Exosomal miR-125b-5p derived from cancer-associated fibroblasts promotes the growth, migration, and invasion of pancreatic cancer cells by decreasing adenomatous polyposis coli (APC) expression
Highlight box
Key findings
• In the PDAC tumor environment, exosomes released by CAFs promote PDAC cell growth, invasion, and metastasis. MiR-125b-5p in exosomes may be the key molecule mediating CAF to promote the malignant progression of pancreatic cancer. Exosomal miR-125b-5p inhibition offers an alternate strategy for combating the basic malady of PDAC.
What is known and what is new?
• According to mounting data, CAFs in PDAC have been found to assist tumor development, invasion, and metastasis. However, the CAFs-derived molecular determinants that regulate PDAC molecular mechanisms have not been fully characterized.
• For the first time, we found that miR-125b-5p in CAF-derived exosomes are highly expressed and can promote the malignant progression of pancreatic cancer.
What are the implications, and what should change now?
• In CAF-derived exosomes, miR-125b-5p may be a key molecule in promoting the development of pancreatic cancer. We may achieve pancreatic cancer inhibition by suppressing the expression of miR-125b-5p.
Introduction
The 5-year survival rate of pancreatic ductal adenocarcinoma (PDAC) is about 10%, highlighting its lethality (1). Micrometastasis is the most common type of metastasis and the primary cause of mortality in pancreatic ductal adenocarcinoma (PDAC) (2). Growing evidence has indicated that stroma cells in the tumor microenvironment (TME) play critical roles in controlling the development, invasion, and metastasis of cancer (3,4). The TME’s essential components, cancer-associated fibroblasts (CAFs), interact with cancer cells to promote carcinogenesis and growth (5,6). However, more research on the processes through which CAFs encourage cancer invasion and metastasis is required.
Vesicles, known as an exosome, which has a diameter of 30 to 100 nm and a lipid bilayer membrane structure, are made up of proteins, double-stranded DNA (dsDNA), RNA, and other substances (7). Their primary role in cell communication is the delivery of messenger RNAs (mRNAs) and microRNAs (miRNAs), as well as proteins and nucleic acids that are tailored to certain cell types, to control intracellular signaling cascades (8). MicroRNAs (miRNAs) are a class of single-stranded non-coding RNA molecules of 18−24 nucleotides in length that play a role in regulating gene expression by specifically recognizing and binding to the 3'-terminal untranslated region (UTR) of target gene mRNA through sequence complementation and inhibiting the translation of target gene mRNA (9). Existing studies have confirmed that miRNAs play an important role in cellular processes such as cell proliferation, differentiation, apoptosis and migration, and miRNAs have been found to act as cancer suppressors or promoters in a variety of cancers (10,11). In the available studies (12), miR-125b-5p plays an important role in the development and progression of several tumors. It has been shown that (13) miR-125b-5p can downregulate TPD52 gene expression, thereby affecting the proliferation, migration and invasion of breast cancer cells. Another study showed that miR-125b-5p showed aberrant expression in bladder cancer (14). It has been reported that miR-125b-5p can regulate glycolysis, proliferation and metastasis of pancreatic cancer cells (15). According to several studies, exosomal-derived miRNAs encourage metastases and improve endothelial cell migration (16,17). However, the mechanisms through which exosomes released by stromal cells control changes in miRNA expression, encourage metastases, and improve endothelial cell migration have not yet been elucidated.
The adenomatous polyposis coli (APC) is a tumor suppressor gene, and the functional significance of altered APC is the dysregulation of several physiological processes that control cellular homeostasis, including cell cycle progression, migration, differentiation, and apoptosis (18,19). The expression of the oncogene APC is deficient to varying degrees in the early stages of epithelial-derived malignancies, such as colon (20), gastric (21), and esophageal (22) cancers. Studies on other malignant tumors have shown that rectal (23), pancreatic (24), liver (25), breast (26), endometrial (27), and other cancers have all shown reduced APC gene expression, which is strongly associated with the development, invasion, and metastasis of these malignant tumors. In addition to genetic alterations, i.e., mutations and deletion factors of genes, epigenetic alterations—abnormal methylation of gene promoters—are a key mechanism in the development of malignant tumors due to the deletion of oncogene expression and functional inactivation (28,29). In recent years, with an in-depth understanding of the pathogenesis of pancreatic cancer, it has been recognized that the development of pancreatic cancer is not only related to genetic factors such as gene mutations but is also closely related to epigenetic abnormalities (30). Moreover, it has also been found that the APC gene, as an cancer suppressor gene, is rarely mutated, and the mechanisms causing the loss of function of this gene include loss of heterozygosity and promoter methylation, in which the “silencing” of gene function caused by promoter methylation is the main cause of the down-regulated gene expression (31,32). Currently, APC gene promoter hypermethylation as well as gene transcription and expression deletion have been observed in colorectal (33), esophageal (34), gastric (35), pancreatic (36), hepatocellular (37), breast (38), endometrial (39), and lung (40) cancers.
In this study, we demonstrated that miR-125b-5p-enriched in exosomes, which are released from CAFs, can promote the metastasis and proliferation of pancreatic cancer cells by suppressing the expression of APC, and as a result, promote the metastasis, invasive, and proliferation of pancreatic cancer cells. This finding highlighted an underlying mechanism for the application of CAFs-derived exosomes as well as a new therapeutic approach for pancreatic cancer. We present the following article in accordance with the MDAR and ARRIVE reporting checklists (available at https://jgo.amegroups.com/article/view/10.21037/jgo-23-198/rc).
Methods
Cell lines and pancreatic cancer samples
The normal epithelial cells of the pancreatic ducts HPDE and human pancreatic ductal adenocarcinoma cell lines, AsPC-1, BxPC-3, Capan2, PANC-1, SW1990, Mia-paca2 andHs-766T, were purchased from the American Type Culture Collection (ATCC) (USA). CAFs cells were obtained from primary cultures of pancreatic ductal adenocarcinoma tissues collected from the Department of Hepatobiliary Surgery, Affiliated Hospital of Guizhou Medical University. These cell lines were grown in 10% Fetal Bovine Serum (FBS)-supplemented dulbecco’s modified eagle medium (DMEM) (Gibco, USA). The experimental protocol was established according to the ethical guidelines of the Helsinki Declaration (as revised in 2013) and was approved by the Human Ethics Committee of The Affiliated Hospital of Guizhou Medical University (No. 162). Written informed consent was obtained from the participants or their guardians.
Cell transfection
APC, si-APC, si-NC, miR-125b-5p mimics, or the corresponding negative vectots were obtained from GenePharma (Shanghai, China). Transfection was performed using Lipofectamine 3000 (Invitrogen, Carlsbad, CA, USA) based on the manufacturer’s recommendations.
Exosome isolation from medium and plasma
Exosomes in medium and plasma were isolated from cell by differential centrifugation. After removing cells and other debris by centrifugation at 300 and 3,000 g respectively, the supernatant was centrifuged at 10,000 g for 30 min to remove shedding vesicles and the other vesicles with larger sizes. Finally, the supernatant was centrifuged at 110,000 g for 70 min, and exosomes were collected from the pellet and re-suspended in PBS (all steps were performed at 4 ℃).
Western blot analysis
In this investigation, primary antibodies were employed against Wingless (WNT), a tumor suppressor gene (APC) and glyceraldehyde-3-phosphate dehydrogenase (GAPDH) (Santa Cruz Biotechnology, Santa Cruz, CA, USA). Horseradish peroxidase-conjugated goat anti-mouse antibodies were utilized as secondary antibodies (1:2,000, Jackson ImmunoResearch, PA, USA). The experiment was conducted according to the aforementioned established protocols.
Quantitative real-time PCR (qPCR) analysis
Total RNA was extracted using TRIzol reagent according to the manufacturer’s instructions (Invitrogen, CA, USA). Next, RNA was reverse-transcribed into complementary DNA (cDNA) using a Superscript Reverse Transcriptase Kit supplied by Transgene (France). Real-time PCR was performed using an Applied Biosystems (USA) ABI7300 real-time PCR instrument. The primers used are listed in Table 1.
Table 1
Gene | Forward primer (5'-3') | Reverse primer (5'-3') |
---|---|---|
miR-355-3p | ATGACAGCTGACCACTGAG | ATTTGTTGCCCAGGAAAGTG |
miR-26a-5p | GGGGTTCAAGTAATCCAGGA | TGCGTGTCGTGGAGTC |
miR-125b-5p | CGTCCCTGAGA | CCCTAACTTGTGA |
miR-130a-5p | AACACGCGCTGACTCCTAGT | CAGTGCAGGGTCCGAGGT |
miR-188-5p | CCCTCTCTCACATCCCTTGCAT | ATCCTGCAAACCCTGCATGTG |
miR-142a-3p | GAACCCTGTAGTGTTTCCTACTT | CAGTGCGTGTCGTGGAGT |
miR-196-5p | CCGACGTAGGTAGTTTCATGTT | GTGCAGGGTCCGAGGTATTC |
miR-204-5p | AGATCTGGAAGAAGATGGTGGT | AATTCACAGTTGCCTACAGTA |
miR-345-5p | CTCCAGCTGGGGCTGACCCCTA | CTCAACTGGT GTCGTGGA |
miR-149-5p | GGCTCTGGCTCCGTGTCTT | CAGTGCAGGGTCCGAGGTATT |
APC | AAAATGTCCCTCCGTTCTTATG | CTGAAGTTGAGCGTAATACCAGT |
PCR, polymerase chain reaction; APC, adenomatous polyposis coli.
Subcutaneous tumorigenesis experiment
Fifteen female BALB/c mice (4 weeks old, weighing about 16 g) were obtained and given a week of adaptive feeding. After being suspended in 200 mL of PBS (1×106), stably transfected cells were subcutaneously injected into the mice. Five weeks later, the tumor tissues were aseptically removed, and tumor weights were calculated according to the guidelines of The Affiliated Hospital of Guizhou Medical University Animal Protection Committee. Animal experiments were performed under a project license (No. 2304120) granted by The Affiliated Hospital of Guizhou Medical University Animal Protection Committee, in compliance with the institutional guidelines for the care and use of animals. A protocol was prepared before the study without registration.
Cell proliferation assay
After seeding the tumor cells into 96-well plates, the culture medium was changed to CCK8 (10 µL) after one night. Next, the cells were cultured in CCK8 containing culture medium for 2 h, a 450 nm absorption rate was obtained to calculate the Optical Density (OD) value, and different time points were observed according to the protocol provided by the manuscript provider (24, 48, 72, and 96 h). For colony formation assays, PC cells were inoculated at 1,000 cells/plate into culture dishes. 2 weeks later, clones were fixed, stained with crystal violet, and counted under a microscope. Images were taken at a 400× zoom using a Zeiss lens.
Cell migration, and invasion assays
For Transwell experiments, 800 µL of DMEM medium containing 20% fetal bovine serum and 1×104 resuspended cells were added to the bottom compartment of an 8 µM Transwell. The top chamber was boronized with Matrigel before the invasion test. Cells were preserved with 4% paraformaldehyde for 10 minutes at room temperature, stained with 0.1% crystal violet, and kept for 20 min at room temperature twenty-four hours after cell seeding. Images were taken at a 400× zoom using a Zeiss lens.
Wound healing assays
For the wound healing experiment, all tumor cells were seeded into six-well plates and cultivated until confluent. A wound that had healed after being scratched in a cell monolayer with a sterile pipette tip was imaged after 24 h. Images were taken at a 400× zoom using a Zeiss lens.
Bioinformatics
Prediction of downstream target genes of miRNAs through the online prediction website STARBASE database (https://starbase.sysu.edu.cn/starbase2/). Downstream target genes of miR-125b were retrieved in miRNA-Target intersections. Selection of downstream target genes by scoring and binding sites.
Statistical analysis
Data from repeated experiments are shown as the mean ± standard deviation (SD). GraphPad Prism 5 software (GraphPad, San Diego, CA, USA) was used to compute the outcome. The statistical significance level was established at P<0.05, and a one-way analysis of variance (ANOVA) was employed to identify significant differences between the groups.
Results
CAF enhanced the invasive and metastatic abilities of pancreatic cancer cells
To confirm the biological effects of CAF cells on pancreatic cancer cells, we constructed a CAF- pancreatic cancer cell co-culture model. We discovered through the CCK8 test that when CAF cells were co-cultured with pancreatic cancer cells, the proliferative viability of the pancreatic cancer cells was considerably increased (Figure 1A). Additionally, we also discovered using a Transwell experiment that co-culturing CAF cells with pancreatic cancer cells dramatically enhanced their invasion and migration abilities (Figure 1B). Finally, the plate cloning assay showed that the co-culture of CAF cells with pancreatic cancer cells enhanced the colony formation ability of pancreatic cancer cells (Figure 1C). Therefore, these results indicate that CAF may promote pancreatic cancer cells’ ability to proliferate and spread invasively.
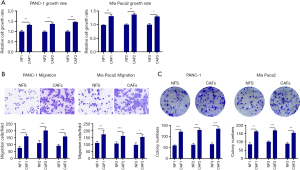
miR-125b-5p was highly expressed in CAF exosomes
To further clarify the key miRNAs in CAF exosomes, we identified the differentially expressed miRNAs by transcriptome sequencing analysis (Figure 2A). Further, we also confirmed and validated the top 10 differentially expressed miRNAs (miR-355-3p, miR-26a-5p, miR-125b-5p, miR-130a-5p, miR-188-5p, miR-142a-3p, miR-196-5p, miR-204-5p, miR-345-5p, miR-149-5p) by PCR experiments. According to the findings, miR-125b-5p was substantially more expressed in CAF exosomes than in the necessary pre-miRNAs (Figure 2B-2D). Based on these results, miR-125b-5p may play a significant role in pancreatic cancer’s CAF-mediated malignant development.
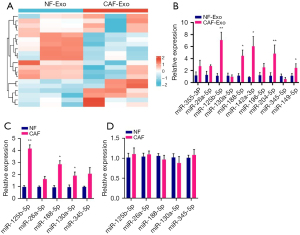
miR-125b-5p promoted pancreatic cancer proliferation, migration, and invasion
Firstly, we used a PCR test to measure the expression levels of miR-125b-5p in pancreatic cancer cell lines, which showed that these cells had a considerably high level of miR-125b-5p expression (Figure 3A). Additionally, we also significantly increased the expression level of miR-125b-5p in pancreatic cancer cells via miRNA mimic transfection (Figure 3B). Furthermore, through the CCK8, Transwell, plate cloning test, and cell scratching assays, we discovered that miR-125b-5p overexpression greatly increased the proliferation and invasive metastasis capacity of pancreatic cancer cells (Figure 3C-3F). These data imply that miR-125b-5p may have a biological role in accelerating the malignant development of pancreatic cancer.
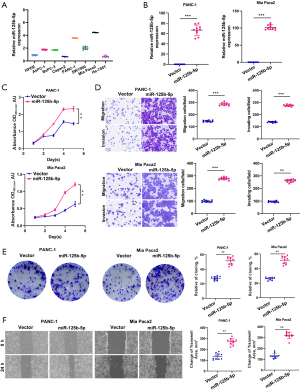
miR-125b-5p directly targets APC
In this investigation, our initial hypothesis was that miR-125b-5p may target APC via informatics. According to the PCR (Figure 4A) and western blotting results (Figure 4B), both the protein and mRNA levels of APC were down-regulated in miR-125b-5p-overexpressing cells. To further confirm the underlying mechanism, a luciferase activity assay was performed. As shown in Figure 4C,4D, wild-type APC reporter gene luciferase activity was reduced when miR-125b-5p was overexpressed in both pancreatic cancer cell lines. In addition, 36 fresh pancreatic cancer tissue samples were used to investigate the underlying relationship between miR-125b-5p and APC. Figure 4E shows that miR-125b-5p was over-expressed while APC was downregulated in pancreatic cancer tissues. Spearman correlation analysis showed that the expression of miR-125b-5p was negatively correlated with the expression of APC (r=−0.44). APC was also downregulated in pancreatic cancer tissues (Figure 4F).
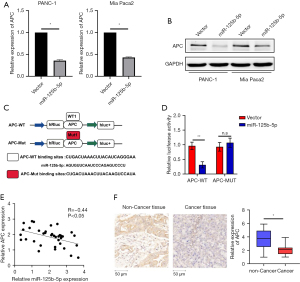
miR-125b-5p-targeted APC promotes pancreatic cancer progression
Further, the western blot assay confirmed that APC was downregulated in pancreatic cancer (Figure 5A-5D). Meanwhile, APC expression in pancreatic cancer cells with APC overexpression was suppressed by miR-125b-5p (Figure 5E,5F). The CCK8 assay demonstrated that the growth ability of pancreatic cancer cells was significantly upregulated in APC-knocked-down cells, and miR-125b-5p could partly reverse this effect in APC-overexpressed pancreatic cancer cells (Figure 5G-5I). The Transwell assay demonstrated that pancreatic cancer cell migration and invasion were significantly upregulated in APC-knocked-down cells, and miR-125b-5p could partly reverse this effect in APC-overexpressed pancreatic cancer cells (Figure 5J-5L). These results indicate that miR-125b-5p-targeted APC promotes pancreatic cancer progression.
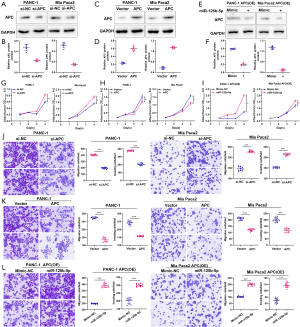
miR-125b-5p inhibited pancreatic cancer cell proliferation in vivo
Figure 6 demonstrates that the PANC-1-miR-125b-5p group produced a greater tumor volume than the control group (Figure 6A,6B).
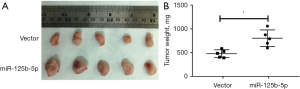
Discussion
The growth and spread of malignant tumors are intimately correlated with CAFs, which are the predominant stromal cell type in the tumor microenvironment (41). CAF can be activated by resting fibroblasts or transformed by epithelial cells, endothelial cells, and mesenchymal stem cells (42). Exosomes function as carriers of miRNAs during the early stages of pancreatic cancer and can distribute miRNAs in an unstable milieu, transforming recipient cells and encouraging the growth of pancreatic cancer cells (43). For instance, in a hypoxic milieu, pancreatic cancer cells produce miR-301a-3p-rich exosomes that polarize macrophages to support the malignant behaviors of pancreatic cancer cells. Pancreatic cancer might perhaps be diagnosed and treated by focusing on exosomal miR-301a-3p (44). According to other research, miR-30b-5p was noticeably more abundant in exosomes produced by hypoxic PDAC cells. These exosomes may be transported to human umbilical vein endothelial cells (HUVECs), where they would upregulate miR-30b-5p. Exosomes produced by hypoxic PDAC cells may facilitate endothelial cell migration and tube formation by downregulating the gap junction protein Gap Junction Protein Alpha 1 (GJA1). Moreover, these exosomes boosted in vivo new microvascular density. Total miR-30b-5p and exosomal miR-30b-5p levels in peripheral blood plasma were greater in PDAC patients than in controls. Furthermore, there were substantial associations between the levels of total miR-30b-5p or exosomal miR-30b-5p in portal vein plasma and peripheral blood plasma (45). In the present study, we discovered that exosomes from CAF substantially elevated the expression of miR-125b-5p. Furthermore, we also discovered that CAF cells may use miR-125b-5p as a critical molecule to accelerate the malignant development of pancreatic cancer.
MiRNAs are crucial for controlling development, growth, and other important biological processes (46). Currently, numerous miRNAs have been investigated as diagnostic biomarkers for diseases, biomarkers for cancer subtypes, and therapeutic targets (47,48). Several disorders are regulated by miR-125b-5p. For instance, miR-125b-5p knockdown decreases Ring1 and YY1 binding protein (RYBP) expression and the biologically malignant activity of gastric cancer cells (49). In ischemia acute kidney damage, miR-125b-5p from bone marrow mesenchymal stem cells enhances tubular healing by concentrating on and suppressing P53 expression in renal tubular epithelial cells (50). In the present study, we discovered that miR-125b-5p expression was increased in pancreatic cancer cells and that miR-125b-5p overexpression significantly inhibited pancreatic cancer cell proliferation, lactate production, glucose consumption, Adenosine Triphosphate (ATP) production, as well as the expression of the Lactate dehydrogenase A (LDHA), Phosphatidylinositol-dependent protein kinase-1 (PDK1), and Hexokinase 2 (HK2) proteins. Wu et al. (51) also showed that miR-125b-5p was upregulated in highly invasive pancreatic cancer cells and promoted migration, invasion, and EMT. We discovered that the expression of miR-125b-5p was markedly elevated in tissues and cells from pancreatic cancer patients. In addition, we also found that miR-125b-5p might considerably boost the capacity of pancreatic cancer cells to proliferate, migrate, and invade. Prediction of downstream target genes of miRNAs through the online prediction website STARBASE database (https://starbase.sysu.edu.cn/starbase2/). Downstream target genes of miR-125b were retrieved in miRNA-Target intersections. Selection of downstream target genes by scoring and binding sites. APC may be its downstream functional target gene, which was supported by dual luciferase and PCR experiments.
Given that malignant carcinogenesis is a multi-stage, multi-factorial, and multi-genetic illness with an unclear specific mechanism, there are currently no optimal anti-tumor medications. The APC-associated Wnt pathway is closely related to tumor development, and the opening and closing of this pathway directly control the expression of downstream target genes, including many oncogenes related to growth and metabolism (52). It is now generally accepted that the APC gene is a typical oncogene, and structural and expression abnormalities have been successively identified in a variety of human tumors. These findings imply that the APC gene may be involved in a variety of human cancer processes. The APC protein expression product plays a crucial regulatory role in cell proliferation, apoptosis, and signaling (53). Since it is an important component of the Wnt signaling pathway, it also has a significant impact on the malignant transformation of cells. It has been found that in the early stages of epithelial-derived malignant tumors, such as colon, gastric, and endometrial cancers, the expression of the APC oncogene is deficient to varying degrees, promoting the development of tumor development and invasive metastasis (21,54,55). Following APC gene inactivation, the inability of the β-catenin protein to be properly broken down results in the accumulation of free β-catenin in the cytoplasm and subsequent entry into the nucleus, which leads to abnormal transcription of target genes downstream of the Wnt signaling pathway, including C-myc, c-jun, and cyclin D1, and ultimately, cancer development (25,56).
Conclusions
In the present study, we found that CAFs encourage PDAC cell growth, epithelial-mesenchymal transition (EMT), and metastasis. Importantly, CAFs release exosomes into PDAC cells, which significantly increase the levels of miR-125b-5p in these cells. Meanwhile, pancreatic cancer cell lines and PDAC tissues have considerably higher miR-125b-5p expression. MiR-125b-5p’s elevated expression mechanically suppresses the expression of APC and accelerates the spread of pancreatic cancer. Exosomes released by CAFs encourage PDAC growth, invasion, and metastasis. Exosomal miR-125b-5p inhibition offers an alternate strategy for combating the basic malady of PDAC.
Acknowledgments
Funding: This work was supported by the Science and Technology Foundation of Guizhou Health Committee (No. gzwjkj2017-1-003).
Footnote
Reporting Checklist: The authors have completed the MDAR and ARRIVE reporting checklists. Available at https://jgo.amegroups.com/article/view/10.21037/jgo-23-198/rc
Data Sharing Statement: Available at https://jgo.amegroups.com/article/view/10.21037/jgo-23-198/dss
Peer Review File: Available at https://jgo.amegroups.com/article/view/10.21037/jgo-23-198/prf
Conflicts of Interest: All authors have completed the ICMJE uniform disclosure form (available at https://jgo.amegroups.com/article/view/10.21037/jgo-23-198/coif). The authors have no conflicts of interest to declare.
Ethical Statement: The authors are accountable for all aspects of the work in ensuring that questions related to the accuracy or integrity of any part of the work are appropriately investigated and resolved. The experimental protocol was established according to the ethical guidelines of the Helsinki Declaration (as revised in 2013) and was approved by the Human Ethics Committee of The Affiliated Hospital of Guizhou Medical University (Approval number 162). Written informed consent was obtained from individual participants or their guardians. Animals experiments were performed under a project license (No. 2304120) granted by The Affiliated Hospital of Guizhou Medical University Animal Protection Committee, in compliance with the institutional guidelines for the care and use of animals.
Open Access Statement: This is an Open Access article distributed in accordance with the Creative Commons Attribution-NonCommercial-NoDerivs 4.0 International License (CC BY-NC-ND 4.0), which permits the non-commercial replication and distribution of the article with the strict proviso that no changes or edits are made and the original work is properly cited (including links to both the formal publication through the relevant DOI and the license). See: https://creativecommons.org/licenses/by-nc-nd/4.0/.
References
- Yang J, Li Y, Han X, et al. The antitumor effect of the novel agent MCL/ACT001 in pancreatic ductal adenocarcinoma. J Cancer Res Clin Oncol 2022; [Crossref] [PubMed]
- Du L, Wang-Gillam A. Trends in Neoadjuvant Approaches in Pancreatic Cancer. J Natl Compr Canc Netw 2017;15:1070-7. [Crossref] [PubMed]
- Bejarano L, Jordao MJC, Joyce JA. Therapeutic Targeting of the Tumor Microenvironment. Cancer Discov 2021;11:933-59. [Crossref] [PubMed]
- Jin MZ, Jin WL. The updated landscape of tumor microenvironment and drug repurposing. Signal Transduct Target Ther 2020;5:166. [Crossref] [PubMed]
- Mao X, Xu J, Wang W, et al. Crosstalk between cancer-associated fibroblasts and immune cells in the tumor microenvironment: new findings and future perspectives. Mol Cancer 2021;20:131. [Crossref] [PubMed]
- Kochetkova M, Samuel MS. Differentiation of the tumor microenvironment: are CAFs the Organizer? Trends Cell Biol 2022;32:285-94. [Crossref] [PubMed]
- Kalluri R, LeBleu VS. The biology, function, and biomedical applications of exosomes. Science 2020;367:eaau6977. [Crossref] [PubMed]
- Sun Z, Shi K, Yang S, et al. Effect of exosomal miRNA on cancer biology and clinical applications. Mol Cancer 2018;17:147. [Crossref] [PubMed]
- Borghaei H, Gettinger S, Vokes EE, et al. Five-Year Outcomes From the Randomized, Phase III Trials CheckMate 017 and 057: Nivolumab Versus Docetaxel in Previously Treated Non-Small-Cell Lung Cancer. J Clin Oncol 2021;39:723-33. [Crossref] [PubMed]
- Huang X, Zhu X, Yu Y, et al. Dissecting miRNA signature in colorectal cancer progression and metastasis. Cancer Lett 2021;501:66-82. [Crossref] [PubMed]
- Wang JK, Wang Z, Li G. MicroRNA-125 in immunity and cancer. Cancer Lett 2019;454:134-45. [Crossref] [PubMed]
- Tang L, Yuan Y, Zhai H, et al. MicroRNA-125b-5p Correlates With Prognosis and Lung Adenocarcinoma Progression. Front Mol Biosci 2021;8:788690. [Crossref] [PubMed]
- Wang Y, Fang J, Gu F. MiR-125b-5p/TPD52 Axis Affects Proliferation, Migration and Invasion of Breast Cancer Cells. Mol Biotechnol 2022;64:1003-12. [Crossref] [PubMed]
- Wen Z, Huang G, Lai Y, et al. Diagnostic panel of serum miR-125b-5p, miR-182-5p, and miR-200c-3p as non-invasive biomarkers for urothelial bladder cancer. Clin Transl Oncol 2022;24:909-18. [Crossref] [PubMed]
- Yu T, Li G, Wang C, et al. MIR210HG regulates glycolysis, cell proliferation, and metastasis of pancreatic cancer cells through miR-125b-5p/HK2/PKM2 axis. RNA Biol 2021;18:2513-30. [Crossref] [PubMed]
- Garcia-Martin R, Wang G, Brandao BB, et al. MicroRNA sequence codes for small extracellular vesicle release and cellular retention. Nature 2022;601:446-51. [Crossref] [PubMed]
- Whiteside TL. Exosome and mesenchymal stem cell cross-talk in the tumor microenvironment. Semin Immunol 2018;35:69-79. [Crossref] [PubMed]
- Goss KH, Groden J. Biology of the adenomatous polyposis coli tumor suppressor. J Clin Oncol 2000;18:1967-79. [Crossref] [PubMed]
- Minde DP, Anvarian Z, Rudiger SG, et al. Messing up disorder: how do missense mutations in the tumor suppressor protein APC lead to cancer? Mol Cancer 2011;10:101. [Crossref] [PubMed]
- Gobert AP, Latour YL, Asim M, et al. Protective Role of Spermidine in Colitis and Colon Carcinogenesis. Gastroenterology 2022;162:813-27 e8. [Crossref] [PubMed]
- Yang XZ, Cheng TT, He QJ, et al. LINC01133 as ceRNA inhibits gastric cancer progression by sponging miR-106a-3p to regulate APC expression and the Wnt/beta-catenin pathway. Mol Cancer 2018;17:126. [Crossref] [PubMed]
- Wang W, Shao F, Yang X, et al. METTL3 promotes tumour development by decreasing APC expression mediated by APC mRNA N(6)-methyladenosine-dependent YTHDF binding. Nat Commun 2021;12:3803. [Crossref] [PubMed]
- Cancer Genome Atlas N. Comprehensive molecular characterization of human colon and rectal cancer. Nature 2012;487:330-7. [Crossref] [PubMed]
- Vietri MT, D'Elia G, Caliendo G, et al. Pancreatic Cancer with Mutation in BRCA1/2, MLH1, and APC Genes: Phenotype Correlation and Detection of a Novel Germline BRCA2 Mutation. Genes (Basel) 2022;13:321. [Crossref] [PubMed]
- Jung YS, Stratton SA, Lee SH, et al. TMEM9-v-ATPase Activates Wnt/beta-Catenin Signaling Via APC Lysosomal Degradation for Liver Regeneration and Tumorigenesis. Hepatology 2021;73:776-94. [Crossref] [PubMed]
- Narayan S, Jaiswal AS, Law BK, et al. Interaction between APC and Fen1 during breast carcinogenesis. DNA Repair (Amst) 2016;41:54-62. [Crossref] [PubMed]
- Kitchen-Goosen SM, Schumacher H, Good J, et al. Endometrial hyperplasia with loss of APC in a novel population of Lyz2-expressing mouse endometrial epithelial cells. Carcinogenesis 2022; Epub ahead of print. [Crossref] [PubMed]
- Yang B, Wang JQ, Tan Y, et al. RNA methylation and cancer treatment. Pharmacol Res 2021;174:105937. [Crossref] [PubMed]
- Papanicolau-Sengos A, Aldape K. DNA Methylation Profiling: An Emerging Paradigm for Cancer Diagnosis. Annu Rev Pathol 2022;17:295-321. [Crossref] [PubMed]
- Li J, Yuan S, Norgard RJ, et al. Epigenetic and Transcriptional Control of the Epidermal Growth Factor Receptor Regulates the Tumor Immune Microenvironment in Pancreatic Cancer. Cancer Discov 2021;11:736-53. [Crossref] [PubMed]
- Liu F, Lu X, Zhou X, et al. APC gene promoter methylation as a potential biomarker for lung cancer diagnosis: A meta-analysis. Thorac Cancer 2021;12:2907-13. [Crossref] [PubMed]
- Ying R, Wei Z, Mei Y, et al. APC gene 3'UTR SNPs and interactions with environmental factors are correlated with risk of colorectal cancer in Chinese Han population. Biosci Rep 2020;40:BSR20192429. [Crossref] [PubMed]
- Chun SK, Fortin BM, Fellows RC, et al. Disruption of the circadian clock drives Apc loss of heterozygosity to accelerate colorectal cancer. Sci Adv 2022;8:eabo2389. [Crossref] [PubMed]
- Kawakami K, Brabender J, Lord RV, et al. Hypermethylated APC DNA in plasma and prognosis of patients with esophageal adenocarcinoma. J Natl Cancer Inst 2000;92:1805-11. [Crossref] [PubMed]
- Tomita H, Yamada Y, Oyama T, et al. Development of gastric tumors in Apc(Min/+) mice by the activation of the beta-catenin/Tcf signaling pathway. Cancer Res 2007;67:4079-87. [Crossref] [PubMed]
- Shamai S, Nabiochtchikov I, Kraus S, et al. CD24 and APC Genetic Polymorphisms in Pancreatic Cancers as Potential Biomarkers for Clinical Outcome. PLoS One 2015;10:e0134469. [Crossref] [PubMed]
- Bala S, Wunsch PH, Ballhausen WG. Childhood hepatocellular adenoma in familial adenomatous polyposis: mutations in adenomatous polyposis coli gene and p53. Gastroenterology 1997;112:919-22. [Crossref] [PubMed]
- Virmani AK, Rathi A, Sathyanarayana UG, et al. Aberrant methylation of the adenomatous polyposis coli (APC) gene promoter 1A in breast and lung carcinomas. Clin Cancer Res 2001;7:1998-2004. [PubMed]
- Tanwar PS, Zhang L, Roberts DJ, et al. Stromal deletion of the APC tumor suppressor in mice triggers development of endometrial cancer. Cancer Res 2011;71:1584-96. [Crossref] [PubMed]
- Poursoltan P, Currey N, Pangon L, et al. Loss of heterozygosity of the Mutated in Colorectal Cancer gene is not associated with promoter methylation in non-small cell lung cancer. Lung Cancer 2012;77:272-6. [Crossref] [PubMed]
- Papait A, Romoli J, Stefani FR, et al. Fight the Cancer, Hit the CAF! Cancers (Basel) 2022;14:3570. [Crossref] [PubMed]
- Mhaidly R, Mechta-Grigoriou F. Role of cancer-associated fibroblast subpopulations in immune infiltration, as a new means of treatment in cancer. Immunol Rev 2021;302:259-72. [Crossref] [PubMed]
- Ariston Gabriel AN, Wang F, Jiao Q, et al. The involvement of exosomes in the diagnosis and treatment of pancreatic cancer. Mol Cancer 2020;19:132. [Crossref] [PubMed]
- Wang X, Luo G, Zhang K, et al. Correction: Hypoxic Tumor-Derived Exosomal miR-301a Mediates M2 Macrophage Polarization via PTEN/PI3Kgamma to Promote Pancreatic Cancer Metastasis. Cancer Res 2020;80:922. [Crossref] [PubMed]
- Chen K, Wang Q, Liu X, et al. Hypoxic pancreatic cancer derived exosomal miR-30b-5p promotes tumor angiogenesis by inhibiting GJA1 expression. Int J Biol Sci 2022;18:1220-37. [Crossref] [PubMed]
- Rupaimoole R, Slack FJ. MicroRNA therapeutics: towards a new era for the management of cancer and other diseases. Nat Rev Drug Discov 2017;16:203-22. [Crossref] [PubMed]
- Lu Q, Wu R, Zhao M, et al. miRNAs as Therapeutic Targets in Inflammatory Disease. Trends Pharmacol Sci 2019;40:853-65. [Crossref] [PubMed]
- Kabekkodu SP, Shukla V, Varghese VK, et al. Clustered miRNAs and their role in biological functions and diseases. Biol Rev Camb Philos Soc 2018;93:1955-86. [Crossref] [PubMed]
- Jin FE, Xie B, Xian HZ, et al. Knockdown of miR-125b-5p inhibits the proliferation and invasion of gastric carcinoma cells by targeting RYBP. Kaohsiung J Med Sci 2021;37:863-71. [Crossref] [PubMed]
- Cao JY, Wang B, Tang TT, et al. Exosomal miR-125b-5p deriving from mesenchymal stem cells promotes tubular repair by suppression of p53 in ischemic acute kidney injury. Theranostics 2021;11:5248-66. [Crossref] [PubMed]
- Wu M, Tan X, Liu P, et al. Role of exosomal microRNA-125b-5p in conferring the metastatic phenotype among pancreatic cancer cells with different potential of metastasis. Life Sci 2020;255:117857. [Crossref] [PubMed]
- Lahde M, Heino S, Hogstrom J, et al. Expression of R-Spondin 1 in Apc(Min/+) Mice Suppresses Growth of Intestinal Adenomas by Altering Wnt and Transforming Growth Factor Beta Signaling. Gastroenterology 2021;160:245-59. [Crossref] [PubMed]
- van Neerven SM, de Groot NE, Nijman LE, et al. Apc-mutant cells act as supercompetitors in intestinal tumour initiation. Nature 2021;594:436-41. [Crossref] [PubMed]
- Wong CC, Xu J, Bian X, et al. In Colorectal Cancer Cells With Mutant KRAS, SLC25A22-Mediated Glutaminolysis Reduces DNA Demethylation to Increase WNT Signaling, Stemness, and Drug Resistance. Gastroenterology 2020;159:2163-80.e6. [Crossref] [PubMed]
- Katoh M, Katoh M. Molecular genetics and targeted therapy of WNT-related human diseases Int J Mol Med 2017;40:587-606. (Review). [Crossref] [PubMed]
- Peng K, Kou L, Yu L, et al. Histone Demethylase JMJD2D Interacts With beta-Catenin to Induce Transcription and Activate Colorectal Cancer Cell Proliferation and Tumor Growth in Mice. Gastroenterology 2019;156:1112-26. [Crossref] [PubMed]
(English Language Editor: A. Kassem)