Stereotactic body radiation therapy for colorectal cancer liver metastases
Introduction
Colorectal cancer is the third most common cancer worldwide and a leading cause of cancer-related death (1). Approximately 25% to 30% of patients with colorectal cancer present with distant metastases and an additional 50% to 60% develop metastatic disease during the course of their disease (2-4). The liver is the most common site of metastases owing to the portal venous drainage of the colon and superior rectum (5); roughly 80% of patients with colorectal liver metastases (CRLM) (6). A multidisciplinary approach is an essential component of the management of CRLM and may incorporate surgical resection, systemic therapy, and/or local ablative therapies.
Hepatic resection is the preferred treatment for resectable CRLM (7), offering a potentially curative option with an increase in overall survival (OS) and potential for long-term disease control (8,9); 5-year OS ranges from 35% to 60% in patients who undergo surgery and 10-year survival is approximately 24% (3,8,9). The reported cure rate following hepatic resection of CRLM is 20% (8). Ablative techniques [e.g., thermal ablation—radiofrequency ablation (RFA) or microwave ablation (MWA)] can be also be considered in the management of CRLM, either alone or in conjunction with resection, if all sites are amenable to treatment (7,10). RFA or MWA may be considered in patients with three or less CRLM lesions, each with a diameter <3 cm and distant from vulnerable structures (e.g., major blood vessels, central biliary tract or gallbladder, just beneath the diaphragm) (11-13). In a phase II randomized trial, the addition of RFA to systemic therapy for patients with <10 CRLM resulted in an improvement in 3-year progression-free survival (PFS: 27.6% vs. 10.6%, P=0.025) (13).
Stereotactic body radiation therapy (SBRT) is another locally ablative therapy that enables the delivery of large radiation doses to a well-defined target, using image-guidance (IGRT), and motion management (14,15). Steep dose gradients are created near the tumor edge, enabling the delivery of a high dose to the target while limiting the dose delivered to the surrounding organs-at-risk (OARs) (14,15). Given the rationale for local therapy for the treatment of CRLM, unresectable CRLM was one of the first sites to be treated with SBRT. SBRT of metastases has reported local control rates of 31% to 90% after 2 years with tumor control correlating to biologically effective dose and motion management (9).
Current evidence has demonstrated that SBRT can achieve good local control (LC) and OS, with an acceptable toxicity profile in patients with CRLM (16-22). The objective of this review is to summarize the existing evidence for the use of SBRT in the management of CRLM, to provide an overview of the clinical indications for SBRT in CRLM, and to provide an overview of radiation planning principles.
Evidence for the use of SBRT in the management of CRLM
Although there are no phase III randomized clinical trials evaluating the use of SBRT in the management of CRLM, several phase I and II prospective studies have demonstrated promising results in terms of OS, LC, and toxicity (Table 1). Median OS following SBRT for CRLM ranges from 16 to 45 months and LC ranges from 50% to 95% at 1 year (Table 1). A meta-analysis of eighteen studies with 656 patients with CRLM reported a pooled 1-year OS estimate of 67.2% [95% confidence interval (CI): 42.1–92.2%], pooled median OS of 31.5 months (range, 15–24.4 months), and pooled 1-year LC estimate of 67% (95% CI: 43.8–90.2%) (22). In a UK prospective registry on the use of metachronous colorectal cancer oligometastases (liver and other sites), the 1- and 2-year OS was of 92% (95% CI: 86.6–95.3%) and 80.3% (95% CI: 71.8–86.5%) (21). The wide range of reported outcomes is attributable to patient heterogeneity, changes in systemic treatment over time including the use of immunotherapy, variable tumor biology, and variations in the radiation dose and fractionation schedules used. An additional consideration when interpreting these results is that most patients enrolled in the SBRT studies listed were not eligible for surgery, often refractory to many lines of systemic therapy, representing a poorer prognosis group at baseline.
Table 1
Study | N† | Dose fractionation (Gy) (BED10) | Lesion size | Median follow-up (months) | Overall survival | Local control (1 year) (%) | Toxicity [%] | |
---|---|---|---|---|---|---|---|---|
Median (months) | 1 year/2 year (%) | |||||||
Scorsetti, 2018 (17) | 29/42 | 75 Gy/3 fr (262.5 Gy) | 1.1–5.4 cm/1.8–134.3 mL | 24 | 29 | 72/65 | 95 | Fatigue [55]; transient hepatic transaminase increase [25]; nausea [12] |
Herfath, 2004 (23) | 18/35 | 14–26 Gy/1 fr (33.6–93.6 Gy) | ≤6 cm/10 (1 to 132) mL | 5.7 | 25 | 72/NR | 71 | No significant toxicity |
Lee, 2009 (24) | 40/68 | 27.7–60 Gy/6 fr (40.44–120 Gy) | 134.8 (6.7–3,090) mL | 10.8 | 17.6 | 63/NR | 71 | Rib fracture [3]; gastritis [2]; nausea [2]; grade 4 thrombocytopenia [1] |
Hoyer, 2006 (25) | 44/64 | 45 Gy/3 fr (112.5 Gy) | 3.5 (1–8.8) cm | 52 | 19.2 | 67/38 | – | Grade 1–2 nausea [34], Grade 1–2 diarrhea [23]; Grade 3 intestinal toxicity [5], liver failure [2], death [1‡] |
Chang, 2011 (16) | 65 | 22–60 Gy/1–6 fr (40.5–180 Gy) | 134.8 (6.7–3,090) mL | 14 | – | 72/38 | 62 | Grade 1–2 GI toxicity [17]; Grade 3+ GI toxicity [3] |
McPartlin, 2017 (21) | 60 | 22.7–62.1/5–6 fr (31.28–126.37 Gy) | 40.8 (0.6–3,089) mL | 28.1 | 16 | 63/26 | 50 | Grade 3 nausea [2] |
Mendéz Romero, 2008 (26) | 14/15 | 30–37.5 Gy/3 fr | 3.2 (0.5–7.2) cm/22.2 (1.1–322) mL | NR | NR | NR/62 | 86 (2 years) | Grade 3 liver toxicity [2] |
Ambrosino, 2009 (27) | 11/27 | 25–60 Gy/3 fr | NR | 13 | NR | NR | 74 | No significant toxicity |
Goodman, 2010 (28) | 6/29 | 18–30 Gy/1 fr | 2.7 (0.9–10.2) cm/29.7 (3.4–149.8) mL | 17 | 28.6 | NR/50 | 77 | Grade 2 late toxicity (n=4) |
†, number of patients with CRLM/total number of patients included in the study; ‡, patient with many comorbidities and poor underlying liver function. CRLM, colorectal liver metastases; GI, gastrointestinal; SBRT, stereotactic body radiation therapy; BED, biologically equivalent dose; NR, not reported.
Radiation dose/response relationship
The biologically equivalent dose (BED) has been reported to be an important prognostic factor for LC of CRLM treated with SBRT, and improved LC has been observed with higher prescribed radiation doses (17,20,22). In a retrospective review of 65 patients with CRLM metastases, a BED10 >75 Gy was an independent prognostic factor for LC and a BED10 >117 Gy was required to achieve a 1-year LC of 90% (16). McPartlin et al. reported improved LC for patients receiving BED10 ≥75 Gy; the LC reported at 1/2/4 years respectively was: 65%/49%/49% for BED10 ≥75 Gy vs. 44%/23%/14% for patients treated with lower doses (21). In another retrospective analysis of 70 patients, the 2-year LC rates were: 52% for BED10 ≤80 Gy; 83% for BED10 100–112 Gy; and 89% for BED10 ≥132 Gy (29). Although delivery of a high BED appears to be associated with favourable outcomes in terms of LC, it may not always be achievable due to tumor factors (number of lesions, size of lesions, tumor location and proximity of target lesion to OARs such as stomach or small bowel), patient factors (volume of normal liver, motion of liver and OARs, e.g., due to breathing) and treatment factors (radiation technique, dose, motion management, image matching).
Role of biomarkers
The accumulation of in genomic mutations and deregulation of multiple signalling pathways play a major role in the development and progression of colorectal cancer (30-34). Assessment of biomarker status is an important component of personalized management as they have predictive and prognostic potential (7,9,32). KRAS and TP53, for example, are predictive of LC; compared with KRAS wild-type tumors, those with KRAS mutations have inferior LC at 1 year (43% vs. 72%; P=0.02) (33). LC is further decreased in tumors with both KRAS and TP53 mutations (1-year LC 20% vs. 69%; P=0.001) (33). Jethwa et al. similarly reported an increased risk of local failure in tumors with a TP53 mutations (HR =3.1; 95% CI: 0.9–10.6; P=0.06) an even higher in those with both TP53 and KRAS mutations (HR =4.5; 95% CI: 1.1–18.7; P=0.04) (34). Conversely, a prospective multicenter cohort study of colorectal cancer oligometastases at various sites (liver, node, lung, bone) found no difference in local control between KRAS wild-type and mutant cases (log rank P=0.63) although there was an improvement in PFS (HR =0.42, 95% CI: 0.2–0.87; P=0.02) (35). Although not yet validated in prospective studies, when planning SBRT, the molecular genomic subtype of the tumor should be incorporated into decision-making. Where possible, consideration should be given to dose escalation for patients with either TP53 mutations, KRAS mutations, or both.
Toxicity
Liver SBRT is generally well tolerated; the most common treatment related toxicities include fatigue, mild nausea, and transient elevation of liver enzymes (Table 1). The risk of significant Grade 3+ liver toxicity [including radiation-induced liver disease (RILD)] is less than 10%, with most studies reporting no incidence of Grade 3+ liver toxicity (Table 1). Gastrointestinal toxicity has been reported and is generally secondary to the proximity of luminal structures to the target lesion in the liver. In a phase I study of individualized SBRT for liver metastases, 11% of patients treated developed Grade 3 intestinal toxicity within 6 months following SBRT (24). Hoyer et al. reported two duodenal ulcerations (conservative management), one colonic perforation (surgically managed), and one treatment-related death due to hepatic failure (patient has significant pre-existing comorbidities) (25). These early clinical trials have helped to consolidate dose/volume/toxicity risk constraints, and adherence to liver and luminal gastrointestinal radiation dose constraints is needed to keep the risk of serious toxicity low. Notably, this may necessitate a reduction in the prescribed dose to CRLM targets adjacent to the stomach or small bowels and resultantly, may lead to reduced LC for metastases at those sites, especially for KRAS/TP53 mutant CRLM. Other OARs to be considered include kidney, heart, lung, and central biliary tract (36). In particular, there are data to suggest that the risk of biliary toxicity increases with increasing doses per fraction of radiation to the central biliary tract (37,38).
Radiation treatment planning and technical considerations
Patient selection
Multidisciplinary case conference discussion is encouraged, where possible, for patients with CRLM. Although a mandatory discussion is not expected for every case undergoing standard management as per the NCCN guidelines, it should be strongly considered for complex cases where the role of local therapy is not established or where there are multiple therapeutic options based on the aforementioned guidelines (7).
SBRT for CRLM may be considered for patients with oligometastatic colorectal cancer in combination with surgery or other locally ablative therapies. It may also be considered in patients who are not candidates for surgical resection (e.g., due to disease location/extent, medical comorbidities, or patient preferences) or after failure of resection or other ablative therapies (7,9). Patients should have an Eastern Cooperative Oncology Group (ECOG) status ≤2 and expected survival greater than 3 months, and no or treatable extrahepatic disease (39). Ideally, there should be at least 700 cc of uninvolved liver [e.g., liver minus gross tumor volume (GTV)]. Finally, there should be no chemotherapy delivered within two weeks of SBRT (39). Although there are some preclinical and phase I trials investigating the combination of immunotherapy and SBRT, the optimal timing and dose/fractionation schedules of these combinations remain unknown (40-42); for patients on any systemic therapy, including immunotherapy, multidisciplinary discussion should take place to determine sequencing and if wash out periods are recommended. Lastly, biomarkers (KRAS, TP53) may be useful in patient selection for treatment and dose selection during SBRT planning.
Treatment planning principles
There are several important considerations when proceeding with SBRT for CRLM including: patient positioning and CT/MRI simulation, treatment planning, and motion management strategies. Patients are generally positioned supine with their arms above their head. Where possible and resources permit, both a CT and MRI simulation are obtained for treatment planning purposes and include a 4D CT simulation with assessment of motion.
Dose fractionation can be individualized taking into account the strong dose response relationship for CRLM (43). Technical considerations for dose selection include location within the liver, proximity to OARs, motion management technique, and type of on treatment image-guidance used. Single fraction or three fraction SBRT may be considered for lesions at least 1 cm away from the central biliary tract and away from luminal OARs. For lesions where the planning target volume (PTV) is in contact with or overlaps with the central biliary tract or a luminal OAR, 5 fraction SBRT is preferred.
SBRT may additionally be used to treat multiple liver metastases simultaneously if technically possible and if clinically appropriate. It is important to ensure adequate hepatic reserve (>700 cc of unaffected liver) and that OARs can be adequately spared (43). Another option is for multi-modality treatment, treating lesions well suited for thermal ablation with thermal ablation and those better suited for SBRT with SBRT.
In terms of contouring, MRI may aid in target definition due to its superior soft tissue contrast resolution compared with CT. An MRI simulation or alternatively a diagnostic MRI may be fused to the planning CT. The GTV is typically defined using all available imaging and review with a dedicated upper abdominal radiologist may be helpful. The GTV is subsequently expanded to account for microscopic disease, generating a clinical target volume (CTV); a margin of 0 mm is generally appropriate. Consideration may be given for a larger margin in areas of uncertainty, or for CRLM that may have responded to previous systemic therapy (44).
When defining OARs, where possible, standardized protocols and naming conventions should be adopted (45). OAR constraints that are currently used at the Princess Margaret Cancer Centre for 3 and 5 fraction SBRT for liver metastases are provided in Table 2. Additional constraints are summarized by Mohamad et al. (18). For lesions in close proximity to or overlapping with OARs, the balance between adequate LC and potential for cure with potential acute and late toxicity must be carefully considered.
Table 2
OAR (dose-limiting toxicity) | 3 fraction constraints (2,400 to 4,500 cGy) | 5 fraction constraints (2,750 to 5,000 cGy) |
---|---|---|
Liver‡ (radiation-induced liver disease) | Mean <1,300 cGy | Mean for specific prescription§: |
5,000 cGy: <1,300 cGy | ||
4,500 and 4,000 cGy: <1,500 cGy | ||
3,500 cGy: <1,550 cGy | ||
3,000 cGy: <1,600 cGy | ||
2,750 cGy: <1,700 cGy | ||
Bowel (chronic ulcer, fistula, perforation) | Duodenum: D0.5cc <2,220 cGy | Duodenum: D0.5cc <3,500 cGy |
Small bowel: D0.5cc <2,400 cGy | Small bowel: D0.5cc <3,500 cGy | |
Large bowel: D0.5cc <2,520 cGy | Large bowel: D0.5cc <3,500 cGy | |
Stomach (chronic ulcer, fistula, perforation) | D0.5cc <2,220 cGy | D0.5cc <3,500 cGy |
Esophagus (stenosis, fistula, perforation) | D0.5cc <2,400 cGy | D0.5cc <3,500 cGy |
Kidney (renal dysfunction) | Bilateral D200cc <1,500 cGy# | Bilateral mean: <1,000 cGy¶ |
Rib (chronic pain, fracture) | No hot spot > prescription dose | No hot spot > prescription dose |
Spinal cord (myelopathy) | D0cc <1,800 cGy | D0cc <2,500 cGy |
Heart (pericarditis) | D0.5cc <2,400 cGy | D0cc <3,800 cGy; D15cc <3,000 cGy |
Skin (chronic ulceration) | D0cc <135% prescription dose | No hot spot > prescription dose |
PTV | D95 >100% | D95 >100% prescription dose |
D0cc <130% prescription dose |
†, these are current 2023 constraints and may change in the future as new dose/response and toxicity data become available. ‡, calculated as “liver minus gross tumor volume”. §, these are the primary ideal constraints at Princess Margaret; depending on the clinical situation higher doses may be acceptable. ¶, if one of the kidneys has a mean dose >1,000 cGy, the remaining kidney V10Gy <10%; if the patient only has one kidney, V10Gy <10%. #, if the total bilateral kidney volume is <200 cc, then the bilateral kidney Dmean <600 cGy. If there is a solitary kidney then the Dmean <600 cGy. SBRT, stereotactic body radiation therapy; OAR, organ-at-risk; PTV, planning target volume.
The planning target volume (PTV) is generated as an expansion of the CTV and accounts for internal organ motion and daily set up variation. There are a number of considerations for determining the appropriate PTV margin, including motion management and image guidance. The amplitude of breathing motion may be reduced by using abdominal compression (46), breath hold techniques (23), or medications such as lorazepam (47). The use of on treatment image guidance also has the potential to substantially decrease PTV margins by accounting for daily changes in the relative position of the liver, variation in shape and position of neighbouring organs, and breathing motion (48). Finally, the use of new technologies, such as the MRI-guided Radiation Therapy (MRgRT) may also allow for planning target volume reduction by improving target visualization and facilitating adaptive RT; this is discussed in another article in this special series. Finally, in terms of PTV coverage, a minimum of 95% coverage is recommended and a hot spot in the GTV of up to 130% is acceptable and can be used to create steep dose gradients.
Additional treatment considerations include, for patients where the stomach is irradiated, to prescribe pre-medication with anti-emetics prior to radiation therapy (e.g., ondansetron 8 mg, 30–60 min prior to radiation therapy). We routinely prescribe a proton pump inhibitor as well if the stomach or duodenum is irradiated. Finally, alternate day dosing may also be considered if there is concern about luminal structure, central liver toxicity, or other OAR doses.
Reirradiation
There is a paucity of high-level data on re-irradiation of CRLM including limited data on dose constraints for normal liver tolerance. A retrospective review of 49 patients [with either recurrent hepatocellular carcinoma or recurrent liver metastases (52% CRLM)] reported on the safety of re-irradiation with only 2 patients (4.1%) developing RILD (49); in their study the normal liver parenchyma received <15 Gy and the luminal structures did not exceed tolerance from the combined courses (49). In another study evaluating cumulative dose and toxicity following SBRT in the abdomen or pelvis reported no Grade 3+ toxicity when the cumulative bowel dose was BED3 90–98 Gy (50). Important considerations when “adding” two courses of radiation include liver deformation and organ motion, both of which add uncertainty to the tolerance calculations (48).
For patients who have previously had Yttrium-90 ablation, the delivered dose should be taken into account when planning further SBRT with a particular focus on total dose absorbed by the liver (51).
Clinical example
A clinical example of CRLM treatment is demonstrated in Figure 1. A 76-year-old patient completed curative intent treatment for a T3N1M0 moderately differentiated colon adenocarcinoma in September 2019. In April 2021, a new liver lesion was noted on follow-up CT and biopsy was consistent with adenocarcinoma of colonic origin. Given the patient’s comorbidities surgical resection was deemed too high risk. The patient additionally expressed a preference for a non-invasive treatment option and resultantly, multidisciplinary consensus was to proceed with SBRT. Figure 1A demonstrates the gross tumor volume on the radiation planning CT (venous phase). Figure 1B shows the isodose distribution—the lesion was treated to a dose of 50 Gy in 5 fractions (BED10 100 Gy). The treatment was delivered using an active breathing control device for motion management. On his most recent follow-up CT, treatment-related changes were evident in the liver and there were no new nor recurrent lesions.
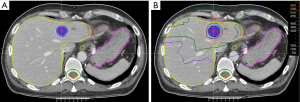
Future directions
There are a number of ongoing clinical trials evaluating the role of SBRT in the management of CRLM. These include evaluation of different techniques, such as proton beam therapy and MR-guided radiation therapy, which aim to increase the therapeutic ratio. Additional studies are also exploring SBRT in the context of immunotherapy and chemotherapy. In terms of treatment planning, more data are required to better define the tolerance of some OARs, such as the central biliary tree as well as the tolerance of the liver and OARs in the setting of re-irradiation. An exciting research opportunity is to exploit the unique immune microenvironment of the liver, that appears to reduce LC of liver metastases versus non-liver metastases, treated with immunotherapy. The most appropriate dosing, sequencing, and combination of SBRT with immunotherapy remains to be determined to overcome this apparent resistance to immunotherapy for liver metastases.
Conclusions
SBRT is an effective non-invasive local treatment for patients with CRLM, providing high rates of local tumor control without significant toxicity, in well selected patients. Patients presenting with CRLM should undergo multidisciplinary discussion, where possible, to obtain a consensus regarding the optimal treatment plan.
Acknowledgments
Funding: None.
Footnote
Provenance and Peer Review: This article was commissioned by the Guest Editors (Falk Roeder and Thomas Brunner) for the series “Precision Radiation Oncology in GI Cancers” published in Journal of Gastrointestinal Oncology. The article has undergone external peer review.
Peer Review File: Available at https://jgo.amegroups.com/article/view/10.21037/jgo-22-1183/prf
Conflicts of Interest: Both authors have completed the ICMJE uniform disclosure form (available at https://jgo.amegroups.com/article/view/10.21037/jgo-22-1183/coif). The series “Precision Radiation Oncology in GI Cancers” was commissioned by the editorial office without any funding or sponsorship. L.A.D. serves as an unpaid editorial board member of Journal of Gastrointestinal Oncology from January 2021 to December 2024. Besides, she is the past president of the American Society for Therapeutic Radiation Oncology and the current University of Toronto Department of Radiation Oncology Chair. The authors have no other conflicts of interest to declare.
Ethical Statement: The authors are accountable for all aspects of the work in ensuring that questions related to the accuracy or integrity of any part of the work are appropriately investigated and resolved.
Open Access Statement: This is an Open Access article distributed in accordance with the Creative Commons Attribution-NonCommercial-NoDerivs 4.0 International License (CC BY-NC-ND 4.0), which permits the non-commercial replication and distribution of the article with the strict proviso that no changes or edits are made and the original work is properly cited (including links to both the formal publication through the relevant DOI and the license). See: https://creativecommons.org/licenses/by-nc-nd/4.0/.
References
- Bray F, Ferlay J, Soerjomataram I, et al. Global cancer statistics 2018: GLOBOCAN estimates of incidence and mortality worldwide for 36 cancers in 185 countries. CA Cancer J Clin 2018;68:394-424. [Crossref] [PubMed]
- Manfredi S, Lepage C, Hatem C, et al. Epidemiology and management of liver metastases from colorectal cancer. Ann Surg 2006;244:254-9. [Crossref] [PubMed]
- Hackl C, Neumann P, Gerken M, et al. Treatment of colorectal liver metastases in Germany: a ten-year population-based analysis of 5772 cases of primary colorectal adenocarcinoma. BMC Cancer 2014;14:810. [Crossref] [PubMed]
- Khatri VP, Petrelli NJ, Belghiti J. Extending the frontiers of surgical therapy for hepatic colorectal metastases: is there a limit? J Clin Oncol 2005;23:8490-9. [Crossref] [PubMed]
- Riihimäki M, Hemminki A, Sundquist J, et al. Patterns of metastasis in colon and rectal cancer. Sci Rep 2016;6:29765. [Crossref] [PubMed]
- Donadon M, Ribero D, Morris-Stiff G, et al. New paradigm in the management of liver-only metastases from colorectal cancer. Gastrointest Cancer Res 2007;1:20-7. [PubMed]
- Benson AB, Venook AP, Al-Hawary MM, et al. Colon Cancer, Version 2.2021, NCCN Clinical Practice Guidelines in Oncology. J Natl Compr Canc Netw 2021;19:329-59. [Crossref] [PubMed]
- Creasy JM, Sadot E, Koerkamp BG, et al. Actual 10-year survival after hepatic resection of colorectal liver metastases: what factors preclude cure? Surgery 2018;163:1238-44. [Crossref] [PubMed]
- Cervantes A, Adam R, Roselló S, et al. Metastatic colorectal cancer: ESMO Clinical Practice Guideline for diagnosis, treatment and follow-up. Ann Oncol 2023;34:10-32. [Crossref] [PubMed]
- Gillams A, Goldberg N, Ahmed M, et al. Thermal ablation of colorectal liver metastases: a position paper by an international panel of ablation experts, The Interventional Oncology Sans Frontières meeting 2013. Eur Radiol 2015;25:3438-54. [Crossref] [PubMed]
- Wang X, Sofocleous CT, Erinjeri JP, et al. Margin size is an independent predictor of local tumor progression after ablation of colon cancer liver metastases. Cardiovasc Intervent Radiol 2013;36:166-75. [Crossref] [PubMed]
- Shady W, Petre EN, Do KG, et al. Percutaneous Microwave versus Radiofrequency Ablation of Colorectal Liver Metastases: Ablation with Clear Margins (A0) Provides the Best Local Tumor Control. J Vasc Interv Radiol 2018;29:268-275.e1. [Crossref] [PubMed]
- Ruers T, Punt C, Van Coevorden F, et al. Radiofrequency ablation combined with systemic treatment versus systemic treatment alone in patients with non-resectable colorectal liver metastases: a randomized EORTC Intergroup phase II study (EORTC 40004). Ann Oncol 2012;23:2619-26. [Crossref] [PubMed]
- Timmerman RD, Herman J, Cho LC. Emergence of stereotactic body radiation therapy and its impact on current and future clinical practice. J Clin Oncol 2014;32:2847-54. [Crossref] [PubMed]
- Kavanagh BD, Miften M, Rabinovitch RA. Advances in treatment techniques: stereotactic body radiation therapy and the spread of hypofractionation. Cancer J 2011;17:177-81. [Crossref] [PubMed]
- Chang DT, Swaminath A, Kozak M, et al. Stereotactic body radiotherapy for colorectal liver metastases: a pooled analysis. Cancer 2011;117:4060-9. [Crossref] [PubMed]
- Scorsetti M, Comito T, Clerici E, et al. Phase II trial on SBRT for unresectable liver metastases: long-term outcome and prognostic factors of survival after 5 years of follow-up. Radiat Oncol 2018;13:234. [Crossref] [PubMed]
- Mohamad I, Barry A, Dawson L, et al. Stereotactic body radiation therapy for colorectal liver metastases. Int J Hyperthermia 2022;39:611-9. [Crossref] [PubMed]
- Chalkidou A, Macmillan T, Grzeda MT, et al. Stereotactic ablative body radiotherapy in patients with oligometastatic cancers: a prospective, registry-based, single-arm, observational, evaluation study. Lancet Oncol 2021;22:98-106. [Crossref] [PubMed]
- Scorsetti M, Comito T, Tozzi A, et al. Final results of a phase II trial for stereotactic body radiation therapy for patients with inoperable liver metastases from colorectal cancer. J Cancer Res Clin Oncol 2015;141:543-53. [Crossref] [PubMed]
- McPartlin A, Swaminath A, Wang R, et al. Long-Term Outcomes of Phase 1 and 2 Studies of SBRT for Hepatic Colorectal Metastases. Int J Radiat Oncol Biol Phys 2017;99:388-95. [Crossref] [PubMed]
- Petrelli F, Comito T, Barni S, et al. Stereotactic body radiotherapy for colorectal cancer liver metastases: A systematic review. Radiother Oncol 2018;129:427-34. [Crossref] [PubMed]
- Herfarth KK, Debus J, Lohr F, et al. Extracranial stereotactic radiation therapy: set-up accuracy of patients treated for liver metastases. Int J Radiat Oncol Biol Phys 2000;46:329-35. [Crossref] [PubMed]
- Lee MT, Kim JJ, Dinniwell R, et al. Phase I study of individualized stereotactic body radiotherapy of liver metastases. J Clin Oncol 2009;27:1585-91. [Crossref] [PubMed]
- Hoyer M, Roed H, Traberg Hansen A, et al. Phase II study on stereotactic body radiotherapy of colorectal metastases. Acta Oncol 2006;45:823-30. [Crossref] [PubMed]
- Méndez Romero A, Wunderink W, van Os RM, et al. Quality of life after stereotactic body radiation therapy for primary and metastatic liver tumors. Int J Radiat Oncol Biol Phys 2008;70:1447-52. [Crossref] [PubMed]
- Ambrosino G, Polistina F, Costantin G, et al. Image-guided robotic stereotactic radiosurgery for unresectable liver metastases: preliminary results. Anticancer Res 2009;29:3381-4. [PubMed]
- Goodman KA, Wiegner EA, Maturen KE, et al. Dose-escalation study of single-fraction stereotactic body radiotherapy for liver malignancies. Int J Radiat Oncol Biol Phys 2010;78:486-93. [Crossref] [PubMed]
- Joo JH, Park JH, Kim JC, et al. Local Control Outcomes Using Stereotactic Body Radiation Therapy for Liver Metastases From Colorectal Cancer. Int J Radiat Oncol Biol Phys 2017;99:876-83. [Crossref] [PubMed]
- Calistri D, Rengucci C, Seymour I, et al. Mutation analysis of TP53, K-ras, and BRAF genes in colorectal cancer progression. J Cell Physiol 2005;204:484-8. [Crossref] [PubMed]
- Zhang J, Zheng J, Yang Y, et al. Molecular spectrum of KRAS, NRAS, BRAF and PIK3CA mutations in Chinese colorectal cancer patients: analysis of 1,110 cases. Sci Rep 2015;5:18678. [Crossref] [PubMed]
- Bokemeyer C, Van Cutsem E, Rougier P, et al. Addition of cetuximab to chemotherapy as first-line treatment for KRAS wild-type metastatic colorectal cancer: pooled analysis of the CRYSTAL and OPUS randomised clinical trials. Eur J Cancer 2012;48:1466-75. [Crossref] [PubMed]
- Hong TS, Wo JY, Borger DR, et al. Phase II Study of Proton-Based Stereotactic Body Radiation Therapy for Liver Metastases: Importance of Tumor Genotype. J Natl Cancer Inst 2017; [Crossref] [PubMed]
- Jethwa KR, Jang S, Mullikin TC, et al. Association of tumor genomic factors and efficacy for metastasis-directed stereotactic body radiotherapy for oligometastatic colorectal cancer. Radiother Oncol 2020;146:29-36. [Crossref] [PubMed]
- O'Cathail SM, Smith T, Owens R, et al. Superior outcomes of nodal metastases compared to visceral sites in oligometastatic colorectal cancer treated with stereotactic ablative radiotherapy. Radiother Oncol 2020;151:280-6. [Crossref] [PubMed]
- Dawson LA, Winter KA, Katz AW, et al. NRG Oncology/RTOG 0438: A Phase 1 Trial of Highly Conformal Radiation Therapy for Liver Metastases. Pract Radiat Oncol 2019;9:e386-93. [Crossref] [PubMed]
- Osmundson EC, Wu Y, Luxton G, et al. Predictors of toxicity associated with stereotactic body radiation therapy to the central hepatobiliary tract. Int J Radiat Oncol Biol Phys 2015;91:986-94. [Crossref] [PubMed]
- Toesca DA, Osmundson EC, Eyben RV, et al. Central liver toxicity after SBRT: An expanded analysis and predictive nomogram. Radiother Oncol 2017;122:130-6. [Crossref] [PubMed]
- Palma DA, Olson R, Harrow S, et al. Stereotactic Ablative Radiotherapy for the Comprehensive Treatment of Oligometastatic Cancers: Long-Term Results of the SABR-COMET Phase II Randomized Trial. J Clin Oncol 2020;38:2830-8. [Crossref] [PubMed]
- Twyman-Saint Victor C, Rech AJ, Maity A, et al. Radiation and dual checkpoint blockade activate non-redundant immune mechanisms in cancer. Nature 2015;520:373-7. [Crossref] [PubMed]
- Schoenhals JE, Seyedin SN, Tang C, et al. Preclinical Rationale and Clinical Considerations for Radiotherapy Plus Immunotherapy: Going Beyond Local Control. Cancer J 2016;22:130-7. [Crossref] [PubMed]
- Monjazeb AM, Giobbie-Hurder A, Lako A, et al. A Randomized Trial of Combined PD-L1 and CTLA-4 Inhibition with Targeted Low-Dose or Hypofractionated Radiation for Patients with Metastatic Colorectal Cancer. Clin Cancer Res 2021;27:2470-80. [Crossref] [PubMed]
- Dawson LA, Eccles C, Craig T. Individualized image guided iso-NTCP based liver cancer SBRT. Acta Oncol 2006;45:856-64. [Crossref] [PubMed]
- Olsen CC, Welsh J, Kavanagh BD, et al. Microscopic and macroscopic tumor and parenchymal effects of liver stereotactic body radiotherapy. Int J Radiat Oncol Biol Phys 2009;73:1414-24. [Crossref] [PubMed]
- Lukovic J, Henke L, Gani C, et al. MRI-Based Upper Abdominal Organs-at-Risk Atlas for Radiation Oncology. Int J Radiat Oncol Biol Phys 2020;106:743-53. [Crossref] [PubMed]
- Dawson LA, Brock KK, Kazanjian S, et al. The reproducibility of organ position using active breathing control (ABC) during liver radiotherapy. Int J Radiat Oncol Biol Phys 2001;51:1410-21. [Crossref] [PubMed]
- Tsang DS, Voncken FE, Tse RV, et al. A randomized controlled trial of lorazepam to reduce liver motion in patients receiving upper abdominal radiation therapy. Int J Radiat Oncol Biol Phys 2013;87:881-7. [Crossref] [PubMed]
- Brock KK. Imaging and image-guided radiation therapy in liver cancer. Semin Radiat Oncol 2011;21:247-55. [Crossref] [PubMed]
- McDuff SGR, Remillard KA, Zheng H, et al. Liver reirradiation for patients with hepatocellular carcinoma and liver metastasis. Pract Radiat Oncol 2018;8:414-21. [Crossref] [PubMed]
- Abusaris H, Hoogeman M, Nuyttens JJ. Re-irradiation: outcome, cumulative dose and toxicity in patients retreated with stereotactic radiotherapy in the abdominal or pelvic region. Technol Cancer Res Treat 2012;11:591-7. [Crossref] [PubMed]
- Mee SF, Polan DF, Dewaraja YK, et al. Stereotactic body radiation therapy (SBRT) following Yttrium-90 (90Y) selective internal radiation therapy (SIRT): a feasibility planning study using90Y delivered dose. Phys Med Biol 2023;68:065003. [Crossref] [PubMed]