Plasminogen activator, urokinase enhances the migration, invasion, and proliferation of colorectal cancer cells by activating the Src/ERK pathway
Highlight box
Key findings
• Plasminogen activator, urokinase (PLAU), or urokinase-type plasminogen activator (uPA) promotes the migration, invasion, and proliferation of CRC cells via Src/ERK pathway.
What is known and what is new?
• Src activation is an epigenetic characteristic of colorectal cancer at the early stage and is related to metastasis character.
• Abnormal activation of the mitogen-activated protein kinase (MAPK)/extracellular-regulated kinase (ERK) pathway contributes to the migration and invasion of CRC.
What is the implication, and what should change now?
• The miRNA regulation for PLAU provides a reference for potential CRC treatment strategies.
Introduction
Colorectal cancer (CRC) is a life-threatening digestive tract malignancy and is the fourth leading cause of cancer-related deaths worldwide (1). The infinite proliferation, infiltration, and metastasis of CRC cells are the major causes of patient deaths. It is reported that metastasis has already occurred in 25% of patients at the time of initial diagnosis, and a similar proportion of patients develop metastasis during the disease progression (2,3). Tumors in the colon wall (stages I and II) can be cured by excision, and approximately 73% of CRC with lymph node metastasis (stage III) can be cured by surgery combined with adjuvant chemotherapy. More recently, patients’ survival rate has been improved by chemotherapy, but tumors with distant metastasis are often incurable (4,5). Therefore, it is critically important to thoroughly explore the molecular mechanisms involved in the occurrence and development of CRC to search for targets for early diagnosis, recurrence, and metastasis.
Plasminogen activator, urokinase (PLAU) encodes a secreted serine protease that converts plasminogen to plasmin. It is also known as urokinase-type plasminogen activator (uPA) and is secreted in many cancer cells, including CRC cells (6). PLAU binds to the uPA receptor (uPAR) (7,8) to promote the proteolytic cascade and convert inactive proteases, such as plasmin and matrix metallopeptidase 9 (MMP-9), into active forms, thereby endowing tumor cells with the ability to degrade extracellular matrix (ECM) components and stimulate tumor cell proliferation and adhesion (7,9). Specifically, by degrading the ECM and basement membranes (for example, laminin, fibronectin, and collagen), PLAU has been shown to facilitate the metastasis and angiogenesis of tumors (8,10). Therefore, PLAU is a key player in the migration, invasion, and metastasis of tumor cells (11,12). As reported, increased PLAU expression corresponds to a poor prognosis, and the American Society of Clinical Oncology (ASCO) has called for PLAU to be used as a possible target for risk assessment and treatment. Src is one of a tyrosine kinase protein mainly acts in signal transduction. It is normally located in the cytoplasm. Once activated, it is transferred to the nucleus to regulate the activity of transcription factors and produce cell effects (13). Src activation is common in the early stage of CRC, and its activation is related to metastasis of CRC (14). Abnormal activation of the mitogen-activated protein kinase (MAPK)/extracellular-regulated kinase (ERK) pathway contributes greatly to the malignant behavior of CRC (15).
However, PLAU has rarely been studied in CRC, especially regarding its expression and mechanism. In this study, the effects of PLAU expression on the migration, invasion, and proliferation of CRC cells were detected, and its possible mechanism of action was preliminarily analyzed using bioinformatics analysis, thereby laying a foundation for the research on potential biological targets of CRC. We present the following article in accordance with the MDAR reporting checklist (available at https://jgo.amegroups.com/article/view/10.21037/jgo-22-1215/rc).
Methods
Bioinformatics analysis
We searched the Gene Expression Omnibus (GEO) database (https://www.ncbi.nlm.nih.gov/gds/) for CRC-related datasets. The datasets GSE156355 and GSE184093 of CRC-related mRNA expressions were identified and downloaded. The study was conducted in accordance with the Declaration of Helsinki (as revised in 2013). The RNA-seq data in GSE156355 were subjected to quantile normalization using the “limma” package of R software [R Core Team (2021)]. Correlation analysis and principal component analysis were performed on the samples, and the differentially expressed genes (DEGs) were analyzed (|logFC| >2.5, P<0.05). Moreover, the “ggplot2” package of R software was used to visualize a volcano plot of the DEGs in GSE156355. The “pheatmap” package of R software was selected for the cluster analysis and to generate a heatmap of the DEGs. Similarly, the RNA-seq data in GSE184093 were subjected to quantile normalization, correlation and principal component analyses were performed on the samples, and the DEGs were analyzed (|logFC| >2.5, P<0.05). The “pheatmap” package of R software was used to generate the relevant visual graphics.
Functional enrichment analysis
The intersection was taken between GSE156355 and GSE184093 using the “RobustRankAggreg” R software package to obtain the common DEGs. The Kyoto Encyclopedia of Genes and Genomes (KEGG) and Gene Ontology (GO) enrichment analyses of DEGs were performed by the “ggplot2” and “GOplot” R software packages (16). The DAVID (Database for Annotation, Visualization and Integrated Discovery) database (https://david.ncifcrf.gov) was used to integrate the GO functional analyses at the biological process (BP), cellular component (CC), and molecular function (MF) levels.
Gene set enrichment analysis (GSEA)
The GSEA tool (http://www.gsea-msigdb.org/) was used to perform the GSEA and plot the pathway enrichment diagram.
Cell culture and grouping
The human CRC cell lines SW480 (#TCHu172) and SW620 (#TCHu101) were purchased from the Chinese National Collection of Authenticated Cell Cultures (Shanghai, China). The SW480 and SW620 cells were cultured in L15 medium (Hyclone, CA, USA) containing 10% fetal bovine serum (FBS; Hyclone) and 1% penicillin-streptomycin (Solarbio, Beijing, China) in a 37 ℃ incubator with 5% CO2. Cells in the logarithmic growth phase were selected and inoculated into a 6-well plate, and the medium was replaced before transfection to ensure optimal condition of the cells. When the cell density reached approximately 70%, the CRC cells were transfected with PLAU-NC, PLAU-mimic, or PLAU-inhibitor (Ribobio, Guangzhou, China) in accordance with the Lipofectamine 2000 kit (Invitrogen, CA, USA) instructions, and divided into a PLAU-NC group, PLAU-mimic group (30 nM), and PLAU-inhibitor group (60 nM). Three replicates for each group were adopted. After 48 h, the cells were collected for later studies.
Detection of cell migration and invasion by Transwell assays
Transwell migration and invasion assays were performed according to the previous publication (17). For the invasion assay, Matrigel (Solarbio) was pre-coated in the upper Transwell chamber, and the SW480 and SW620 cells were inoculated into the upper Transwell chamber (BD, CA, USA) (approximately 1×105/well) with 200 µL of serum-free medium. Six hundred microliters of medium containing 20% FBS was added to the lower chamber. The chamber was removed after incubation at 37 ℃ for 36 h, and the cells were fixed with 4% paraformaldehyde (Solarbio) for 30 min, then stained with 0.1% crystal violet (Solarbio) for 15 min. The cells on the internal surface of the chamber were gently wiped off. Finally, the migrating or invading cells were observed and counted in five randomly fields under an Olympus inverted microscope (Olympus, Tokyo, Japan).
Wound healing assay
The SW480 and SW620 cells were inoculated into a 6-well plate (#3335; Corning, CA, USA) (approximately 7×105/well) and cultured overnight. When the cell density reached 90–100%, uniformly thick scratches were made on the cell surface using a 10 µL pipette tip. The cells were washed three times with PBS (#P1022; Solarbio) to remove the shedding cells and cultured with a serum-free medium. At 0, 12, and 24 h, cells were fixed in 4% paraformaldehyde for 20 minutes and stained with 0.1% crystal violet for 20 minutes. The images of the migration area were captured under an optical microscope to observe the wound healing status.
Monoclonal assay
The cells in each group were inoculated into a 6-well plate (400/well) and dispersed evenly, followed by culture for 7–14 d until the colonies could be observed. The medium was gently removed, and the cultured cells were washed twice with PBS. The colonies were fixed with methanol for 30 min and stained with 0.1% crystal violet for 20 min. Finally, the colonies with more than 50 cells were counted under the microscope.
Western blotting
The western blot was performed according to the previous publications (18,19). Briefly, the total protein of cells was extracted with RIPA (radioimmunoprecipitation assay) lysis buffer (#R0020; Solarbio) in each group, and its concentration was determined by Bicinchoninic Acid Assay (BCA) (#PC0020; Solarbio). Then, 30 µg of protein samples were added to each lane, separated by 10% sodium dodecyl sulfate-polyacrylamide gel electrophoresis, and transferred onto a polyvinylidene fluoride (PVDF) membrane (#IPFL00010; Millipore, MA, USA). The membrane was sealed with 5% skim milk (#232100; BD) at room temperature for 1 h and incubated with a primary antibody solution of PLAU (#ab282403; Abcam biotech, CA, USA), Src (#ab109381; Abcam), p-Src (#2101; Cell signaling), ERK (#EPR17526; Abcam), p-ERK, MMP-2, MMP-3, MMP-9, CyclinD1, CyclinA2 and glyceraldehyde-3-phosphate dehydrogenase (GAPDH) at 4 ℃ overnight. The secondary antibody (Santa Cruz, CA, USA) solution (1:1,000) was added to the membrane and cultured at room temperature for 1 h, washed with phosphate-buffered saline (PBS) three times, and developed and fixed with ECL (enhanced chemiluminescence, enhanced chemiluminescence) reagent (Promega, TA, USA). GAPDH was adopted as the internal reference. The gray value of the band was analyzed using ImageJ software (NIH, USA).
Statistical analysis
The target gene expressions in different diseases in The Cancer Genome Atlas (TCGA) were queried using the GEPIA (Gene Expression Profiling Interactive Analysis) database, and a survival analysis was conducted. SPSS 21.0 software (IBM, IL, USA) was used for the data analysis. Measurement data were expressed as mean ± SD (standard deviation). A one-way analysis of variance (ANOVA) was used to compare multiple groups, and Fisher’s least significant difference (LSD) t-test was used for pairwise comparisons. A P value <0.05 was considered statistically significant.
Results
Screening of DEGs
The DEG analysis in the GSE156355 dataset showed 335 DEGs in the mRNA of these CRC samples. Of these, 127 were upregulated, and 208 were downregulated. Figure 1A shows the volcano plot of the DEGs in GSE156355, and the heatmap of DEGs is presented in Figure 1B. Furthermore, we found 233 DEGs in the mRNA of the GSE184093 dataset, which included 62 upregulated mRNAs and 171 downregulated mRNAs. The volcano plot and heatmap of the differentially expressed mRNAs are displayed in Figure 1C,1D.
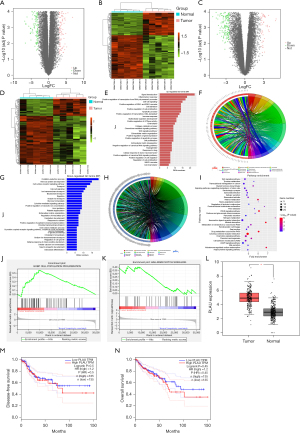
Bioinformatics analysis
The intersection was taken between GSE156355 and GSE184093 using the “RobustRankAggreg” R software package, and the DEGs obtained were analyzed by GO and KEGG enrichment analyses. The GO analysis at the BP (Biological Processes) level identified signal transduction, inflammatory response, and positive regulation of ERK1 and ERK2 cascade pathways (Figure 1E,1F). The downregulated pathways included the oxidation-reduction process, cell surface receptor signaling, and cell-cell signaling (Figure 1G,1H). The KEGG enrichment analysis of DEGs (Figure 1I) indicated that neuroactive ligand-receptor interaction, cyclic adenosine monophosphate (cAMP) signaling, and transcriptional misregulation pathways were enriched in CRC. In addition, GSEA analysis showed that the CELL_POPULATION_PROLIFERATION and NOTCH_SIGNALING pathways were enriched (Figure 1J,1K).
Statistical analysis
PLAU was analyzed using the GEPIA database. The results showed that PLAU was highly expressed in CRC (Figure 1L), and a high expression of PLAU corresponded to a low survival rate. The disease-free survival (DFS) and overall survival (OS) curves are shown in Figure 1M,1N.
PLAU enhanced the migration and invasion of CRC cells
The wound healing assay results (Figure 2A,2B) showed that after 12 and 24 h, the scratch width in the PLAU-mimic group was significantly smaller than in the PLAU-NC group, suggesting that migration was enhanced with increased PLAU. In contrast, the scratch width in the PLAU-inhibitor group was significantly increased compared with the PLAU-NC group. Similarly, Transwell analysis (Figure 3A,3B) showed that the number of cells that migrated and passed through the basement membrane was significantly greater in the PLAU-mimic group than the PLAU-NC group, while it was significantly smaller in the PLAU-inhibitor group than in the PLAU-NC group. Consistent with the above results, the protein expressions of MMP-2, MMP-3, and MMP-9 in SW480 cells increased significantly in the PLAU-mimic group compared with those in the PLAU-NC group (Figure 4A,4B). The above findings demonstrate the ability of PLAU to enhance the migration and invasion of CRC SW480 cell lines.
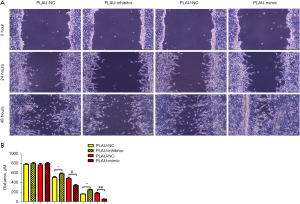
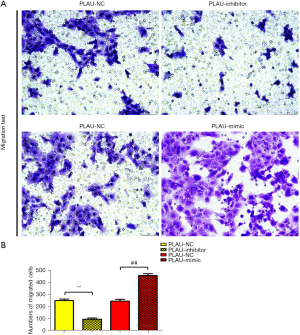
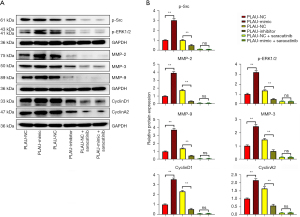
PLAU promoted proliferation of CRC cells
The effect of PLAU on the colony formation of SW480 cells was measured (Figure 5A-5E). Compared with the PLAU-NC group, SW480 cells formed an increased number of colonies after in vitro overexpression of PLAU. In contrast, the number of colonies formed after PLAU was downregulated decreased, indicating that PLAU enhances the proliferation of SW480 cells (Figure 5A-5E). In addition, the proliferation-related proteins Cyclin D1 and Cyclin A2 in the SW480 cells (Figure 4A,4B) were significantly increased in the PLAU-mimic group compared with the PLAU-NC group, indicating that overexpressed PLAU can promote the proliferation of CRC cells.
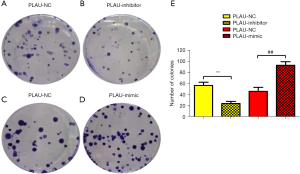
PLAU affected the migration, invasion, and proliferation of CRC cells by activating the Src/ERK pathway
As seen from the above results, PLAU facilitated the migration, invasion, and proliferation of CRC cells. The molecular mechanism of PLAU in enhancing the migration, invasion, and proliferation of CRC cells was investigated after abolishing Src expression combined with the results of the bioinformatics analysis. We noticed the protein expressions of p-Src and p-ERK in SW480 cells were significantly higher in the PLAU-mimic group than in the PLAU-NC group, while the results were the opposite in the PLAU-inhibitor group. After saracatinib was added, the protein levels of p-ERK, MMP-2, MMP-3, MMP-9, Cyclin D1, and Cyclin A2 significantly declined in SW480 and SW620 cells. To sum up, PLAU promotes the migration, invasion, and proliferation of CRC cells by activating the Src/ERK pathway.
Discussion
CRC is primarily treated with surgery but is prone to postoperative metastasis, recurrence, and poor prognosis. Therefore, it is essential to investigate the mechanisms related to CRC to improve its diagnosis, treatment, and prognosis (20,21). Invasion and metastasis are the prominent malignant biological characteristics of tumor cells. They are the major factors causing poor prognosis and the main causes of clinical anti-tumor therapy failure and subsequent patient deaths (14). Malignant tumor invasion and metastasis are multi-factor, multiple-gene complex processes, and inhibiting them is essential to improve patients’ quality of life (22,23). In this study, PLAU was analyzed using the GEPIA database. The results showed that PLAU was highly expressed in CRC tissues compared with normal controls, and the survival curve revealed that a high expression of PLAU corresponded to a low survival rate, suggesting that PLAU has a carcinogenic effect in the progression of CRC. According to previous studies, PLAU contributes to tumor progression by degrading the ECM and related basement membranes (10,11). The effects of PLAU expression on the migration, invasion, and proliferation of CRC cells were detected in our study, and its possible mechanism of action was preliminarily analyzed and combined with a bioinformatics analysis, thereby laying a foundation for the research on potential biological targets of CRC.
The current study found that PLAU enhanced the migration, invasion, and proliferation of CRC cells. At 12 and 24 h after scratches were made on the cell surface using a 10 µL pipette tip, the scratch width decreased in the PLAU-mimic group compared with the PLAU-NC group, while it was significantly larger in the PLAU-inhibitor group than in the PLAU-NC group, indicating that overexpression of PLAU in vitro facilitates the migration of CRC cells. Similarly, the number of cells that migrated and passed through the membrane was significantly greater in the PLAU-mimic group than in the PLAU-NC group but was decreased in the PLAU-inhibitor group. Consistent with the above results, upregulation of PLAU by PLAU-mimic increased MMP-2, MMP-3, and MMP-9 proteins in SW480 and SW620 cells. It is reported that MMPs are crucial regulators in cancer cell migration, growth, and inflammation. MMP-9 is involved in phytic acid-stimulated migration and invasion of CRC cells (24), and the ERK pathway contributes to MMP-9-induced metastasis in various cancers (25). As an important proteolytic enzyme, MMP-9 is expressed in multiple tumors and enhances cell invasion and metastasis by degrading the ECM (26-28). To sum up, PLAU promoted the migration and invasion of CRC SW480 cell lines. In this study, the results of the colony formation assay revealed that SW480 cells formed an increased number of colonies after in vitro overexpression of PLAU, indicating that proliferation of SW480 cells is enhanced by PLAU. Moreover, the changes in the expression of proliferation-related proteins in the SW480 cells were measured. The results showed that the protein expressions of Cyclin D1 and Cyclin A2 in SW480 cells increased significantly after PLAU overexpression. Cyclin A2 was the first cyclin found to be associated with the malignant transformation of cells (29,30), and its high expression in a variety of tumor tissues but little to no expression in normal tissues has been verified (31,32). Cyclin D1 is an important cell cycle gene mainly acting in the G1 phase and is a key regulator of cell cycle progression. Increased Cyclin D1 expression promotes the proliferation and differentiation of cells and closely correlates with the occurrence and development of tumors (33). Overexpression of PLAU can contribute to the proliferation of CRC cells.
The molecular mechanism of PLAU in enhancing the migration, invasion, and proliferation of CRC cells was investigated by decreasing Src protein expression combined with the results of our bioinformatics analysis. After PLAU overexpression, the protein expressions of p-Src and p-ERK increased significantly in SW480 cells. Studies have demonstrated that ERK is involved in tumor proliferation, apoptosis, invasion, and migration and is associated with the MAPK signaling pathway (15,34). P-ERK is an index for the activity of the ERK signaling pathway, and the continuously activated ERK pathway facilitates the transformation of normal cells to tumor phenotypes (35), consistent with the findings in this study. After adding saracatinib, p-ERK, MMP-2, MMP-3, MMP-9, Cyclin D1, and Cyclin A2 proteins reduced significantly in SW480 and SW620 cells. On the other hand, we only studied and verified the relevant mechanisms at the cellular level. Our research has laid a preliminary foundation for the miRNA application of PLAU, which, of course, needs further in vivo research to verify.
Conclusions
In conclusion, our research demonstrates that PLAU plays a key role in regulating the migration, invasion, and proliferation of CRC cells by activating the Src/ERK pathway. Further study is needed to verify the effect of PLAU in vivo.
Acknowledgments
Funding: This work was supported by Hebei Provincial Health Commission Youth Science and Technology Project (No. 20211337)
Footnote
Reporting Checklist: The authors have completed the MDAR reporting checklist. Available at https://jgo.amegroups.com/article/view/10.21037/jgo-22-1215/rc
Data Sharing Statement: Available at https://jgo.amegroups.com/article/view/10.21037/jgo-22-1215/dss
Conflicts of Interest: All authors have completed the ICMJE uniform disclosure form (available at https://jgo.amegroups.com/article/view/10.21037/jgo-22-1215/coif). The authors have no conflicts of interest to declare.
Ethical Statement: The authors are accountable for all aspects of the work in ensuring that questions related to the accuracy or integrity of any part of the work are appropriately investigated and resolved. The study was conducted in accordance with the Declaration of Helsinki (as revised in 2013).
Open Access Statement: This is an Open Access article distributed in accordance with the Creative Commons Attribution-NonCommercial-NoDerivs 4.0 International License (CC BY-NC-ND 4.0), which permits the non-commercial replication and distribution of the article with the strict proviso that no changes or edits are made and the original work is properly cited (including links to both the formal publication through the relevant DOI and the license). See: https://creativecommons.org/licenses/by-nc-nd/4.0/.
References
- Sung H, Ferlay J, Siegel RL, et al. Global Cancer Statistics 2020: GLOBOCAN Estimates of Incidence and Mortality Worldwide for 36 Cancers in 185 Countries. CA Cancer J Clin 2021;71:209-49. [Crossref] [PubMed]
- Ferlay J, Colombet M, Soerjomataram I, et al. Estimating the global cancer incidence and mortality in 2018: GLOBOCAN sources and methods. Int J Cancer 2019;144:1941-53. [Crossref] [PubMed]
- Wang N. Interpretation on the report of Global Cancer Statistics 2018. Journal of Multidisciplinary Cancer Management 2019;5:87-98. (Electronic Version).
- Liu Z, Xu Y, Xu G, et al. Nomogram for predicting overall survival in colorectal cancer with distant metastasis. BMC Gastroenterol 2021;21:103. [Crossref] [PubMed]
- Guo K, Feng Y, Yuan L, et al. Risk factors and predictors of lymph nodes metastasis and distant metastasis in newly diagnosed T1 colorectal cancer. Cancer Med 2020;9:5095-113. [Crossref] [PubMed]
- Leth JM, Ploug M. Targeting the Urokinase-Type Plasminogen Activator Receptor (uPAR) in Human Diseases With a View to Non-invasive Imaging and Therapeutic Intervention. Front Cell Dev Biol 2021;9:732015. [Crossref] [PubMed]
- Brungs D, Chen J, Aghmesheh M, et al. The urokinase plasminogen activation system in gastroesophageal cancer: A systematic review and meta-analysis. Oncotarget 2017;8:23099-109. [Crossref] [PubMed]
- Su SC, Lin CW, Yang WE, et al. The urokinase-type plasminogen activator (uPA) system as a biomarker and therapeutic target in human malignancies. Expert Opin Ther Targets 2016;20:551-66. [Crossref] [PubMed]
- Mahmood N, Mihalcioiu C, Rabbani SA. Multifaceted Role of the Urokinase-Type Plasminogen Activator (uPA) and Its Receptor (uPAR): Diagnostic, Prognostic, and Therapeutic Applications. Front Oncol 2018;8:24. [Crossref] [PubMed]
- Zhai BT, Tian H, Sun J, et al. Urokinase-type plasminogen activator receptor (uPAR) as a therapeutic target in cancer. J Transl Med 2022;20:135. [Crossref] [PubMed]
- Mauro CD, Pesapane A, Formisano L, et al. Urokinase-type plasminogen activator receptor (uPAR) expression enhances invasion and metastasis in RAS mutated tumors. Sci Rep 2017;7:9388. [Crossref] [PubMed]
- Moirangthem A, Bondhopadhyay B, Mukherjee M, et al. Simultaneous knockdown of uPA and MMP9 can reduce breast cancer progression by increasing cell-cell adhesion and modulating EMT genes. Sci Rep 2016;6:21903. [Crossref] [PubMed]
- Ortiz MA, Mikhailova T, Li X, et al. Src family kinases, adaptor proteins and the actin cytoskeleton in epithelial-to-mesenchymal transition. Cell Commun Signal 2021;19:67. [Crossref] [PubMed]
- Jin W. Regulation of Src Family Kinases during Colorectal Cancer Development and Its Clinical Implications. Cancers (Basel) 2020;12:1339. [Crossref] [PubMed]
- Pashirzad M, Khorasanian R, Fard MM, et al. The Therapeutic Potential of MAPK/ERK Inhibitors in the Treatment of Colorectal Cancer. Curr Cancer Drug Targets 2021;21:932-43. [Crossref] [PubMed]
- Zhang Y, He W, Zhang S. Seeking for Correlative Genes and Signaling Pathways With Bone Metastasis From Breast Cancer by Integrated Analysis. Front Oncol 2019;9:138. [Crossref] [PubMed]
- Zhang Y, Huang X, Liu J, et al. New insight into long non-coding RNAs associated with bone metastasis of breast cancer based on an integrated analysis. Cancer Cell Int 2021;21:372. [Crossref] [PubMed]
- Zhang S, Xin H, Li Y, et al. Skimmin, a Coumarin from Hydrangea paniculata, Slows down the Progression of Membranous Glomerulonephritis by Anti-Inflammatory Effects and Inhibiting Immune Complex Deposition. Evid Based Complement Alternat Med 2013;2013:819296. [PubMed]
- Wang W, Sheng L, Chen Y, et al. Total coumarin derivates from Hydrangea paniculata attenuate renal injuries in cationized-BSA induced membranous nephropathy by inhibiting complement activation and interleukin 10-mediated interstitial fibrosis. Phytomedicine 2022;96:153886. [Crossref] [PubMed]
- Cao W, Chen HD, Yu YW, et al. Changing profiles of cancer burden worldwide and in China: a secondary analysis of the global cancer statistics 2020. Chin Med J (Engl) 2021;134:783-91. [Crossref] [PubMed]
- Chen W, Zheng R, Baade PD, et al. Cancer statistics in China, 2015. CA Cancer J Clin 2016;66:115-32. [Crossref] [PubMed]
- Lin M, Zhang Z, Gao M, et al. MicroRNA-193a-3p suppresses the colorectal cancer cell proliferation and progression through downregulating the PLAU expression. Cancer Manag Res 2019;11:5353-63. [Crossref] [PubMed]
- Yu W, Jiang J, Xie L, et al. Mortality Trends in Colorectal Cancer in China During 2000-2015: A Joinpoint Regression and Age-Period-Cohort Analysis. Prev Chronic Dis 2018;15:E156. [Crossref] [PubMed]
- Fridman R, Scott AF, Muller D, et al. The role of cell adhesion and migration in the in vitro invasiveness of mouse adrenal carcinoma cells. Invasion Metastasis 1990;10:208-24. [PubMed]
- Li Y, Zhang M, Dorfman RG, et al. SIRT2 Promotes the Migration and Invasion of Gastric Cancer through RAS/ERK/JNK/MMP-9 Pathway by Increasing PEPCK1-Related Metabolism. Neoplasia 2018;20:745-56. [Crossref] [PubMed]
- Mendes O, Kim HT, Stoica G. Expression of MMP2, MMP9 and MMP3 in breast cancer brain metastasis in a rat model. Clin Exp Metastasis 2005;22:237-46. [Crossref] [PubMed]
- Vermeer PD, Denker J, Estin M, et al. MMP9 modulates tight junction integrity and cell viability in human airway epithelia. Am J Physiol Lung Cell Mol Physiol 2009;296:L751-62. [Crossref] [PubMed]
- Lv Y, Liu W, Ruan Z, et al. Myosin IIA Regulated Tight Junction in Oxygen Glucose-Deprived Brain Endothelial Cells Via Activation of TLR4/PI3K/Akt/JNK1/2/14-3-3ε/NF-κB/MMP9 Signal Transduction Pathway. Cell Mol Neurobiol 2019;39:301-19. [Crossref] [PubMed]
- Yam CH, Fung TK, Poon RY. Cyclin A in cell cycle control and cancer. Cell Mol Life Sci 2002;59:1317-26. [Crossref] [PubMed]
- Thoma OM, Neurath MF, Waldner MJ. Cyclin-Dependent Kinase Inhibitors and Their Therapeutic Potential in Colorectal Cancer Treatment. Front Pharmacol 2021;12:757120. [Crossref] [PubMed]
- Molendini L, Benassi MS, Magagnoli G, et al. Prognostic significance of cyclin expression in human osteosarcoma. Int J Oncol 1998;12:1007-11. [Crossref] [PubMed]
- Mrena J, Wiksten JP, Kokkola A, et al. Prognostic significance of cyclin A in gastric cancer. Int J Cancer 2006;119:1897-901. [Crossref] [PubMed]
- Luangdilok S, Wanchaijiraboon P, Chantranuwatana P, et al. Cyclin D1 expression as a potential prognostic factor in advanced KRAS-mutant non-small cell lung cancer. Transl Lung Cancer Res 2019;8:959-66. [Crossref] [PubMed]
- Asl ER, Amini M, Najafi S, et al. Interplay between MAPK/ERK signaling pathway and MicroRNAs: A crucial mechanism regulating cancer cell metabolism and tumor progression. Life Sci 2021;278:119499. [Crossref] [PubMed]
- Wang Y, Wu N, Pang B, et al. TRIB1 promotes colorectal cancer cell migration and invasion through activation MMP-2 via FAK/Src and ERK pathways. Oncotarget 2017;8:47931-42. [Crossref] [PubMed]
(English Language Editor: D. Fitzgerald)