology. J Cell Biochem 2020;121:1273-82. [Crossref] [PubMed]
- Miliaraki M, Briassoulis P, Ilia S, et al. Survivin and caspases serum protein levels and survivin variants mRNA expression in sepsis. Sci Rep 2021;11:1049. [Crossref] [PubMed]
- Eslami F, Mahdavi M, Babaei E, et al. Down-regulation of Survivin and Bcl-2 concomitant with the activation of caspase-3 as a mechanism of apoptotic death in KG1a and K562 cells upon exposure to a derivative from ciprofloxacin family. Toxicol Appl Pharmacol 20205;409:115331.
- Peery RC, Liu JY, Zhang JT. Targeting survivin for therapeutic discovery: past, present, and future promises. Drug Discov Today 2017;22:1466-77. [Crossref] [PubMed]
- Shi CT, Ma J, Shi QF, et al. High Survivin and Low Zinc Finger of the Cerebellum 1 Expression Indicates Poor Prognosis in Triple-negative Breast Carcinoma. J Breast Cancer 2019;22:248-59. [Crossref] [PubMed]
- Kapiris I, Nastos K, Karakatsanis A, et al. Survivin expression in hepatocellular carcinoma. Correlation with clinicopathological characteristics and overall survival. J BUON 2019;24:1934-42. [PubMed]
- Narayanankutty A. Natural Products as PI3K/ Akt Inhibitors: Implications in Preventing Hepatocellular Carcinoma. Curr Mol Pharmacol 2021;14:760-9. [Crossref] [PubMed]
- Montopoli M, Bertin R, Chen Z, Bolcato J, Caparrotta L, Froldi G. Croton lechleri sap and isolated alkaloid taspine exhibit inhibition against human melanoma SK23 and colon cancer HT29 cell lines. J Ethnopharmacol 2012;144:747-53. [Crossref] [PubMed]
- Liu R, Yu R, Cui Y, et al. Inhibitory effect of taspine derivative TAD1822-7 on tumor cell growth and angiogenesis via suppression of EphrinB2 and related signaling pathways. Acta Pharm 2019;69:423-31. [Crossref] [PubMed]
- Li F, Aljahdali I, Ling X. Cancer therapeutics using survivin BIRC5 as a target: what can we do after over two decades of study? J Exp Clin Cancer Res 2019;38:368. [Crossref] [PubMed]
- Sam MR, Ghoreishi S. Prodigiosin produced by Serratia marcescens inhibits expression of MMP-9 and survivin and promotes caspase-3 activation with induction of apoptosis in acute lymphoblastic leukaemia cells. J Appl Microbiol 2018;125:1017-29. [Crossref] [PubMed]
- Xu P, Cai X, Zhang W, et al. Flavonoids of Rosa roxburghii Tratt exhibit radioprotection and anti-apoptosis properties via the Bcl-2(Ca(2+))/Caspase-3/PARP-1 pathway. Apoptosis 2016;21:1125-43. [Crossref] [PubMed]