Atractylenolide I inhibited the development of malignant colorectal cancer cells and enhanced oxaliplatin sensitivity through the PDK1-FoxO1 axis
Introduction
Colorectal cancer (CRC) is a highly invasive tumor in the mucosa and glands of the colon and rectum (1). The occurrence rate and death rate of CRC have increased in recent years (1,2). Indeed, the fatality rate of CRC ranks 2nd among all cancers (3). The early detection rate of CRC is low (4), and the surgical treatment effect of advanced CRC patients is poor. The primary treatment for unresectable metastatic CRC is systemic therapy (cytotoxic chemotherapy, biologic therapy such as antibodies to cellular growth factors including cetuximab and panitumumab, immunotherapy and their combinations, ) (5). Currently, chemotherapy is the common clinical treatment for patients with advanced CRC (5,6). Chemotherapeutic drugs can effectively kill tumor cells; however, they can also kill normal cells, which can lead to various adverse reactions such as chemotherapy resistance (7). Oxaliplatin resistance is the major obstacle to the anticancer effects of chemotherapy in colorectal cancer patients. The molecular mechanisms of oxaliplatin resistance are proved to be associated with multifactoriality activation at the same time, such as intracellular transport and detoxification, alterations in DNA repair mechanisms, epigenetic, and cell death mechanisms (8). The question of how to reduce the adverse reactions of chemotherapeutic drugs and improve patients’ tolerance to chemotherapy is an important area of clinical research.
Atractylodes is the dried rhizome of Atractylodes macrocephala Koidz (Atractylodes macrocephala Koidz), which is the most effective part of Chinese herbal medicine in treating diseases (9). Modern medical research has showed that atractylodes have anti-aging and anti-tumor effects, promote cellular immunity, liver protection, diuresis, hypoglycemia, anticoagulant, and protein synthesis and benefit the gallbladder (10,11). The chemical components of the rhizome of the atractylode, Atractylenolide I (AT-I), include volatile oil, polysaccharides, and lactones (12). Among these chemical components, volatile oil has the most significant effect, while AT-I is a natural sesquiterpenoid that has an immunomodulatory affect (13). AT-I is a type of semi terpene lactone derivative that is commonly used in atractylodes. Studies have shown that AT-I effectively suppresses the CRC process by inducing apoptosis of human CRC cells through activation of the mitochondria-dependent pathway and suppressing proliferation and glycolysis (14-16). Thus, AT-I has important research significance in the treatment of CRC.
To gain a deeper understanding of the role of AT-I in CRC, we conducted a literature review and found that AT-I can inhibit the expression and promoter activity of pyruvate dehydrogenase kinase 1 (PDK1) in lung cancer cells (17). PDK1 is a serine-threonine kinase that promotes Forkhead box protein O1 (FoxO1) phosphorylation, and its expression is upregulated in CRC and promotes cell growth and glycolysis (18). PDK1 suppressors enhanced oxaliplatin sensitivity (19). Additionally, p-FoxO1 has been shown to be positively correlated with angiogenesis markers in CRC, and p-FoxO1-positive patients have a worse survival rate than p-FoxO1-negative patients (20). Inosine-5'-monophosphate dehydrogenase 2 (IMPDH2) has been shown to promote the development of CRC by promoting the phosphorylation of FoxO1 (21). In bladder cancer, p-FoxO1 expression is highly expressed in cisplatin-resistant strains (22). Thus, it was hypothesized that AT-I would inhibit the malignant development of CRC cells and increase oxaliplatin sensitivity by inhibiting FoxO1 phosphorylation by decreasing PDK1. In this study, we demonstrated the inhibitory role of AT-I in CRC and explored the mechanism involved in enhancing oxaliplatin sensitivity through the PDK1-FoxO1 axis. We present the following article in accordance with the MDAR reporting checklist (available at https://jgo.amegroups.com/article/view/10.21037/jgo-22-910/rc).
Methods
Cell culture and treatment
Human colorectal cancer cells HCT116 cells supplied by Shanghai Chinese Academy of Science were cultured in Dulbecco's Modified Eagle Medium (DMEM) (Gibco) comprising 10% fetal bovine serum (FBS; Gibco) and 10% antibiotics (Hyclone, UT, USA) in 37 ℃-incubator with 5% carbon dioxide (CO2). AT-I (0, 25, 50, 100, and 200 µM) was used to treat the HCT116 cells at 37 ℃ for 72 h. Next, 1 µM of FoxO1 inhibitor (AS1842856) or 3 µmol/L of Oxaliplatin was used to treat the HCT116 cells for 24 h at 37 ℃.
Cell transfection
The overexpression-PDK1 (Oe-PDK1) and negative control (Oe-NC) were acquired from Shanghai GenePharma Co., Ltd. As per the instructions for the Lipofectamine 2000 by Thermo Fisher Scientific, the aforementioned plasmids were transduced into cells plated in 6-well plates. Transfection efficacy was tested 48 h later.
Detection of cell viability
The density of the HCT116 cells inoculated in the 96-well plates was 8.0×103 cells per well. After 24 h of incubation, 10 µL of Cell Counting Kit-8 (CCK-8) reagent was used to treat the HT-22 cells at 37 ℃ for 1 h. A microplate reader (BioTek, Winooski, VT, USA) was used to record Optical Density (OD) 450 nm.
Colony formation assays
Following the incubation, the HCT116 cells plated in the 6-well plates were stained with 75% methanol immobilization for 30 min and 0.5% crystal violet staining for 15 min. An optical microscope (IX51; Olympus Corporation) was used to monitor the stained cells.
TUNEL assays
The treated HCT116 cells were exposed to 4% paraformaldehyde and 0.1% Triton X-100 (Solarbio) at room temperature for 30 min and 5 min, respectively. As per the manufacturer’s instructions, terminal deoxynucleotidyl-transferase-mediated dUTP nick end labeling (TUNEL) assay kit (Roche) was used. The apoptotic ratio was calculated as the ratio of the number of TUNEL-positive cells to the total number of 4',6-diamidino-2-phenylindole (DAPI)-positive cells.
Cell migration and invasion
After the cells inoculated in the 6-well plates (5×105 cells/well) reached 100% confluence, a scratch was made. The cells were then cultivated in serum-starved Roswell Park Memorial Institute medium (RPMI)-1640 medium for 24 h, after which the migratory distance of cells was observed under light microscope (Olympus, Japan) and analyzed by Image J software.
To examine the cell invasion, the upper chamber was coated with Matrigel (8.0 µm membrane, 24-well plate, Corning, USA). The cells in the upper chamber (1×104 cells/well) were cultured in 400 µL of serum-starved DMEM medium. RPMI-1640 (600 µL) comprising 10% FBS was added to the lower chamber. The cells in the bottom of the upper chamber were fixed in 90% ethanol for 30 min 24 h later. Crystal violet cell staining (0.1%) was performed for 10 min. Finally, a light microscope was used to photograph the invaded cells.
Tube formation assays
Matrigel (50 µL; Becton Dickinson Labware, Bedford, MA) was used to coat the 96-well plate and left to solidify at 37 ℃ for 1 hour. Human umbilical vein endothelial cells (HUVECs) were seeded in the plates with Matrigel and treated with AT-I and cultured for 24 hours. A light microscope was used to photograph angiogenesis in 5 fields chosen at random.
Western blot analysis
The protein isolation of the tm HT-22 cells was performed by applying RIPA lysis buffer (Beyotime) supplemented with protease inhibitor. The protein samples (20 µg) were subjected to 10% sodium dodecyl sulfate-polyacrylamide gel electrophoresis (SDS-PAGE) to separate it. After the polyvinylidene difluoride (PVDF) transfer, the cells were blocked with 5% skim milk for 2 h at room temperature. The cells were then cultivated overnight with primary antibodies at 4 ℃ and exposed for 2 h to goat anti-Rabbit IgG H&L (HRP) secondary antibodies (cat. no. ab6759; 1:5,000; Abcam) at room temperature. An enhanced chemiluminescence (ECL) kit was used, and Image J Software (NIH, Bethesda, MD, USA) was used to analyze the band intensities. The following primary antibodies were used: PDK1 (cat. no. ab202468; 1:2,000; Abcam), p-FoxO1 (cat. no. ab131339; 1:1,000; Abcam), FoxO1 (cat. no. ab179450; 1:1,000; Abcam), Bcl2 (cat. no. ab32124; 1:1,000; Abcam), Bax (cat. no. ab32503; 1:1,000; Abcam), MMP2 (cat. no. ab92536; 1:1,000; Abcam), vimentin (cat. no. ab92547; 1:1,000; Abcam), E-cadherin (cat. no. ab40772; 1:10,000; Abcam), VEGFA (cat. no. ab46154; 1:1,000; Abcam), and VEGFAR2 (cat. no. #96981; 1,000; Cell Signaling Technology).
Statistical analysis
SPSS 13.0 software was used for the statistical analysis. All the data are reported as the mean ± standard error of mean. A 1-way analysis of variance and Dunnett’s post-hoc test were used for the multiple group comparisons. A P value <0.05 was considered statistically significant. All the experiments were independently repeated in triplicate, and all the experiments were repeated in triplicate.
Results
AT-I inhibits HCT116 cell viability through the PDK1/FoxO1 axis
The structure of AT-I was determined using PubChem (https://pubchem.ncbi.nlm.nih.gov/) (see Figure 1A). CCK-8 assays were used to detect the HCT116 cell activity based on the administration of increasing doses (25, 50, and 100 µM) of AT-I. As Figure 1B shows, AT-I inhibited cell activity. As Figure 1C shows, the expression of PDK1 and p-FoxO1 was downregulated by AT-I stimulation, but no obvious alterations in FOXO1 expression were observed. These results suggested that AT-I inhibited the viability of the HCT116 cancer cells, decreased the expression of PDK1, and inhibited FoxO1 phosphorylation in a concentration-dependent manner.
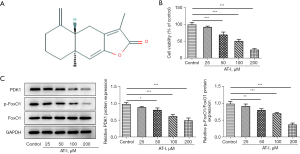
The concentration 100 µm was selected for the subsequent experiment. The function of AT-I is presented in Figure 2A,2B. The successful transfection of Oe-PDK1 in the HCT116 cells was established using western blot and RNA extraction and quantitative real-time PCR (qRT-PCR). Further, a FoxO1 suppressor (1 µM, AS1842856) was used to treat cells. As Figure 2C shows, the AT-I stimulation diminished cell viability. Notably, Oe-PDK1 increased the cell viability of the HCT116 cells, while AS1842856 reversed this effect. Additionally, the anti-proliferative activity of AT-I was further evaluated using colony formation assays. As Figure 2D shows, there was a significant decrease in the colony number after treatment with AT-I, and the colony number was increased in Oe-PDK1 group, but AS1842856 reversed this trend. These results suggested that AT-I inhibits HCT116 cell viability through the PDK1/FoxO1 axis.
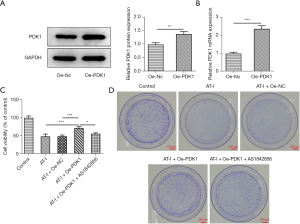
AT-I inhibits HCT116 cell apoptosis through the PDK1/FoxO1 axis
Conversely, the TUNEL staining assay results showed that AT-I significantly increased the apoptosis of the HCT116 cells, Oe-PDK1 reduced the apoptosis level, and AS1842856 reversed the apoptosis level of HCT116 in the T-I+Oe-PDK1 group (see Figure 3A,3B). AT-I significantly decreased anti-apoptotic protein Bcl-2 expression, but increased pro-apoptotic protein BAX expression relative to the control group (see Figure 3C). Thus, AT-I appears to increase HCT116 cell apoptosis. Collectively, these findings indicated that AT-I inhibits HCT116 cell apoptosis through the PDK1/FoxO1 axis.
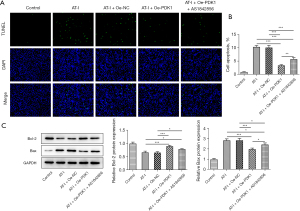
AT-I inhibits HCT116 cell migration and invasion through the PDK1/FoxO1 axis
The results of the wound-healing and Transwell assays showed that AT-I significantly reduced the migration and invasion of HCT116 cells compared to the control group, while Oe-PDK1 reversed this trend, and the cell migration and invasion were decreased in the AT-I+Oe-PDK1+AS1842856 group (see Figure 4A-4D). Additionally, AT-I significantly decreased MMP2 and vimentin expression, and increased E-cadherin expression relative to the control group (see Figure 4E). Thus, the results showed that AT-I prevents CRC metastasis through the PDK1/FoxO1 axis.
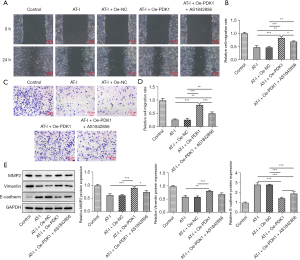
Upregulation of PDK1 reduces the inhibitory effect of AT-I on HCT116 angiogenesis
A tubule formation test was conducted to detect angiogenesis. The tubule formation rate of HUVECs of the AT-I group was significantly decreased compared to that of the control group, and the tubule formation rate was significantly increased after OE-PDK1 was added. AS1842856 reversed this trend (see Figure 5A). Further, AT-I significantly decreased VEGFA and VEGFAR2 expression relative to the control group (see Figure 5B,5C). Thus, the results showed that the upregulation of PDK1 reduces the inhibitory effect of AT-I on HCT116 angiogenesis.
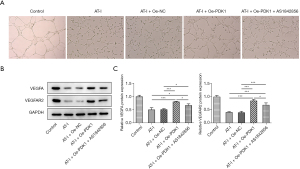
AT-I enhances oxaliplatin sensitivity via the PDK1/FoxO1 axis
We then examined the effect of AT-I on oxaliplatin sensitivity. The CCK-8 assay results showed that in the oxaliplatin-administrated HCT116 cells, the activity was further decreased after the AT-I treatment, but increased after PDK1 elevation. AS1842856 reversed this trend (see Figure 6A). Conversely, PDK1 decreased the AT-I administration-stimulated apoptosis of the oxaliplatin-administrated HCT116 cells. AS1842856 reversed this trend (see Figure 6B,6C). Thus, the results showed that AT-I enhances oxaliplatin sensitivity through the PDK1/FoxO1 axis.
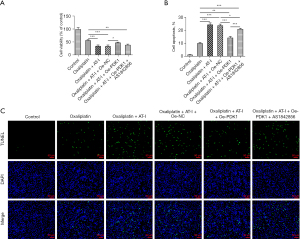
Discussion
Newly discovered natural drugs are gradually being applied in CRC therapy (23-25). AT-I has anti-cancer effects (15,26). In this study, we evaluated the role of AT-I in CRC and showed that AT-I mediated the PDK1/FoxO1 axis by inhibiting HCT116 cell viability, migration, and invasion. Additionally, the upregulation of PDK1 decreased the inhibitory effect of AT-I on HCT116 angiogenesis, and AT-I enhanced oxaliplatin sensitivity through the PDK1/FoxO1 axis, which provide a novel fundamental insight into how AT-I against CRC and gives us clues for future therapeutic strategy for CRC by reducing oxaliplatin resistance.
AT-I is one of the main natural ingredients of atractylodes, and has received attention due to its anti-inflammatory, anti-allergic, and anti-tumor effects, and other properties (12,27). There is substantial evidence that AT-I effectively inhibits tumor cell proliferation and aggravates apoptosis by blocking the Notch pathway (28). Previous research has shown that AT-I can induce ROS-mediated apoptosis in leukemia cells (29). Additionally, AT-I has been shown to increase anti-proliferation, induce cell differentiation and inhibit cell migration in melanoma cells through the Ras/ERK and PI3K/Akt signal transduction pathways (30). A recent study has shown that the inhibition of PDK1 and lncRNA HOTAIR-mediated EZH2 gene expression increases the inhibitory effect of AT-I and erlotinib on human lung cancer cells and inhibits the growth of lung cancer cells by activating ERK1/2 (31). This study confirmed that AT-I inhibited CRC process. We also showed that PDK1-FoxO1 expression was decreased in the group treated with AT-I. Thus, AT-I affects the occurrence and development of CRC through the PDK1-FoxO1 pathway.
Platinum-based drugs, a class of classic anti-cancer drugs, play a pivotal role in clinical chemotherapy and are commonly used in the treatment of cancers (32). Following improvements in 1st-generation cisplatin and 2nd-generation carboplatin, 3rd-generation platinum-based drugs, which use oxaliplatin as a substitute, have been developed (33). Oxaliplatin exerts anti-cancer effects by interfering with deoxyribonucleic acid (DNA) replication and the transcription system through the formation of cross-forks with nuclear DNA (19,34). Oxaliplatin is widely used, as it is better tolerated and has less toxic effects and side effects than other platinum compounds, but it still has some toxic effects and side effects, such as neurotoxicity, blood and gastrointestinal toxicity, neutropenia and vomiting, which undoubtedly limit the available dose range (35). Often, a combination of drugs is used to combat the toxicity and drug resistance of chemotherapy drugs. Our study showed that the upregulation of PDK1 reduces the inhibition of angiogenesis of HCT116 by AT-I, and AT-I enhances oxaliplatin sensitivity through the PDK1/FoxO1 axis, further demonstrating the suppressive role of AT-I in CRC process. Here, there are several limitations in our study. Our results were only supported by in vitro experiments. Thus, further experiments using animals, as well as clinical studies, are required to confirm our findings. In addition, the effects of AT-I in enhancing oxaliplatin sensitivity in CRC might be associated with glycolysis as previous study has revealed AT-I inhibited CRC by suppressing glycolysis. We will perform experiments on the effect of AT-I on glycolysis in the future study. Moreover, AT-I concentration used in this study is different from that in animals and human, thus the result of this study might not be suitable for in vivo experiments and clinical trials. We will explore the suitable concentration of AT-I for further experiments in animals and clinical studies to confirm our findings.
Conclusions
Our study showed that AT-I inhibits the malignant development of CRC cells and increases oxaliplatin sensitivity by decreasing PDK1 and inhibiting FoxO1 phosphorylation. Our findings may provide novel targets for CRC therapy.
Acknowledgments
Funding: None.
Footnote
Reporting Checklist: The authors have completed the MDAR reporting checklist. Available at https://jgo.amegroups.com/article/view/10.21037/jgo-22-910/rc
Data Sharing Statement: Available at https://jgo.amegroups.com/article/view/10.21037/jgo-22-910/dss
Conflicts of Interest: All authors have completed the ICMJE uniform disclosure form (available at https://jgo.amegroups.com/article/view/10.21037/jgo-22-910/coif). The authors have no conflicts of interest to declare.
Ethical Statement: The authors are accountable for all aspects of the work in ensuring that questions related to the accuracy or integrity of any part of the work are appropriately investigated and resolved.
Open Access Statement: This is an Open Access article distributed in accordance with the Creative Commons Attribution-NonCommercial-NoDerivs 4.0 International License (CC BY-NC-ND 4.0), which permits the non-commercial replication and distribution of the article with the strict proviso that no changes or edits are made and the original work is properly cited (including links to both the formal publication through the relevant DOI and the license). See: https://creativecommons.org/licenses/by-nc-nd/4.0/.
References
- Fakih MG. Metastatic colorectal cancer: current state and future directions. J Clin Oncol 2015;33:1809-24. [Crossref] [PubMed]
- Li J, Ma X, Chakravarti D, et al. Genetic and biological hallmarks of colorectal cancer. Genes Dev 2021;35:787-820. [Crossref] [PubMed]
- Zarkavelis G, Boussios S, Papadaki A, et al. Current and future biomarkers in colorectal cancer. Ann Gastroenterol 2017;30:613-21. [Crossref] [PubMed]
- Siegel RL, Miller KD, Goding Sauer A, et al. Colorectal cancer statistics, 2020. CA Cancer J Clin 2020;70:145-64. [Crossref] [PubMed]
- Biller LH, Schrag D. Diagnosis and Treatment of Metastatic Colorectal Cancer: A Review. JAMA 2021;325:669-85. [Crossref] [PubMed]
- Shike M, Winawer SJ, Greenwald PH, et al. Primary prevention of colorectal cancer. The WHO Collaborating Centre for the Prevention of Colorectal Cancer. Bull World Health Organ 1990;68:377-85. [PubMed]
- Zeng X, Liu L, Zheng M, et al. Pantoprazole, an FDA-approved proton-pump inhibitor, suppresses colorectal cancer growth by targeting T-cell-originated protein kinase. Oncotarget 2016;7:22460-73. [Crossref] [PubMed]
- Martinez-Balibrea E, Martínez-Cardús A, Ginés A, et al. Tumor-Related Molecular Mechanisms of Oxaliplatin Resistance. Mol Cancer Ther 2015;14:1767-76. [Crossref] [PubMed]
- Dong H, He L, Huang M, et al. Anti-inflammatory components isolated from Atractylodes macrocephala Koidz. Nat Prod Res 2008;22:1418-27. [Crossref] [PubMed]
- Li CQ, He LC, Dong HY, et al. Screening for the anti-inflammatory activity of fractions and compounds from Atractylodes macrocephala koidz. J Ethnopharmacol 2007;114:212-7. [Crossref] [PubMed]
- Gu S, Li L, Huang H, et al. Antitumor, Antiviral, and Anti-Inflammatory Efficacy of Essential Oils from Atractylodes macrocephala Koidz. Produced with Different Processing Methods. Molecules 2019;24:2956. [Crossref] [PubMed]
- Long F, Lin H, Zhang X, et al. Atractylenolide-I Suppresses Tumorigenesis of Breast Cancer by Inhibiting Toll-Like Receptor 4-Mediated Nuclear Factor-κB Signaling Pathway. Front Pharmacol. 2020;11:598939. [Crossref] [PubMed]
- Ji G, Chen R, Zheng J. Atractylenolide I inhibits lipopolysaccharide-induced inflammatory responses via mitogen-activated protein kinase pathways in RAW264.7 cells. Immunopharmacol Immunotoxicol 2014;36:420-5. [Crossref] [PubMed]
- Chan KWK, Chung HY, Ho WS. Anti-Tumor Activity of Atractylenolide I in Human Colon Adenocarcinoma In Vitro. Molecules 2020;25:212. [Crossref] [PubMed]
- Li Y, Wang Y, Liu Z, et al. Atractylenolide I Induces Apoptosis and Suppresses Glycolysis by Blocking the JAK2/STAT3 Signaling Pathway in Colorectal Cancer Cells. Front Pharmacol 2020;11:273. [Crossref] [PubMed]
- Wang K, Huang W, Sang X, et al. Atractylenolide I inhibits colorectal cancer cell proliferation by affecting metabolism and stemness via AKT/mTOR signaling. Phytomedicine 2020;68:153191. [Crossref] [PubMed]
- Xiao Q, Zheng F, Wu J, et al. Activation of ERK and Mutual Regulation of Stat3 and SP1 Contribute to Inhibition of PDK1 Expression by Atractylenolide-1 in Human Lung Cancer Cells. Cell Physiol Biochem 2017;43:2353-66. [Crossref] [PubMed]
- Liu S, Huang F, Ye Q, et al. SPRY4-IT1 promotes survival of colorectal cancer cells through regulating PDK1-mediated glycolysis. Anim Cells Syst (Seoul) 2020;24:220-7. [Crossref] [PubMed]
- Wang Y, Zhang D, Li Y, et al. MiR-138 Suppresses the PDK1 Expression to Decrease the Oxaliplatin Resistance of Colorectal Cancer. Onco Targets Ther 2020;13:3607-18. [Crossref] [PubMed]
- Ko YS, Kim NY, Pyo JS. Clinicopathological significance and angiogenic role of the constitutive phosphorylation of the FOXO1 transcription factor in colorectal cancer. Pathol Res Pract 2020;216:153150. [Crossref] [PubMed]
- Duan S, Huang W, Liu X, et al. IMPDH2 promotes colorectal cancer progression through activation of the PI3K/AKT/mTOR and PI3K/AKT/FOXO1 signaling pathways. J Exp Clin Cancer Res 2018;37:304. [Crossref] [PubMed]
- Ide H, Goto T, Teramoto Y, et al. FOXO1 inactivation induces cisplatin resistance in bladder cancer. Cancer Sci 2020;111:3397-400. [Crossref] [PubMed]
- Yu Y, Yan R, Chen X, et al. Paeonol suppresses the effect of ox-LDL on mice vascular endothelial cells by regulating miR-338-3p/TET2 axis in atherosclerosis. Mol Cell Biochem 2020;475:127-35. [Crossref] [PubMed]
- Qian J, Fang D, Lu H, et al. Tanshinone IIA promotes IL2-mediated SW480 colorectal cancer cell apoptosis by triggering INF2-related mitochondrial fission and activating the Mst1-Hippo pathway. Biomed Pharmacother 2018;108:1658-69. [Crossref] [PubMed]
- Wen C, Chen J, Zhang D, et al. Pseudolaric acid B induces mitotic arrest and apoptosis in both 5-fluorouracil-sensitive and -resistant colorectal cancer cells. Cancer Lett 2016;383:295-308. [Crossref] [PubMed]
- Xu H, Van der Jeught K, Zhou Z, et al. Atractylenolide I enhances responsiveness to immune checkpoint blockade therapy by activating tumor antigen presentation. J Clin Invest 2021;131:146832. [Crossref] [PubMed]
- Huang JM, Zhang GN, Shi Y, et al. Atractylenolide-I sensitizes human ovarian cancer cells to paclitaxel by blocking activation of TLR4/MyD88-dependent pathway. Sci Rep 2014;4:3840. [Crossref] [PubMed]
- Ma L, Mao R, Shen K, et al. Atractylenolide I-mediated Notch pathway inhibition attenuates gastric cancer stem cell traits. Biochem Biophys Res Commun 2014;450:353-9. [Crossref] [PubMed]
- Huang HL, Lin TW, Huang YL, et al. Induction of apoptosis and differentiation by atractylenolide-1 isolated from Atractylodes macrocephala in human leukemia cells. Bioorg Med Chem Lett 2016;26:1905-9. [Crossref] [PubMed]
- Fu XQ, Chou JY, Li T, et al. The JAK2/STAT3 pathway is involved in the anti-melanoma effects of atractylenolide I. Exp Dermatol 2018;27:201-4. [Crossref] [PubMed]
- Xiao Q, Zheng F, Tang Q, et al. Repression of PDK1- and LncRNA HOTAIR-Mediated EZH2 Gene Expression Contributes to the Enhancement of Atractylenolide 1 and Erlotinib in the Inhibition of Human Lung Cancer Cells. Cell Physiol Biochem 2018;49:1615-32. [Crossref] [PubMed]
- Liu N, Wu C, Jia R, et al. Traditional Chinese Medicine Combined With Chemotherapy and Cetuximab or Bevacizumab for Metastatic Colorectal Cancer: A Randomized, Double-Blind, Placebo-Controlled Clinical Trial. Front Pharmacol 2020;11:478. [Crossref] [PubMed]
- Wang FW, Cai MY, Mai SJ, et al. Ablation of EIF5A2 induces tumor vasculature remodeling and improves tumor response to chemotherapy via regulation of matrix metalloproteinase 2 expression. Oncotarget 2014;5:6716-33. [Crossref] [PubMed]
- Na YS, Kim SM, Jung KA, et al. Effects of the HDAC inhibitor CG2 in combination with irinotecan, 5-fluorouracil, or oxaliplatin on HCT116 colon cancer cells and xenografts. Oncol Rep 2010;24:1509-14. [PubMed]
- Cheng C, Xie Z, Li Y, et al. PTBP1 knockdown overcomes the resistance to vincristine and oxaliplatin in drug-resistant colon cancer cells through regulation of glycolysis. Biomed Pharmacother 2018;108:194-200. [Crossref] [PubMed]
(English Language Editor: L. Huleatt)