Curcumin inhibits pancreatic cancer cell proliferation by regulating Beclin1 expression and inhibiting the hypoxia-inducible factor-1α-mediated glycolytic pathway
Introduction
While significant progress has been made in recent years for the treatment of pancreatic cancer, its pathogenesis remains to be fully elucidated (1). An in-depth understanding of the characteristics of various cancers will help the development of novel therapeutic targets and strategies, and provide clues for individualized treatment (2-4). Beclin1 was originally identified as a protein that interacts with anti-apoptotic members of the B-cell lymphoma 2 (BCL2) family and acts as an apoptosis inducer and transcriptional repressor. Beclin1 regulates gene transcription and post-transcriptional processing (5,6). Tumor cells adapt to the hypoxic condition by using glycolysis instead of oxidative phosphorylation for adenosine triphosphate (ATP) generation, with the former metabolic pathway being oxygen-independent. Hypoxia inducible factor-1α (HIF-1α) is an important transcription factor that is highly upregulated under hypoxia (7).
Cellular response to hypoxia involves upregulating the expression of key glycolytic enzymes, including hexokinase 2 (HK2), glucose transporter 1 (GLUT1), lactate dehydrogenase A (LDHA), and pyruvate dehydrogenase kinase 1 (PDK1), and regulating the transcription of key enzyme target genes. Curcumin is a chemical component extracted from the rhizome of the Zingiberaceae and Araceae plants. Curcumin is a diketone compound derived from the rare pigment found in these plants (8-10). It has a wide range of antioxidant and anti-inflammatory activities, as well as inhibition of the mitochondrial cascade. Additionally, it has shown significant antitumor activity. Therefore, this current study investigated whether curcumin could inhibit the growth of pancreatic cancer cells by blocking the HIF-1α-mediated glycolytic pathway. We present the following article in accordance with the MDAR reporting checklist (available at https://jgo.amegroups.com/article/view/10.21037/jgo-22-802/rc).
Methods
Cell lines and reagents
Rabbit polyclonal antibodies against GLUT1, PDK1, HK2, HSP70, HSP90, and glyceraldehyde 3-phosphate dehydrogenase (GAPDH) were purchased from Solebo Biotechnology Co., Ltd. The Cell Counting Kit-8 (CCK-8) kit, flow cytometry apoptosis detection kit, and cell cycle analysis kit were purchased from Biyuntian Biotechnology Co., Ltd. Human pancreatic cancer cell lines, PANC-1 and SW1990, were purchased from the Cell Collection Center of Chinese Academy of Sciences and cultured in DMEM containing 10% fetal bovine serum and 100 U/mL penicillin-streptomycin in an incubator containing 5% CO2 at 37 ℃.
Cell proliferation
Cell proliferation was detected using the CCK-8 assay. Cells in the logarithmic growth phase were digested and resuspended in culture medium. Approximately 8×104 cells/mL were seeded in 96-well plates overnight. The cells were treated with different concentrations of curcumin (20, 40, and 60 mM) and 0.1% dimethyl sulfoxide was used as the control. At three time points of 24, 48, and 72 hours, 90 mL of fresh medium was added to the 96-well plate together with 10 µL CCK-8 solution.
Cell apoptosis and cycle analysis
Cells were washed twice with pre-cooled phosphate buffered saline (PBS) and the cell apoptosis assay was performed according to manufacturer’s instructions. Briefly, added 100 µL 1× binding buffer to each tube and then fully suspended the tumor cells. The dye was added and the cells were incubated for 15 minutes at room temperature in the dark, followed by addition of 300 µL 1× binding buffer suspension cells. The cell suspension was then transferred to a 5-mL flow cytometer tube protected from light and flow cytometry was performed within 1 hour.
Immunocytochemical staining analysis
A total of 1×105 cells were seeded in six-well plates and incubated overnight under hypoxic conditions before being treated with different concentrations of curcumin (20, 40, and 60 mM) and 5-FU for 48 hours. PANC-1 and SW1990 cells were blocked with 3% hydrogen peroxide for 15 minutes followed by incubation with primary antibody against Beclin1 (1:1,000) overnight at 4 ℃. The light staining was quantitatively assessed using the Image ProPlu digital Image Analysis System.
Western blot
The total protein was extracted with radio-immunoprecipitation assay (RIPA) lysis buffer. Proteins were separated by SDS-PAGE and transferred to polyvinylidene fluoride membranes. Subsequently, the membranes were blocked with 5% skim milk and incubated with primary antibodies (both diluted 1:1,000) overnight at 4 ℃. The membranes were then incubated with anti-rabbit secondary antibody (diluted 1:5,000) for 2 hours at room temperature. The ECL kit was used to detect the protein bands, and the relative expression of each protein was quantified using the bioradiometric imaging system.
Real-time ATP determination
Cells in the logarithmic growth phase were digested and resuspended into a cell suspension. Approximately 1×104 cells/mL were seeded in 96-well plates overnight. Subsequently, 0.1 mL ATP releasing factor was added to each well, and the reaction was carried out for 5 minutes at room temperature. Another 96-well cell culture plate was taken and 0.1 mL of cytolysis was aspirated from each well of the first plate to the corresponding well of the second plate. The second plate was placed in an automatic porous fluorescence detector and 20 mL ATP reaction solution was added to each well with an automatic sampler. The fluorescence intensity for 10 times was detected immediately. The glycolytic capacity of the cells was analyzed and measured.
Statistical analysis
The SPSS version 19.0 and GraphPad Prism 5.0 software were used to analyze and plot the data. All experiments were repeated three times. Measurement data were expressed as mean ± standard deviation and analyzed using the t test. A P value <0.05 was considered statistically significant.
Results
Curcumin inhibits the proliferation of hypoxic pancreatic cancer cells
The effect of curcumin on the proliferation of pancreatic cancer cells under hypoxic conditions was investigated. PANC-1 and SW1990 cells were treated with different concentrations of curcumin (20, 40, and 60 µM) under hypoxic conditions for 24, 48, and 72 hours. The CCK-8 assay showed that the median maximum inhibitory concentration (IC50) values of curcumin in PANC-1 cells at 24, 48, and 72 hours were 64.68, 39.65, and 29.86 µM, respectively. In SW1990 cells, the IC50 values were 63.00, 37.65, and 29.28 µM, respectively. When the curcumin dose was 0, 20, 40 and 60 µM, the proliferation inhibition rates in PANC-1 cells were 0%, 31.6%, 47.2%, and 63.9%, respectively, and the proliferation inhibition rates in SW1990 cells were 0%, 18.8%, 46.3%, and 63.5%, respectively (Figure 1).
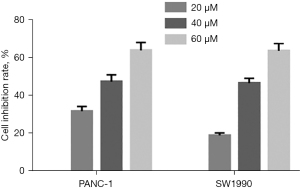
The effects of curcumin on cell clone formation ability, apoptosis, and cell cycle
The clone formation experiments showed that treatment with 30 µM curcumin significantly reduced the number of colonies compared to control cells, and the volume of cell colonies was smaller than that in the control group. Cell cycle analysis by flow cytometry revealed that the number of cells in the G0/G1 phase was significantly increased, while the number of cells in the G2/M phase was decreased after treatment with curcumin. These results suggested that curcumin inhibited pancreatic cell carcinoma cell proliferation in a dose-dependent manner (Figure 2).
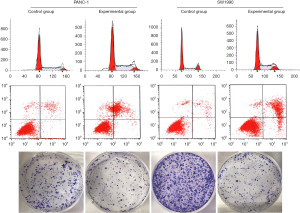
Curcumin inhibits the expression of Beclin1 and its binding to hypoxia-inducible factor-1α in pancreatic cancer cells
Western blot analysis demonstrated that the expression of Beclin1 in curcumin treated cells was significantly decreased. Immunohistochemical staining showed that the expression of Beclin1 in the nucleus and cytoplasm decreased with increasing curcumin concentrations (P<0.05) (Figure 3).
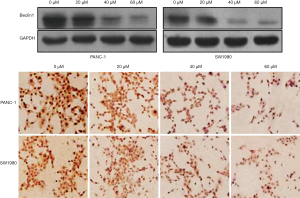
Curcumin inhibits the interaction between Beclin1 and hypoxia-inducible factor-1α
These results suggested that curcumin inhibited the expression of Beclin1, thereby reducing the binding between Beclin1 and HIF-1α in hypoxic pancreatic cancer cells (P<0.05) (Figure 4).
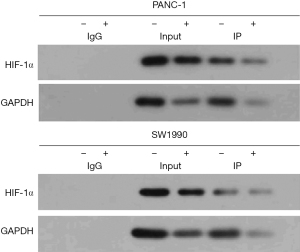
Curcumin promotes the ubiquitination and degradation of hypoxia inducible factor-1α in hypoxic pancreatic cancer cells
To investigate whether curcumin affects the glycolysis of hypoxic pancreatic cancer cells by regulating the ubiquitination of HIF-1α, pancreatic cancer cell lines PANC-1 and SW1990 were treated with different concentrations of curcumin (20, 40, and 60 µM). The protein expression of HIF-1α was significantly decreased after treatment with 40 and 60 µM curcumin. The expression levels of KSP70, KSP90, and von Hippel-Lindau protein (pVHL) were significantly decreased after treatment with different concentrations of curcumin, while the expression levels of PHD and RACK1 were significantly elevated (P<0.05) (Figure 5).
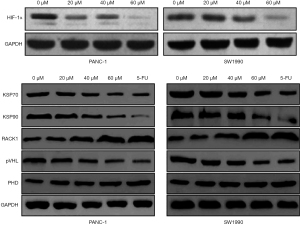
Curcumin inhibits glycolysis in hypoxic pancreatic cancer cells
To determine the effect of curcumin on the glycolysis of pancreatic cancer cells, PANC-1 and SW1990 cells were treated with different doses of curcumin (20, 40, 60 µM) under hypoxia and the rate of ATP production was assessed. As the concentration of curcumin increased, ATP production was reduced in a dose-dependent manner (Figure 6).
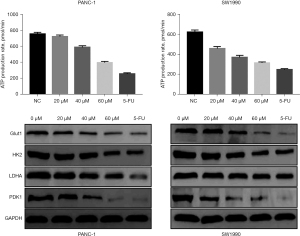
Discussion
The “Warburg effect” refers to the preference of tumor cells for glucose metabolism by glycolysis, even under aerobic conditions (11). A large number of studies have confirmed that the enhanced glycolytic effect is closely related to the occurrence and development of tumors and plays an important role in the malignant progression of tumors (12-14). In this study, curcumin was found to inhibit the proliferation and glycolysis of human pancreatic cancer cells under hypoxic conditions. Similarly, CCK8 assays showed that curcumin inhibited the viability of pancreatic cancer cells under hypoxic conditions, and the cell cycle was arrested in the G0/G1 phase (15). Pancreatic cancer has been shown to be closely related to glycolysis. In this study, gene set enrichment analysis was performed to analyze the gene sets related to glycolysis, which revealed genes related to biological processes. Univariate and multivariate Cox proportional regression models were used to screen genes associated with prognosis. Mrnas significantly associated with overall survival were identified in Cox proportional regression models for pancreatic cancer. Based on this genetic profile, patients were divided into high-risk and low-risk subgroups. In this study, a gene marker associated with glycolysis was developed to predict survival in patients with pancreatic cancer. This finding provides novel insights into the mechanism of glycolysis and is of great application value for the identification of pancreatic cancer patients with poor prognosis.
In vitro and in vivo studies on hepatocellular carcinoma and pancreatic cancer cell lines and nude mouse models of liver orthotopic tumor revealed the inhibitory effect of salinomycin on the growth of liver and pancreas tumors. The relevant results were recently published in the International Journal. The research is considered a breakthrough in the fight against liver cancer and pancreatic cancer (16). Ginsenoside PPD has significant anti-pancreatic cancer effect and is expected to become a new therapeutic drug for pancreatic cancer, other drugs used to treat pancreatic cancer include gemcitabine, 5-fluorouracil, capecitabine, oxaliplatin, paclitaxel and erlotinib (17).
Curcumin can be induced by external death receptor pathway of apoptosis of human pancreatic cancer cells to induce cell cycle arrest inhibition of pancreatic cancer cell proliferation, through a variety of signal transduction pathways of tumor cells to suppress the pancreatic cancer cell proliferation and induce its apoptosis, by reducing the expression of vascular endothelial growth factor to inhibit the angiogenesis of pancreatic cancer cells to form solid tumors, tong Overregulating the expression of tumor cell adhesion molecules can inhibit the motility and invasiveness of pancreatic cancer cells, and can also increase the sensitivity of chemotherapy drugs to cancer cells by regulating the expression of multidrug resistance related genes. The application of curcumin opens up a new way for cancer treatment (18).
Metabolic analysis showed that curcumin significantly reduced the rate of intracellular ATP production under hypoxic conditions (19-22). Incubation with curcumin increased the expression of PHD and a corresponding increase in hydroxylation of HIF-1α was observed in pancreatic cancer cells exposed to hypoxic conditions (23). The ubiquitination of pVHL promoted the degradation of HIF-1α. In addition, the expression of HSP70 was also significantly elevated, thereby promoting the ubiquitination degradation of HIF-1α (24-27). Thus, these results suggested that curcumin inhibits the glycolytic pathway of hypoxic pancreatic cancer cell proliferation by inhibiting the ubiquitination degradation of HIF-1α (28). Beclin1 is highly expressed in pancreatic cancer cells. It has been suggested that Beclin1 can promote the transcription of HIF-1α in hypoxic pancreatic cancer cells through the bZIP domain, thereby increasing the transcription of HIF-1α downstream targeting factors and promoting the proliferation of liver cancer cells. Levels of HIF-1α have been shown to be significantly reduced after Beclin1 gene knockout, suggesting that Beclin1 is an upstream regulator of HIF-1α in hypoxic microenvironments (29). However, the relevant studies are very limited (30).
In conclusion, in hypoxic pancreatic cancer cells, curcumin can inhibit the HIF-1α-mediated glycolytic pathway by down-regulating Beclin1 expression, thereby inhibiting cell proliferation.
Acknowledgments
Funding: None.
Footnote
Reporting Checklist: The authors have completed the MDAR reporting checklist. Available at https://jgo.amegroups.com/article/view/10.21037/jgo-22-802/rc
Data Sharing Statement: Available at https://jgo.amegroups.com/article/view/10.21037/jgo-22-802/dss
Conflicts of Interest: All authors have completed the ICMJE uniform disclosure form (available at https://jgo.amegroups.com/article/view/10.21037/jgo-22-802/coif). The authors have no conflicts of interest to declare.
Ethical Statement: The authors are accountable for all aspects of the work in ensuring that questions related to the accuracy or integrity of any part of the work are appropriately investigated and resolved.
Open Access Statement: This is an Open Access article distributed in accordance with the Creative Commons Attribution-NonCommercial-NoDerivs 4.0 International License (CC BY-NC-ND 4.0), which permits the non-commercial replication and distribution of the article with the strict proviso that no changes or edits are made and the original work is properly cited (including links to both the formal publication through the relevant DOI and the license). See: https://creativecommons.org/licenses/by-nc-nd/4.0/.
References
- Kim HS, Hoang VH, Hong M, et al. Investigation of B,C-ring truncated deguelin derivatives as heat shock protein 90 (HSP90) inhibitors for use as anti-breast cancer agents. Bioorg Med Chem 2019;27:1370-81. [Crossref] [PubMed]
- Qin C, Yang G, Yang J, et al. Metabolism of pancreatic cancer: paving the way to better anticancer strategies. Mol Cancer 2020;19:50. [Crossref] [PubMed]
- Chen Y, Wang J, Zhang X, et al. Correlation between apparent diffusion coefficient and pathological characteristics of patients with invasive breast cancer. Ann Transl Med 2021;9:143. [Crossref] [PubMed]
- Qi A, Li Y, Yan S, et al. Effect of postoperative chemotherapy on blood glucose and lipid metabolism in patients with invasive breast cancer. Gland Surg 2021;10:1470-7. [Crossref] [PubMed]
- Wu L, Zheng Y, Liu J, et al. Comprehensive evaluation of the efficacy and safety of LPV/r drugs in the treatment of SARS and MERS to provide potential treatment options for COVID-19. Aging (Albany NY) 2021;13:10833-52. [Crossref] [PubMed]
- Zeng Z, Zhao Y, Chen Q, et al. Hypoxic exosomal HIF-1α-stabilizing circZNF91 promotes chemoresistance of normoxic pancreatic cancer cells via enhancing glycolysis. Oncogene 2021;40:5505-17. [Crossref] [PubMed]
- Zhang L, Shi H, Chen H, et al. Dedifferentiation process driven by radiotherapy-induced HMGB1/TLR2/YAP/HIF-1α signaling enhances pancreatic cancer stemness. Cell Death Dis 2019;10:724. [Crossref] [PubMed]
- Hesari A, Azizian M, Sheikhi A, et al. Chemopreventive and therapeutic potential of curcumin in esophageal cancer: Current and future status. Int J Cancer 2019;144:1215-26. [Crossref] [PubMed]
- Chen S, Zhang J, Chen J, et al. RER1 enhances carcinogenesis and stemness of pancreatic cancer under hypoxic environment. J Exp Clin Cancer Res 2019;38:15. [Crossref] [PubMed]
- Wu L, Zhong Y, Wu D, et al. Immunomodulatory Factor TIM3 of Cytolytic Active Genes Affected the Survival and Prognosis of Lung Adenocarcinoma Patients by Multi-Omics Analysis. Biomedicines 2022;10:2248. [Crossref] [PubMed]
- Ou ZL, Zhang M, Ji LD, et al. Long noncoding RNA FEZF1-AS1 predicts poor prognosis and modulates pancreatic cancer cell proliferation and invasion through miR-142/HIF-1α and miR-133a/EGFR upon hypoxia/normoxia. J Cell Physiol 2019; Epub ahead of print. [Crossref] [PubMed]
- Nagaraju GP, Zakka KM, Landry JC, et al. Inhibition of HSP90 overcomes resistance to chemotherapy and radiotherapy in pancreatic cancer. Int J Cancer 2019;145:1529-37. [Crossref] [PubMed]
- Wang H, Zhao Z, Lei S, et al. Gambogic acid induces autophagy and combines synergistically with chloroquine to suppress pancreatic cancer by increasing the accumulation of reactive oxygen species. Cancer Cell Int 2019;19:7. [Crossref] [PubMed]
- Kataria N, Martinez CA, Kerr B, et al. C-Terminal HSP90 Inhibitors Block the HIF-1 Hypoxic Response by Degrading HIF-1α through the Oxygen-Dependent Degradation Pathway. Cell Physiol Biochem 2019;53:480-95. [Crossref] [PubMed]
- Feng J, Xie G, Zhan Y, et al. Elevated HSP90 associates with expression of HIF-1α and p-AKT and is predictive of poor prognosis in nasopharyngeal carcinoma. Histopathology 2019;75:202-12. [Crossref] [PubMed]
- Wu L, Zheng Y, Ruan X, et al. Long-chain noncoding ribonucleic acids affect the survival and prognosis of patients with esophageal adenocarcinoma through the autophagy pathway: construction of a prognostic model. Anticancer Drugs 2022;33:e590-e603. [Crossref] [PubMed]
- Jiang PC, Bu SR. Clinical value of circular RNAs and autophagy-related miRNAs in the diagnosis and treatment of pancreatic cancer. Hepatobiliary Pancreat Dis Int 2019;18:511-6. [Crossref] [PubMed]
- Chen L, Yuan R, Wen C, et al. E3 ubiquitin ligase UBR5 promotes pancreatic cancer growth and aerobic glycolysis by downregulating FBP1 via destabilization of C/EBPα. Oncogene 2021;40:262-76. [Crossref] [PubMed]
- Hu Q, Qin Y, Ji S, et al. MTAP Deficiency-Induced Metabolic Reprogramming Creates a Vulnerability to Cotargeting De Novo Purine Synthesis and Glycolysis in Pancreatic Cancer. Cancer Res 2021;81:4964-80. [Crossref] [PubMed]
- Hu L, Xu X, Li Q, et al. Caveolin-1 increases glycolysis in pancreatic cancer cells and triggers cachectic states. FASEB J 2021;35:e21826. [Crossref] [PubMed]
- Zhang D, He Z, Shen Y, et al. MiR-489-3p Reduced Pancreatic Cancer Proliferation and Metastasis By Targeting PKM2 and LDHA Involving Glycolysis. Front Oncol 2021;11:651535. [Crossref] [PubMed]
- Li L, Yu Y, Zhang Z, et al. TRIM47 accelerates aerobic glycolysis and tumor progression through regulating ubiquitination of FBP1 in pancreatic cancer. Pharmacol Res 2021;166:105429. [Crossref] [PubMed]
- Nimmakayala RK, Leon F, Rachagani S, et al. Metabolic programming of distinct cancer stem cells promotes metastasis of pancreatic ductal adenocarcinoma. Oncogene 2021;40:215-31. [Crossref] [PubMed]
- Zhu LL, Wu Z, Li RK, et al. Deciphering the genomic and lncRNA landscapes of aerobic glycolysis identifies potential therapeutic targets in pancreatic cancer. Int J Biol Sci 2021;17:107-18. [Crossref] [PubMed]
- Chen Y, Liu Y, Tao Q, et al. circPUM1 Activates the PI3K/AKT Signaling Pathway by Sponging to Promote the Proliferation, Invasion and Glycolysis of Pancreatic Cancer. Curr Pharm Biotechnol 2022;23:1405-14. [Crossref] [PubMed]
- Wu L, Zhong Y, Yu X, et al. Selective poly adenylation predicts the efficacy of immunotherapy in patients with lung adenocarcinoma by multiple omics research. Anticancer Drugs 2022;33:943-59. [Crossref] [PubMed]
- Wang SJ, Li XD, Wu LP, et al. MicroRNA-202 suppresses glycolysis of pancreatic cancer by targeting hexokinase 2. J Cancer 2021;12:1144-53. [Crossref] [PubMed]
- Nie H, Luo C, Liao K, et al. Seven Glycolysis-Related Genes Predict the Prognosis of Patients With Pancreatic Cancer. Front Cell Dev Biol 2021;9:647106. [Crossref] [PubMed]
- Xu M, Cui R, Ye L, et al. LINC00941 promotes glycolysis in pancreatic cancer by modulating the Hippo pathway. Mol Ther Nucleic Acids 2021;26:280-94. [Crossref] [PubMed]
- Gong S, Xiong L, Luo Z, et al. SIRT6 promotes ferroptosis and attenuates glycolysis in pancreatic cancer through regulation of the NF-κB pathway. Exp Ther Med 2022;24:502. [Crossref] [PubMed]
(English Language Editor: J. Teoh)