LncRNA NORAD mediates KMT2D expression by targeting miR-204-5p and affects the growth of gastric cancer
Highlight box
Key findings
• NORAD supports gastric cancer development and progression via KMT2D/miR-204-5p axis.
What is known and what is new?
• LncRNAs have been recognized as having a critical role in gastric cancer development and progression.
• NORAD acts as a competing endogenous RNA for miR-204-5p, thereby leading to the upregulation of KMT2D in gastric cancer.
What is the implication, and what should change now?
• Our study uncovers the roles of NORAD and may lead to the development of novel therapeutic strategies for gastric cancer, but further clinical studies are needed.
Introduction
Gastric cancer (GC) is one of the most common malignant tumors worldwide, and is 6th leading cause of cancer related-death (1,2). As most GC patients are at an advanced stage at the time of diagnosis, the 5-year survival rate of patients remains low, particularly in those with local and distant metastases (3,4). Thus, improved early diagnosis methods and survival outcomes for GC are urgently needed.
Protein-coding genes regulate many cell processes, including differentiation, metabolism, and tumorigenesis, and have been studied extensively in recent years (5,6). However, with the development of human genome sequencing, non-coding ribonucleic acids (ncRNAs) have gradually received increasing attention (7,8). There is emerging evidence that long-non-coding RNAs (lncRNAs) are key players in a multitude of cellular processes, and lead to the aberrant expression of gene products linked to the progression of diverse human cancers (9,10). However, the role of lncRNAs in GC and their molecular mechanisms remain unclear.
Non-coding RNA activated by deoxyribonucleic acid (DNA) damage (NORAD) is a newly discovered lncRNA that maps to 20q11.23 in humans and has been reported to be an oncogenic factor in a number of human cancers (11-16). For example, a recent study revealed that NORAD is upregulated in GC tissues and promotes GC cell growth in vitro (17). Another study reported that silencing NORAD suppresses cell proliferation and invasion via the positive modulation of the Ras homolog family member A (RhoA)/Rho-associated coiled-coil-containing protein kinase 1 (ROCK1) pathway in GC (18). Micro-RNAs (miRNAs) are endogenous short-chain ncRNA molecules that are involved in the development and progression of human cancers (19,20). One of the most well-established biological roles of lncRNAs is to act as competitive endogenous RNAs (ceRNAs) to sponge miRNAs (21,22). Interestingly, NORAD also functions as a ceRNA in many cancers (23-26). MiR-204-5p is a miRNA that has been implicated in the development and progression of human cancers, including GC (27-30). However, to date, very few studies have examined the relationship between NORAD and miR-204-5p in GC. In the present study, we sought to comprehensively elucidate the functional roles of the NORAD/miR-204-5p axis and its molecular mechanisms in GC, and its potential as therapeutic targets for the disease. We present the following article in accordance with the ARRIVE reporting checklist (available at https://jgo.amegroups.com/article/view/10.21037/jgo-22-1014/rc).
Methods
Clinical samples
Our sample cohort comprised 60 pairs of matched normal and GC tissues obtained from the Affiliated Dongguan People’s Hospital, Southern Medical University (Dongguan People’s Hospital). After liquid nitrogen freezing, all the tissue samples were stored at –80 ℃ in a cryogenic freezer. All the participants in this study signed written informed consent forms. This study was approved by the Affiliated Dongguan People’s Hospital, Southern Medical University (Dongguan People’s Hospital) (No. KYKT-2020-026) and was conducted in accordance with the Declaration of Helsinki (as revised in 2013).
qRT-PCR assays
Cellular RNA was extracted using TRIzol Reagent (Invitrogen, CA, USA) in accordance with the manufacturer’s instructions. The PrimeScript reverse transcriptase (RT) Reagent Kit (TaKaRa Biotechnology) was used for total RNA reverse transcription. The expression of NORAD and KMT2D was detected using a LightCycler 480 Instrument (Roche, Basel, Switzerland) and SYBR green polymerase chain reaction (PCR) kit (TaKaRa Biotechnology), and Glyceraldehyde-3-phosphate dehydrogenase (GAPDH) was used as an internal control. The relative expression of miR-204-5p was measured using TaqMan miRNA assays (Applied Biosystems), and U6 was used as an internal control. All the primer sequences are listed in Table 1.
Table 1
Gene name | Primer sequences |
---|---|
NORAD forward primer | 5'-CTGGATTGAAGGCAGAGAAGGAAGG-3' |
NORAD reverse primer | 5'-TTGTCCACCACATACACAGCACTG-3' |
GAPDH forward primer | 5'-GTCAACGGATTTGGTCTGTATT-3' |
GAPDH reverse primer | 5'-AGTCTTCTGGGTGGCAGTGAT-3' |
miR-204-5p forward primer | 5'-ACTATGGCTTCCCTTTGTCATCC-3' |
miR-204-5p reverse primer | 5'-AGTGCAGGGTCCGAGGTATT-3' |
U6 forward primer | 5'-CTCGCTTCGGCAGCACA-3' |
U6 reverse primer | 5'-AACGCTTCACGAATTTGCGT-3' |
KMT2D forward primer | 5'-TGACAAGTGTGAATCCCGTGAAG-3' |
KMT2D reverse primer | 5'-CATTTCATCCGTTGTTACGAAG-3' |
NORAD, non-coding RNA activated by deoxyribonucleic acid damage; GAPDH, glyceraldehyde-3-phosphate dehydrogenase; KMT2D, Lysine Methyltransferase 2D.
Cell cultures
The cell lines MGC-803, SGC-7901, BGC-823, MKN-45, AGS, and GES-1 were purchased from the Chinese Academy of Sciences (Shanghai, China). The MGC-803, BGC-823, and MKN-45 cells were cultured in Roswell Park Memorial Institute (RPMI) 1640. SGC-7901, AGS, and the GES-1 cells were cultured in Dulbecco’s Modified Eagle Medium(DMEM) supplemented with 10% fetal bovine serum (FBS; Gibco, Rockville, MD, USA), 100 U/mL of penicillin, and 100 mg/mL of streptomycin (Invitrogen, Carlsbad, CA, USA) at 37 ℃ with 5% carbon dioxide.
Cell transfection
Short-hairpin RNA (shRNA) and NC shRNA specific for NORAD were obtained from Sangon Biotech (Guangzhou, China). The GC cells were infected with a mixture of lentiviruses [multiplicity of infection (MOI), 100] and 5 mg/mL of polybrene. MiR-204-5p angomir/NC and miR-204-5p antangomir/NC were synthesized by GenePharma Co. Ltd. (Shanghai, China). They were transfected into the GC cells using Lipofectamine 3000 (Invitrogen, Carlsbad, CA, USA) in accordance with the manufacturer’s instructions.
CCK-8 assays
The cells were seeded in 96-well plates at a density of 2×103 cells/well. At the designated time points (24, 48, and 72 h), 10 µL of Cell Counting Kit-8 (CCK-8; Dojindo, Kumamoto, Japan) solution was added to each well. The absorbance at 450 nm was measured for each sample using a microplate reader (Bio-Rad, Hercules, CA, USA).
Cell-cycle assays
The cells were fixed with 70% cold anhydrous ethanol. The cells were then treated with Propidium iodide (PI) (KeyGen Biotech, Nanjing, China) and Ribonuclease A (RNase A). Cell-cycle distribution was analyzed using a flow cytometer (BD Biosciences, San Diego, CA, USA).
In-vivo tumor formation assays
Lentiviral-mediated stable NORAD-knockdown cells and negative control cells were subcutaneously injected into the flanks of the BALB/c nude mice [Specific Pathogen Free (SPF) grade, 3–4-week-old, male]. Tumor volume was measured every 4 days. The volume of the xenografted tumor was monitored every 3 days using the following formula: volume = (length × width × width)/2. The grouping of the mice was known to the designers of this study, but not to the breeders and operators. This protocol was prepared before the study without registration. Animal experiments were performed under a project license (No. IACUC-AWEC-202011003) granted by the Animal Care & Welfare Committee of The Affiliated Dongguan People’s Hospital, Southern Medical University, in compliance with Chinese National Standard (No. GBT35823-2018) guidelines for the care and use of animals.
Transwell assays
Cells were added to the top chamber with or without Matrigel coating, while the lower chamber was supplemented with culture medium containing 10% FBS only. After 24 h of cell culture, the cells that migrated or invaded the lower chamber were fixed using 4% paraformaldehyde, stained with 0.1% crystal violet solution, and subsequently visualized using an inverted light microscope.
Nucleocytoplasmic fractionation assays
The cytoplasmic and nuclear components were separated using the PARIS kit (Life Technologies, MA, USA). The nuclear and cytoplasmic fractions were detected by quantitative RT-PCR to determine NORAD distribution using GAPDH and U6 as the cytoplasmic and nuclear references, respectively.
Bioinformatics analysis and dual-luciferase reporter gene assays
The binding sites for miR-204-5p within the NORAD–3 prime untranslated region (3'UTR) and KMT2D-3'UTR were obtained from Starbase. Putative wild-type (WT) and mutant (MUT) miR-204-5p binding sites in the 3'-UTR of NORAD or KMT2D mRNA, termed NORAD-WT or NORAD-MUT and KMT2D-WT or KMT2D-MUT, were sub-cloned into the pGL3 basic vector (Promega, Madison, WI, USA). MiR-204-5p angomir or angomir NC was co-transfected with NORAD-WT, NORAD-MUT, KMT2D-WT, or KMT2D-MUT reporter constructs using Lipofectamine™3000 reagent. Luciferase activity was determined using a Multimode Detector Reporter Assay System (Beckman Coulter, Brea, CA, USA).
RIP assays
RNA immunoprecipitation (RIP) assays were performed using a Magna RIP RNA-Binding Protein Immunoprecipitation kit (Millipore, Billerica, MA, USA) in accordance with the manufacturer’s instructions. Anti-Argonaute-2 or anti-immunoglobulin G (IgG) antibodies were also used. The enrichment of NORAD and miR-204-5p was determined using qRT-PCR.
Western blot
Cell lysates were extracted using radioimmunoprecipitation assays (Beyotime, Shanghai, China), fractionated by electrophoresis, and transferred onto a polyvinylidene fluoride membranes (Millipore). The membranes were blocked with 5% non-fat skim milk and incubated with primary and secondary antibodies. The bands on the membranes were visualized using electrochemiluminescence (Pierce, Rockford, IL, USA). The following primary antibodies were used: anti-KMT2D (1:1,000; Abcam, Cambridge, MA, USA), anti-phosphatase and tensin homolog (PTEN; 1:1,000; Cell Signaling Technology, Danvers, CO, USA), anti-PTEN (1:1,000), anti-phosphoinositide 3-kinases (PI3K) (1:1,000; Cell Signaling Technology), anti-protein kinase B (AKT; 1:1,000; Cell Signaling Technology), and anti-phosphorylated AKT (pAKT; 1:1,000; Cell Signaling Technology).
Statistical analysis
All the statistical analyses were performed using SPSS 21.0 (SPSS, Chicago, IL, USA) or GraphPad Prism (GraphPad Prism, Inc., La Jolla, CA, USA). Each experiment was performed based on 3 independent replicates, and the data are presented as the mean ± standard deviation. A Student’s t-test or 1-way analysis of variance was used to compare the means of the 2 or 3 groups, respectively. Statistical significance was set at P<0.05.
Results
NORAD is highly expressed in GC tissues and cell lines
A cohort containing 60 pairs of freshly frozen GC tissues and paired adjacent normal tissues was included in our study. QRT-PCR was performed to determine the expression levels of NORAD. As Figure 1A shows, NORAD was significantly more upregulated in the GC tissues than the matched adjacent normal tissues (P<0.001). We also examined NORAD expression in different cell lines, and found that the expression of NORAD was significantly higher in several GC cell lines (MKN-45, SGC-7901, AGS, BGC-823, and MGC-803) compared to the gastric epithelial cell line (GES-1) (see Figure 1B). Among these cell lines, the MKN-45 and SGC-7901 cells exhibited the highest levels and were used in the subsequent experiments. Collectively, these results indicate that NORAD is highly expressed in GC.
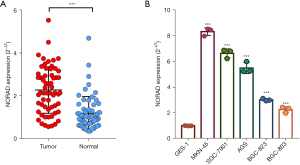
NORAD silencing hampers GC cell growth in vitro and in vivo
To evaluate the biological role of NORAD in the behavior of GC cells, 2 shRNAs specifically targeting NORAD (i.e., shNORAD-1 and shNORAD-2) were designed and constructed. These shRNAs were transfected into the MKN-45 and SGC-7901 cells. The qRT-PCR results showed that shNORAD-1 and shNORAD-2 significantly reduced the expression levels of NORAD in the MKN-45 and SGC-7901 cells (see Figure 2A,2B), indicating satisfactory interfering efficiency for further loss-function experiments.
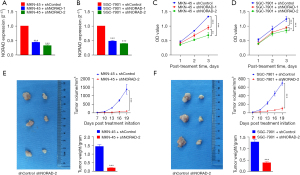
Sustained proliferative signaling is a hallmark of cancer. The roles of NORAD silencing in GC cell growth in GC were investigated first. The CCK-8 results demonstrated that cell proliferation was significantly more suppressed in the shNORAD group than the shControl group (see Figure 2C,2D). We then evaluated the effects of NORAD on GC growth in a mouse model. As Figure 2E,2F show, the sizes and weights of the tumors in the shNORAD group were significantly lower than those in the shControl group 18 days after inoculation. These results suggest that NORAD silencing suppressed GC cell proliferation.
NORAD silencing leads to cell-cycle arrest but does not affect cell migration and invasion in GC
Uncontrolled cell division contributes to the malignant characteristics of cancer cells. To determine whether aberrant NORAD expression disturbs the cell cycle, we analyzed the mitotic cycle of NORAD-silenced GC cells. The results of the flow cytometric analysis showed that the proportions of MKN-45 and SGC-7901 cells increased significantly at the G2/M phase of mitosis after NORAD knockdown (P<0.05, see Figure 3A,3B).
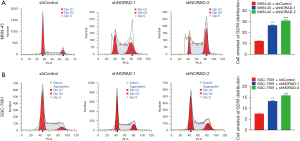
The activation of invasion and metastasis are other malignant characteristics of cancer cells. The invasion and migration abilities of the cells were assessed using Transwell assays. As Figure S1A shows, transfection with shNORAD did not affect GC cell invasion compared to transfection with the shControl. The same trend as that of cell invasion was observed in relation to cell migratory ability (see Figure S1B). These results implied that NORAD silencing arrested the cell cycle but did not alter cell invasion or migration in GC.
NORAD specifically binds to miR-204-5p
Cytoplasmic lncRNAs are often involved in post-transcriptional regulation, and play roles as miRNA sponges. In the present study, we determined the cytoplasmic localization of NORAD in the GC cells (see Figure 4A,4B). As NORAD was mainly co-localized in the cytoplasm, we further analyzed whether it could post-transcriptionally regulate gene expression by acting as an miRNA sponge. First, miR-204-5p was predicted to be a target of NORAD, and putative binding sites were obtained (see Figure 4C). To examine the interaction between NORAD and miR-204-5p, dual-luciferase reporter gene assays were performed using the MKN-45 and SGC-7901 cells. The results showed that the overexpression of miR-204-5p significantly reduced the luciferase activity with the NORAD-WT vector but did not change the luciferase activity with the NORAD-WT vector, indicating an interaction between NORAD and miR-204-5p (see Figure 4D,4E). The RIP assays further confirmed that NORAD could bind to miR-204-5p, with higher levels of NORAD and miR-204-5p in the Ago2 antibody group than in the IgG control group (see Figure 4F,4G). Subsequently, the qRT-PCR results showed that NORAD silencing increased miR-204-5p expression levels (see Figure 4H,4I). MiR-204-5p expression was lower in the GC tissues (see Figure 4J). Further, a Pearson correlation analysis revealed a negative correlation between NORAD and miR-204-5p expression in the GC tissues (see Figure 4K). Overall, our data suggested that NORAD directly binds to miR-204-5p.
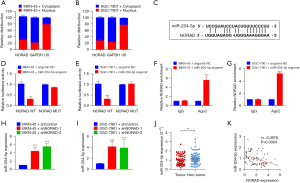
KMT2D is a target gene of miR-204-5p in GC
Based on the bioinformatic prediction, a UTR-binding site was predicted between miR-204-5p and KMT2D (see Figure 5A), and it was hypothesized that KMT2D serves as the target gene of miR-204-5p. Their interactions were confirmed using dual-luciferase reporter gene assays. The results showed that the luciferase activity of the MKN-45 and SGC-7901 cells transfected with the KMT2D WT vector decreased upon the overexpression of miR-204-5p, but no significant change was observed in the MKN-45 and SGC-7901 cells transfected with the KMT2D-MUT vector (see Figure 5B,5C). Moreover, miR-204-5p overexpression significantly reduced KMT2D mRNA and protein expression in the MKN-45 and SGC-7901 cells (see Figure 5D,5E). We also confirmed a negative linear relationship between KMT2D mRNA and miR-204-5p expression in the GC tissues (r=−0.3671, P<0.001; see Figure 5F). Further, qRT-PCR and western blotting revealed that the mRNA and protein levels of KMT2D were suppressed in the MKN-45 and SGC-7901 cells following NORAD silencing (see Figure 5G,5H). Finally, the Pearson’s correlation analysis revealed a positive correlation between NORAD and KMT2D mRNA expression in the same sample cohort (see Figure 5I). Taken together, these results indicate that NORAD positively regulates the miR-204-5p-target gene KMT2D in GC.
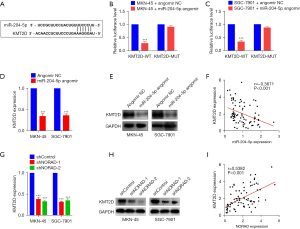
The inhibitory effects of NORAD silencing on GC cell growth are mediated by the miR-204-5p/KMT2D axis
Based on the aforementioned assays, we speculated that the NORAD/miR-204-5p/KMT2D axis plays an important role in GC growth. Thus, rescue assays were performed. As Figure 6A-6D show, the miR-204-5p antangomir reversed the anti-proliferative effects on MKN-45 and SGC-7901 cells induced by NORAD silencing. In addition, NORAD silencing resulted in an increase in the G2/M phase arrest, while the miR-204-5p antagonist rescued this increase. However, such rescue effects were partially abolished by co-transfection with siKMT2D. Based on these results, we showed that the NORAD/miR-204-5p/KMT2D axis may have regulatory effects on GC.
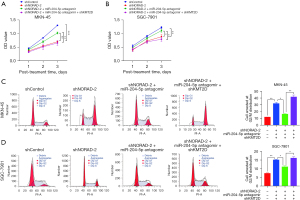
NORAD/miR-204-5p/KMT2D axis regulates the PTEN/PI3K/AKT signaling pathway in GC
There is increasing evidence that the PTEN/PI3K/AKT signaling pathway is a biological mediator of tumor progression. In the present study, we investigated whether NORAD leads to the aberrant activation of the PTEN/PI3K/AKT signaling pathway in GC cells. We measured the protein levels of PTEN/PI3K/AKT pathway factors and found that NORAD silencing significantly increased the expression of PTEN but reduced the expression of PI3K and phosphorylated AKT (pAKT), indicating the potential regulatory effects of NORAD on the PTEN/PI3K/AKT signaling pathway. This regulatory effect was also found to be mediated by the miR-204-5p/KMT2D axis (see Figure 7).
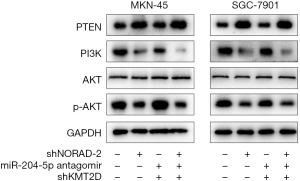
Discussion
GC results from a multi-step process involving the accumulation of numerous genetic and epigenetic alterations in oncogenes and tumor suppressor genes (31,32). LncRNAs were once considered simple cloning artifacts or transcriptional noise (33); however, in recent years, multiple studies have highlighted their potential roles in the pathogenesis of GC (34). The current study showed that NORAD expression is elevated in GC tissues and cell lines and that the silencing of NORAD suppresses GC cell growth via the induction of cell-cycle arrest, which provides evidence of the potential role of this lncRNA in GC progression.
Our study found that NORAD post-transcriptionally regulates the progression of GC through a ceRNA mechanism. The ceRNA network hypothesis states that lncRNAs can absorb miRNAs by binding to them and subsequently function as miRNA sponges, ultimately constructing a framework, under which the miRNA-response element-harboring ncRNAs are systematically functionalized and integrated with the protein-coding RNA element (21,22). As reported previously, long intergenic non-protein coding RNA 00184 (LINC00184) augments the proliferation and invasion of GC cells by targeting the miR-145/ANGPT2 pathway (35). Chen et al. reported that the lncRNA HCP5 inhibits GC progression by functioning as a ceRNA for miR-106b-5p and thus affecting p21 expression (36). Similarly, we found that NORAD interacts with miR-204-5p, a finding which has also been reported in Parkinson’s disease (37).
The present study showed that KMT2D is a downstream target of miR-204-5p. In a previous study, we found that KMT2D is overexpressed in GC tissues (38), and that its depletion suppressed cell proliferation both in vitro and in vivo. In the present study, we found that KMT2D was negatively correlated with miR-204-5p but was positively correlated with NORAD in GC tissues. Based on the luciferase reporter assays, we verified the direct interaction between KMT2D and miR-204-5p in the GC cells. We hypothesized that NORAD sponges miR-204-5p to regulate KMT2D expression in GC cells.
PI3Ks, consisting of the PI3K/AKT signaling pathway, are a family of signaling enzymes that regulate diverse cellular processes, including apoptosis, metabolism, cell proliferation, and cell growth (39). The tumor suppressor PTEN, which is decreased or absent in many tumors, can block the biological processes that inhibit the development of tumors by inactivating the PI3K/AKT signaling pathway (40). The PTEN/PI3K/AKT signaling pathway has been shown to have tumorigenic properties in GC. In our previous study, KMT2D depletion triggered changes in the PTEN/PI3K/AKT pathway (38). In another study, Lv et al. found that KMT2D sustains carcinogenesis by activating the PI3K/AKT pathway (41). In the present study, NORAD, which mediates the expression of KMT2D by targeting miR-204-5p, was confirmed to be a positive regulator of cell proliferation and the cell cycle via the PTEN/PI3K/AKT pathway in GC cells. Most importantly, our rescue experiments further confirmed that NORAD regulates malignant features by targeting the miR-204-5p/KMT2D axis in GC cells. Our findings revealed a novel role for NORAD as a therapeutic target for GC.
Conclusions
In conclusion, our study identified NORAD as a promising oncogene target for GC. NORAD contributes to GC growth by modulating KMT2D expression by targeting miR-204a-5p (see Figure 8). These findings extend understandings of the roles of NORAD and may lead to the development of novel therapeutic strategies for the treatment of GC. However, given that our study was conducted under a retrospective design, further prospective studies with eligibility criteria applicable to clinical trials are needed to confirm our findings that NORAD could accurately predict outcomes for patients with GC.
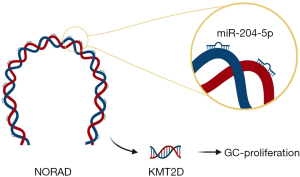
Acknowledgments
Funding: This study was supported by Scientific Research Fund of Dongguan People’s Hospital (No. K201916).
Footnote
Reporting Checklist: The authors have completed the ARRIVE reporting checklist. Available at https://jgo.amegroups.com/article/view/10.21037/jgo-22-1014/rc
Data Sharing Statement: Available at https://jgo.amegroups.com/article/view/10.21037/jgo-22-1014/dss
Conflicts of Interest: All authors have completed the ICMJE uniform disclosure form (available at https://jgo.amegroups.com/article/view/10.21037/jgo-22-1014/coif). The authors have no conflicts of interest to declare.
Ethical Statement: The authors are accountable for all aspects of the work in ensuring that questions related to the accuracy or integrity of any part of the work are appropriately investigated and resolved. This study was approved by the Affiliated Dongguan People’s Hospital, Southern Medical University (Dongguan People's Hospital) (No. KYKT-2020-026) and was conducted in accordance with the Declaration of Helsinki (as revised in 2013). Signed written informed consent forms were obtained from the patients. Animal experiments were performed under a project license (No. IACUC-AWEC-202011003) granted by the Animal Care & Welfare Committee of The Affiliated Dongguan People’s Hospital, Southern Medical University, in compliance with Chinese National Standard (No. GBT35823-2018) guidelines for the care and use of animals.
Open Access Statement: This is an Open Access article distributed in accordance with the Creative Commons Attribution-NonCommercial-NoDerivs 4.0 International License (CC BY-NC-ND 4.0), which permits the non-commercial replication and distribution of the article with the strict proviso that no changes or edits are made and the original work is properly cited (including links to both the formal publication through the relevant DOI and the license). See: https://creativecommons.org/licenses/by-nc-nd/4.0/.
References
- Siegel RL, Miller KD, Fuchs HE, et al. Cancer Statistics, 2021. CA Cancer J Clin 2021;71:7-33. [Crossref] [PubMed]
- Bray F, Ferlay J, Soerjomataram I, et al. Global cancer statistics 2018: GLOBOCAN estimates of incidence and mortality worldwide for 36 cancers in 185 countries. CA Cancer J Clin 2018;68:394-424. [Crossref] [PubMed]
- Cai Q, Zhu C, Yuan Y, et al. Development and validation of a prediction rule for estimating gastric cancer risk in the Chinese high-risk population: a nationwide multicentre study. Gut 2019;68:1576-87. [Crossref] [PubMed]
- Yao K, Uedo N, Kamada T, et al. Guidelines for endoscopic diagnosis of early gastric cancer. Dig Endosc 2020;32:663-98. [Crossref] [PubMed]
- Dai X, Kaushik AC, Zhang J. The Emerging Role of Major Regulatory RNAs in Cancer Control. Front Oncol 2019;9:920. [Crossref] [PubMed]
- Wang T, Liu Y, Zhao M. Mutational analysis of driver genes with tumor suppressive and oncogenic roles in gastric cancer. PeerJ 2017;5:e3585. [Crossref] [PubMed]
- Fabbri M, Girnita L, Varani G, et al. Decrypting noncoding RNA interactions, structures, and functional networks. Genome Res 2019;29:1377-88. [Crossref] [PubMed]
- Chen D, Ping S, Xu Y, et al. Non-Coding RNAs in Gastric Cancer: From Malignant Hallmarks to Clinical Applications. Front Cell Dev Biol 2021;9:732036. [Crossref] [PubMed]
- Yang G, Lu X, Yuan L. LncRNA: a link between RNA and cancer. Biochim Biophys Acta 2014;1839:1097-109. [Crossref] [PubMed]
- Chandra Gupta S, Nandan Tripathi Y. Potential of long non-coding RNAs in cancer patients: From biomarkers to therapeutic targets. Int J Cancer 2017;140:1955-67. [Crossref] [PubMed]
- Yu Y, Chen F, Jin Y, et al. Downregulated NORAD in neuroblastoma promotes cell proliferation via chromosomal instability and predicts poor prognosis. Acta Biochim Pol 2020;67:595-603. [Crossref] [PubMed]
- Li Q, Li C, Chen J, et al. High expression of long noncoding RNA NORAD indicates a poor prognosis and promotes clinical progression and metastasis in bladder cancer. Urol Oncol 2018;36:310.e15-22. [Crossref] [PubMed]
- Zhang XM, Wang J, Liu ZL, et al. LINC00657/miR-26a-5p/CKS2 ceRNA network promotes the growth of esophageal cancer cells via the MDM2/p53/Bcl2/Bax pathway. Biosci Rep 2020;40:BSR20200525. [Crossref] [PubMed]
- Wu X, Lim ZF, Li Z, et al. NORAD Expression Is Associated with Adverse Prognosis in Esophageal Squamous Cell Carcinoma. Oncol Res Treat 2017;40:370-4. [Crossref] [PubMed]
- Wang L, Du L, Duan W, et al. Overexpression of long noncoding RNA NORAD in colorectal cancer associates with tumor progression. Onco Targets Ther 2018;11:6757-66. [Crossref] [PubMed]
- Luo L, Chen C, He H, et al. Silencing of Long Non-Coding RNA (LncRNA) Non-Coding RNA Activated by DNA Damage (NORAD) Inhibits Proliferation, Invasion, Migration, and Promotes Apoptosis of Glioma Cells via Downregulating the Expression of AKR1B1. Med Sci Monit 2020;26:e922659. [Crossref] [PubMed]
- Miao Z, Guo X, Tian L. The long noncoding RNA NORAD promotes the growth of gastric cancer cells by sponging miR-608. Gene 2019;687:116-24. [Crossref] [PubMed]
- Wu Y, Shen QW, Niu YX, et al. LncNORAD interference inhibits tumor growth and lung cancer cell proliferation, invasion and migration by down-regulating CXCR4 to suppress RhoA/ROCK signaling pathway. Eur Rev Med Pharmacol Sci 2020;24:5446-55. [PubMed]
- Lu J, Getz G, Miska EA, et al. MicroRNA expression profiles classify human cancers. Nature 2005;435:834-8. [Crossref] [PubMed]
- Calin GA, Croce CM. MicroRNA signatures in human cancers. Nat Rev Cancer 2006;6:857-66. [Crossref] [PubMed]
- Liz J, Esteller M. lncRNAs and microRNAs with a role in cancer development. Biochim Biophys Acta 2016;1859:169-76. [Crossref] [PubMed]
- Ye J, Li J, Zhao P. Roles of ncRNAs as ceRNAs in Gastric Cancer. Genes (Basel) 2021;12:1036. [Crossref] [PubMed]
- Wang C, Wu D, He M, et al. LncRNA NORAD accelerates the progression of non-small cell lung cancer via targeting miRNA-455/CDK14 axis. Minerva Med 2022;113:817-24. [Crossref] [PubMed]
- Yang Y, Zhang G, Li J, et al. Long noncoding RNA NORAD acts as a ceRNA mediates gemcitabine resistance in bladder cancer by sponging miR-155-5p to regulate WEE1 expression. Pathol Res Pract 2021;228:153676. [Crossref] [PubMed]
- Liu W, Zhou X, Li Y, et al. Long Non-Coding RNA NORAD Inhibits Breast Cancer Cell Proliferation and Metastasis by Regulating miR-155-5p/SOCS1 Axis. J Breast Cancer 2021;24:330-43. [Crossref] [PubMed]
- Sun Y, Wang J, Ma Y, et al. Radiation induces NORAD expression to promote ESCC radiotherapy resistance via EEPD1/ATR/Chk1 signalling and by inhibiting pri-miR-199a1 processing and the exosomal transfer of miR-199a-5p. J Exp Clin Cancer Res 2021;40:306. [Crossref] [PubMed]
- Yin Y, Zhang B, Wang W, et al. miR-204-5p inhibits proliferation and invasion and enhances chemotherapeutic sensitivity of colorectal cancer cells by downregulating RAB22A. Clin Cancer Res 2014;20:6187-99. [Crossref] [PubMed]
- Wang X, Li F, Zhou X. miR-204-5p regulates cell proliferation and metastasis through inhibiting CXCR4 expression in OSCC. Biomed Pharmacother 2016;82:202-7. [Crossref] [PubMed]
- Zhang J, Xing L, Xu H, et al. miR-204-5p Suppress Lymph Node Metastasis via Regulating CXCL12 and CXCR4 in Gastric Cancer. J Cancer 2020;11:3199-206. [Crossref] [PubMed]
- Zeng J, Wei M, Shi R, et al. MiR-204-5p/Six1 feedback loop promotes epithelial-mesenchymal transition in breast cancer. Tumour Biol 2016;37:2729-35. [Crossref] [PubMed]
- Usui G, Matsusaka K, Mano Y, et al. DNA Methylation and Genetic Aberrations in Gastric Cancer. Digestion 2021;102:25-32. [Crossref] [PubMed]
- Grady WM, Yu M, Markowitz SD. Epigenetic Alterations in the Gastrointestinal Tract: Current and Emerging Use for Biomarkers of Cancer. Gastroenterology 2021;160:690-709. [Crossref] [PubMed]
- Cech TR, Steitz JA. The noncoding RNA revolution-trashing old rules to forge new ones. Cell 2014;157:77-94. [Crossref] [PubMed]
- Sun W, Yang Y, Xu C, et al. Roles of long noncoding RNAs in gastric cancer and their clinical applications. J Cancer Res Clin Oncol 2016;142:2231-7. [Crossref] [PubMed]
- Piao HY, Guo S, Jin H, et al. LINC00184 involved in the regulatory network of ANGPT2 via ceRNA mediated miR-145 inhibition in gastric cancer. J Cancer 2021;12:2336-50. [Crossref] [PubMed]
- Chen W, Zhang K, Yang Y, et al. MEF2A-mediated lncRNA HCP5 Inhibits Gastric Cancer Progression via MiR-106b-5p/p21 Axis. Int J Biol Sci 2021;17:623-34. [Crossref] [PubMed]
- Zhou S, Zhang D, Guo J, et al. Long non-coding RNA NORAD functions as a microRNA-204-5p sponge to repress the progression of Parkinson's disease in vitro by increasing the solute carrier family 5 member 3 expression. IUBMB Life 2020;72:2045-55. [Crossref] [PubMed]
- Xiong W, Deng Z, Tang Y, et al. Downregulation of KMT2D suppresses proliferation and induces apoptosis of gastric cancer. Biochem Biophys Res Commun 2018;504:129-36. [Crossref] [PubMed]
- Noorolyai S, Shajari N, Baghbani E, et al. The relation between PI3K/AKT signalling pathway and cancer. Gene 2019;698:120-8. [Crossref] [PubMed]
- Papa A, Pandolfi PP. The PTEN−PI3K Axis in Cancer. Biomolecules 2019;9:153. [Crossref] [PubMed]
- Lv S, Ji L, Chen B, et al. Histone methyltransferase KMT2D sustains prostate carcinogenesis and metastasis via epigenetically activating LIFR and KLF4. Oncogene 2018;37:1354-68. [Crossref] [PubMed]
(English Language Editor: L. Huleatt)