CMTM4 inhibits gastric tumorigenesis and metastasis
Highlight box
Key findings
• The protein chemokine like factor (CKLF)-like MARVEL transmembrane domain-containing 4 (CMTM4) inhibits the proliferation, migration, and invasion of gastric cancer (GC) cells.
• The tumor-suppressive function of CMTM4 in GC cells is related to the signal transducer and activator of transcription 1 (STAT1) signaling pathway.
What is known and what is new?
• CMTM4 is an immune regulatory molecule that plays a role in a variety of tumors including hepatocellular carcinoma, colon cancer, and clear cell renal cell carcinoma.
• We showed that CMTM4 inhibits the proliferation, migration, and invasion of GC cells and the STAT1 signaling pathway is involved in the tumor-suppressive function of CMTM4 in GC cells.
What is the implication, and what should change now?
• As a membrane protein, the pathway through which CMTM4 affects the expression and phosphorylation of STAT1 deserves further study.
Introduction
Gastric cancer (GC) is the fifth most commonly diagnosed malignancy worldwide with over 1 million new cases estimated annually (1). With an estimated 478,508 new cases in 2020, the incidence of GC in China is very high (2). Due to late-stage diagnosis, GC has a high mortality rate, with an estimated 768,793 deaths worldwide in 2020 (1). Thus, stomach cancer is one of the most threatening malignancies affecting the health of the population in China (3). Due to distant metastasis and resistance to chemotherapy drugs, the 5-year survival rate of GC is only 40% (4).
GC has multiple etiologies and possible carcinogenic mechanisms, including Helicobacter pylori (H. pylori) infection, a high-salt diet, and genetics (5,6). The lack of biomarkers for early diagnosis makes it difficult to detect GC at an early stage, which severely affects the treatment outcomes and leads to a poor prognosis (7). Therefore, understanding the molecular mechanisms of GC and the identification of novel genes functionally involved in GC progression are crucial for the diagnosis and treatment of GC.
The CKLF-like MARVEL transmembrane domain-containing family (CMTM) is known as the chemokine-like factor superfamily, whose coding products are structurally similar to those of chemokines (8). The family comprises nine members in humans, including CKLF and CMTM1-8, which contain a MARVEL domain, and play important roles in immune regulation and tumorigenesis (9-11). Several studies have shown that members of the CMTM family (CMTM3–5) can prevent the growth and invasion of different types of cancer cells (12-14). We have previously shown that CMTM3 downregulates epidermal growth factor receptor expression and suppresses epidermal growth factor-mediated tumorigenicity in GC cells (15). More recently, we reported that CMTM3 stabilizes nuclear factor kappa-B (NF-κB) essential modulator (NEMO) and protects gastric epithelial cells from apoptosis during H. pylori infection (16).
Interaction between programmed death 1 (PD-1) and programmed cell death-ligand 1 (PD-L1) resulting in T cell dysfunction is one of the most notable immunotherapeutic targets in GC (17). Among the CMTM family, CMTM4 is highly conserved and, along with CMTM6, acts as a regulatory molecule of PD-L1. Specifically, CMTM6 acts to stabilize PD-L1 by impeding its ubiquitin-mediated proteasomal degradation, while CMTM4 assumes a compensatory role in the absence of CMTM6, a function not shared by other CMTM family members (18,19). Recent studies have revealed that CMTM4 is required for the activation of the interleukin (IL)-17A signaling pathway (20,21). Noteworthy, CMTM4 functions as a subunit of IL-17R, which assembles with IL-17RC and IL-17RA to form the IL17 receptor complex (22). CMTM4 was shown to be significantly downregulated in renal clear cell carcinoma and to inhibit cell proliferation, inducing G2/M arrest (23), while its heightened expression correlates with a favorable prognosis in hepatocellular carcinoma (24,25). We previously reported that CMTM4 inhibits the migration of colorectal cancer cells by regulating the AKT/STAT3 pathway, exerting a tumor-suppressive effect (13). However, the function and mechanism of CMTM4 in GC have not been thoroughly elucidated.
Two recent investigations aimed to bridge this knowledge gap. Liang et al. (26) systematically examined the expression profiles of nine genes belonging to the CMTM family in GC tissues, assessing their diagnostic utility across various databases. Their findings revealed a notable downregulation of CMTM4 expression in GC tissues. Similarly, Zhou et al. (27) employed a multifaceted Cox regression model to analyze the transcriptomic data from 118 normal gastric tissues and 827 GC tissues. Their results indicated that elevated levels of CMTM4 expression serve as a protective factor for GC patients. Collectively, these findings underscore the significance of CMTM4 in the context of GC.
In this study, we explored the role and mechanism of CMTM4 in GC cells. These findings offer new perspectives on the mechanistic role of CMTM4 in GC, which could have substantial clinical implications for the development of GC treatment strategies. We present this article in accordance with the MDAR reporting checklist (available at https://jgo.amegroups.com/article/view/10.21037/jgo-24-466/rc).
Methods
Bioinformatics
The effect of CMTM4 expression on the survival of GC patients was analyzed using the Kaplan-Meier Plotter (https://kmplot.com/analysis/index.php?p=service&cancer=gastric).
Tissues and immunohistochemistry
Human gastric mucosal tissues were obtained from the Department of Gastroenterology, Peking University Third Hospital. Gastric mucosa was collected from patients with gastric adenocarcinoma or chronic superficial gastritis. The demographic profiles of patients were listed in Table S1. CMTM4 immunohistochemistry was performed as previously described (28). Five fields were randomly selected for analysis using ImageJ (Silver Spring, MD, USA), and the intensity of the positive regions was compared. The study was conducted in accordance with the Declaration of Helsinki (as revised in 2013). This study was approved by the Ethics Committee of Peking University Third Hospital (No. S2022179) and informed consent was taken from all the patients.
Cell lines and reagents
Human GC cells AGS (ATCC CRL-1739) and human embryonic kidney 293T cells (ATCC: CRL-3216) were purchased from ATCC (Manassas, VA, USA). Human GC cells SGC7901 was preserved in our laboratory. All the cells were authenticated via short tandem repeat (STR) genotyping by Biowing Viotechnology (Shanghai, China). The AGS and SGC7901 cells were cultured with Roswell Park Memorial Institute Medium (RPMI) 1640 (Gibco, Carlsbad, CA, USA) containing 10% fetal bovine serum (FBS, HyClone, Logan, UT, USA). The 293T cells were cultured with Dulbecco’s Modified Eagle Medium (Gibco) containing 10% FBS (HyClone). All the cells were cultured at 37 °C with 5% carbon dioxide. The STAT1 inhibitor fludarabine was purchased from Selleckchem (Houston, TX, USA). All the cell lines were characterized by Biowing Applied Biotechnology (Shanghai, China).
Generation of CMTM4 overexpression and knockdown cells
The CMTM4 overexpression and knockdown plasmids were constructed by Hanbio (Shanghai, China). The primers used to construct the CMTM4 overexpression vector were: h-CMTM4-F: 5'-TACTAGAGGATCTATTTCCGGTGAATTCGCCACCATGCGGAGCGGCGAG-3'; h-CMTM4-R, and 5'-TCACTTAAGCTTGGTACCGAGGATCCGGCAAGTTGCCAGTGATTCAAAC-3'. The target sequence was digested with EcoRI and BamHI, and then ligated with linearized pHBLV lentiviral vector. The ligated product was transformed into the competent cells DH5α, and the plasmid (HBLV-CMTM4OE) was extracted and identified by DNA sequencing. The HBLV-CMTM4OE plasmid, pSPAX2, and pMD2G were co-transfected into the 293T cells for lentiviral packaging.
The sequence used for CMTM4 knockdown was sh3: 5'-GAAATTGCTGCCGTGATAT-3', and the control sequence was shN: 5'-CCTAAGGTTAAGTCGCCCTCG-3 (13). The target gene was digested with MluI and XhoI and then cloned into the pGIPZ lentiviral vector and transformed into DH5α. Recombinant plasmids (GIPZ-shN, and GIPZ-sh3) were identified by DNA sequencing.
The recombinant plasmids, pSPAX2 and pMD2G were co-transfected into the HEK 293T cells for lentiviral packaging using LipofiterTM (Hanbio). The cell culture supernatants containing lentivirus were collected 48 h after transfection. The cell supernatants were mixed with 2 µg/mL of polybrane (Millipore, Billerica, MA, USA) for the infection of the AGS and SGC7901 cells.
Real-time reverse transcription polymerase chain reaction (RT-qPCR)
The total RNA of the cells was abstracted using the TRIzol reagent (Invitrogen, Carlsbad, CA, USA). Reverse transcription was performed using the complementary DNA (cDNA) Synthesis Kit (Tiangen Biotech, Beijing, China). The primers used for RT-qPCR were: CMTM4-F, 5'-GCACCAATGACTACATCCGAGC-3'; CMTM4-R, 5'-GCCAGTGATTCAAACTCAGCAGG-3'; ACTB-F, 5'-CACCATTGGCAATGAGCGGTTC-3'; and ACTB-R, 5'-AGGTCTTTGCGGATGTCCACGT-3'. The expression of CMTM4 messenger RNA (mRNA) was expressed as the fold change relative to the gene ACTB.
Cell proliferation assay
The cells were inoculated at 4,000 cells/well in 96-well plates. After culturing for 24, 48, and 72 hours, the Cell Counting Kit 8 (CCK-8) reagent (Dojindo, Kumamoto, Japan) was used to detect the growth of the cells. The optical density (OD) value was measured at 460 nm. The experiment was repeated three times.
Colony formation assay
The cells were inoculated at 500 cells/well in 6-well plates. After culturing for 2 weeks, the cells were fixed with 4% paraformaldehyde and stained with crystalline violet. Photographs were taken, and a statistical analysis of the number of cell clones was performed. The experiment was repeated three times.
Cell apoptosis assay
The cells were inoculated at 2×105 cells/well in 12-well plates. After culturing for 72 hours, the cells were collected and resuspended in binding buffer for staining with the 688-conjugated AnnexinV and propidium iodide (PI) reagents (Dojindo). Cell apoptosis was analyzed by flow cytometry using a FACSCanto instrument (Beckman, Brea, CA, USA). The experiment was repeated three times.
Cell cycle analysis
The cells were inoculated at 2×105 cells/well in 12-well plates and cultured for 12 hours. After starving for 24 hours, RPMI 1640 containing 10% FBS was added and incubation was continued for 48 hours. The cells were fixed with cold 70% ethanol and stained with the PI (Dojindo). Cell cycle was analyzed by flow cytometry using a FACSCanto instrument (Beckman). The experiment was repeated three times.
Wound healing assay
The cells were inoculated at 4×105 cells/well in 12-well plates and incubated for 12 hours. A straight line was scratched with a pipette tip in the middle of the wells. RPMI 1640 without FBS was added after washing three times with PBS, and micrographs were taken. Micrographs were taken again 48 hours later. The area of the scratch at different times was measured using Image J 1.53. The wound healing rate was calculated using the scratch area at 0 hour as a reference. The experiment was repeated three times.
Cell invasion assay
Matrigel (100 µL) was added to the transwell chambers (Corning, Steuben County, USA) and incubated at 37 °C for 30 min for clotting. The cells were inoculated at 1×105 cells/well in the chamber in serum-free medium, and medium containing 10% FBS was added to the well. After culturing for 48 hours, cells on the lower surface of the chamber were fixed and then stained with crystal violet. Three fields were randomly selected for cell counting (magnification, ×100). The experiment was repeated three times.
Proteomic analysis
The AGS-NC and AGS-CMTM4OE cells were cultured at 4×106 per 10-cm dish, and the cells were collected after 24 hours. DB proteolytic solution [8 M urea, 100 mM triethylammonium bicarbonate (TEAB), pH =8.5] was added to the protein, and then centrifuged at 4 °C and 12,000 ×g for 15 min after mixing well and sonicating in an ice water bath for 5 min. Add dithiothreitol (DTT) (1 M) to the supernatant and reacted at 56 °C for 1 min, then place the supernatant on ice for 2 min. The IAM was added to the supernatant and reacted at room temperature, avoiding light, for 1 hour.
The protein quantification was performed using the Bradford kit, followed by 12% SDS-PAGE and Komas Brilliant Blue staining. DB proteolytic solution, trypsin, and 100 mm TEAB buffer were added to the protein, and then digested with trypsin and Calcium chloride. Formic acid was added to make pH <3. After centrifugation the supernatant was slowly desalted through a C18SPE column and dried under vacuum. The peptide was re-solubilized with 0.1 M TEAB buffer and labeled with 41 µL of acetonitrile solubilized TMT. The labeled peptides were desalted and lyophilized. Fractions were separated using an L-3000 HPLC system. The separated peptides were analyzed by the Q ExactiveTM HF-X mass spectrometer and mass spectrometry (MS) detection. The resulting spectra were searched by Proteome Discoverer (Thermo, HFX and 480). The expression of the proteins was statistically analyzed, and proteins with a P value <0.05 and |log2 fold change| value >0.25 were defined as differentially expressed proteins. The functions and pathways of the proteins were analyzed using the Gene Ontology (GO) and Kyoto Encyclopedia of Genes and Genomes (KEGG) databases (29,30).
Western blot analysis
The cells were lysed with the lysis buffer (Solarbio, Beijing, China) containing 1% protease inhibitor and 2% phosphatase inhibitor (Solarbio). After determining the concentration, the protein was subjected to 10% sodium dodecyl-sulfate polyacrylamide gel electrophoresis (SDS-PAGE), and then transferred to polyvinylidene fluoride (PVDF) membranes. The PVDF membranes were blocked with 5% skimmed milk and then incubated with the primary antibody overnight. After incubating the membranes with the secondary antibody, protein expression was detected using the chemiluminescence reagent (Yamei, Shanghai, China). Protein expression was measured using the target protein/β-actin ratio. Primary antibodies against STAT1 (YP0249) and p-STAT1 (YM4105) were purchased from Immunoway (Plano, TX, USA). Primary antibody against β-actin (K101527P) was purchased from Solarbio. Primary antibodies against CMTM4 (17433S) were purchased from Sigma-Aldrich (St. Louis, MO, USA). Secondary horseradish peroxidase-conjugated antibodies against-mouse Immunoglobulin G (IgG) (7074S) or rabbit IgG (7076S) were purchased from Cell Signaling Technology (Danvers, MA, USA). The experiment was repeated three times.
Statistical analysis
IBM SPSS Statistics 22.0 (Chicago, IL, USA) was used for the statistical analysis. The Student’s t-test was used to compare the quantitative data between two groups, with a |log2 (fold change)| >0.25 and P value <0.05 indicating a statistically significant difference. All experiments had at least three technical or biological replicates. Three independent replicate experiments were performed.
Results
CMTM4 is downregulated in GC and is associated with a good prognosis
A study based on several GC datasets (Allander Gastric, Wang Gastric, Derrico Gastric, and Cho Gastric) have reported that CMTM4 expression is significantly downregulated in GC tissues (26). We further analyzed the CMTM4 expression in the gastric mucosa of patients with GC or gastritis using immunohistochemistry. The results showed that the expression of CMTM4 was significantly lower in the GC tissues than the gastritis tissues (P<0.01) (Figure 1A,1B). Survival curves were plotted using the Kaplan-Meier Plotter, and the results showed that the median survival time of the GC patients with high CMTM4 expression (23.6 months) was significantly longer than that of the GC patients with low CMTM4 expression (12.67 months, P<0.01) (Figure 1C), suggesting that high CMTM4 expression is a protective factor for GC.
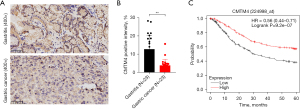
CMTM4 inhibits the proliferation of GC cells
To clarify the function of CMTM4 in the GC cells, we constructed CMTM4 overexpression (AGS-CMTM4OE) and knockdown AGS cells (AGS-sh3), and CMTM4 overexpression SGC7901 cells (SGC7901-CMTM4OE). The expression of CMTM4 was verified using RT-qPCR (Figure S1).
One of the important characteristics of tumor cells is their abnormal proliferative capacity. We analyzed the effect of CMTM4 on the proliferation of GC cells using a CCK-8 analysis. The results showed that CMTM4 overexpression significantly inhibited the proliferation of the AGS and SGC7901 cells (72 h, P<0.01), whereas CMTM4 knockdown promoted the proliferation of AGS cells (48 and 72 h, P<0.01) (Figure 2A-2C). The inhibitory effect of CMTM4 on the growth of the AGS and SGC7901 cells was further confirmed using a plate cloning assay (Figure 2D-2I).
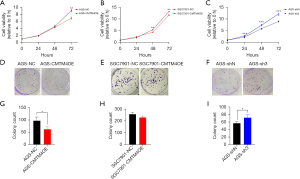
CMTM4 promotes apoptosis and results in the G1/S arrest of GC cells
The effects of CMTM4 on the apoptosis and cell cycle of the GC cells were studied by flow cytometry. The proportion of apoptotic cells in the CMTM4 overexpression AGS and SGC7901 cells was significantly higher than that of the control. The apoptosis of the CMTM4 knockdown AGS cells was lower than that of the control group (P<0.05) (Figure 3A-3F), suggesting that CMTM4 promotes apoptosis in GC cells. Further, we examined the effect of CMTM4 on the cell cycle and found that the proportion of cells in the G0/G1 phase in the CMTM4 overexpression AGS and SGC7901 cells was significantly increased, whereas that of the cells in the S phase was decreased (P<0.05) (Figure 3G-3L), suggesting that CMTM4 may also inhibit cell proliferation by inducing G1/S phase arrest.
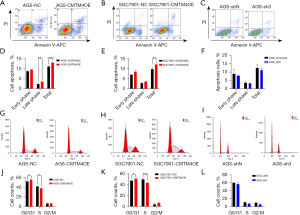
CMTM4 inhibits the migration of GC cells
The effect of CMTM4 on the migration of GC cells was determined by wound healing assay. The migration rates of the AGS-CMTM4OE and SGC7901-CMTM4OE cells were significantly lower than those of the control cells at 48 h (P<0.05), while the migration rate of the AGS-sh3 cells was significantly higher than that of the control group (P<0.05) (Figure 4A-4F), suggesting that CMTM4 inhibited the migration of GC cells.
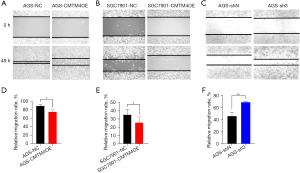
CMTM4 inhibits the invasion of GC cells
Transwell assay was used to examine the effect of CMTM4 on the invasion of the AGS and SGC7901 cells. The number of membrane-penetrating cells was significantly lower in the CMTM4 overexpression AGS and SGC7901 cells (P<0.01), while the number of membrane-penetrating AGS cells with CMTM4 knockdown was significantly higher compared with the control group (P<0.05) (Figure 5A-5F). This suggests that CMTM4 decreased the invasive ability of GC cells.
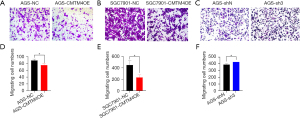
CMTM4 upregulates STAT1 expression
To explore the possible mechanisms of CMTM4 function in GC cells, a proteomic analysis was performed to identify the differentially expressed proteins between the AGS-NC and AGS-CMTM4OE groups. Based on a comparison of the AGS-CMTM4OE and AGS-NC groups, a total of 33 differentially expressed proteins were identified, of which 16 were upregulated and 17 were downregulated, (Figure 6A,6B, Table S2). We found that STAT1 was significantly upregulated in the AGS-CMTM4OE group, and the upregulated proteins were enriched in STAT1 related signaling pathways, such as GO:0035455, GO:0060333, GO:0035456, and GO:0034341 (Figure 6C, Table 1). Further, the western blot experiments verified that both the total and phosphorylated STAT1 were upregulated in the CMTM4 overexpression AGS cells (Figure 6D). These results suggested that CMTM4 exerts an anti-tumor function by affecting the STAT1 signaling pathway.
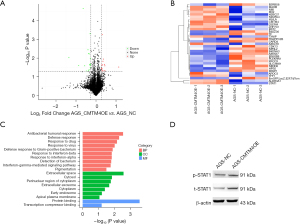
Table 1
Category | Term | Pathways | Count | Proteins | Fold enrichment | P value |
---|---|---|---|---|---|---|
GO-BP | GO:0035455 | Response to interferon-alpha | 2 | BST2, MX2 | 130.189 | 0.02 |
GO-BP | GO:0035456 | Response to interferon-beta | 2 | STAT1, BST2 | 130.189 | 0.02 |
GO-BP | GO:0016045 | Detection of bacterium | 2 | HLA-A, CARD4 | 119.340 | 0.02 |
GO-BP | GO:0060333 | Interferon-gamma-mediated signaling pathway | 2 | STAT1, NMI | 110.160 | 0.02 |
GO-BP | GO:0043473 | Pigmentation | 2 | B4DZ36, HPS5 | 59.670 | 0.03 |
GO-BP | GO:0034341 | Response to interferon-gamma | 2 | STAT1, BST2 | 51.146 | 0.04 |
GO-BP | GO:0019731 | Antibacterial humoral response | 3 | HLA-A, H2BC21, TF | 36.409 | 0.003 |
GO-BP | GO:0006952 | Defense response | 3 | STAT1, MX2, CARD4 | 27.191 | 0.005 |
GO-BP | GO:0009615 | Response to virus | 3 | BST2, MX2, NMI | 19.352 | 0.01 |
GO-BP | GO:0050830 | Defense response to Gram-positive bacterium | 3 | HLA-A, H2BC21, CARD4 | 16.652 | 0.01 |
GO-BP | GO:0042493 | Response to drug | 4 | STAT1, ALDH3A1, TXNIP, MAOB | 9.775 | 0.007 |
GO-BP | GO:0051607 | Defense response to virus | 3 | STAT1, BST2, MX2 | 9.180 | 0.04 |
GO-BP | GO:0009410 | Response to xenobiotic stimulus | 3 | STAT1, TXNIP, MAOB | 8.876 | 0.04 |
GO-CC | GO:0048471 | Perinuclear region of cytoplasm | 5 | STAT1, OLFM4, XRCC3, TRAPPC2B, TF | 4.798 | 0.02 |
GO-BP | GO:0045087 | Innate immune response | 4 | HLA-A, BST2, MX2, CARD4 | 4.734 | 0.046 |
GO-CC | GO:0005615 | Extracellular space | 9 | OLFM4, ALDH3A1, H2BC21, B4DZ36, TF, APOC3, NMI, TFF1, CDK13 | 3.412 | 0.003 |
GO-CC | GO:0070062 | Extracellular exosome | 8 | NEDD8, OLFM4, HLA-A, H2BC21, B4DZ36, TF, BST2, APOC3 | 2.650 | 0.02 |
GO-CC | GO:0005829 | Cytosol | 14 | NEDD8, OLFM4, A8K5X7, ALDH3A1, XRCC3, H2BC21, BST2, CARD4, STAT1, MX2, TXNIP, NMI, CDK13, HPS5 | 1.864 | 0.01 |
GO-CC | GO:0005737 | Cytoplasm | 13 | ZNF703, NEDD8, ALDH3A1, XRCC3, BST2, CARD4, STAT1, TRAPPC2B, B4DZ36, MX2, TXNIP, NMI, HPS5 | 1.708 | 0.04 |
GO-MF | GO:0005515 | Protein binding | 27 | ZNF703, OLFM4, ALDH3A1, BST2, NDUFA4, CARD4, STAT1, SLC39A9, HLA-A, NPRL2, TRAPPC2B, TF, APOC3, MX2, TXNIP, NMI, CDK13, NEDD8, A8K5X7, XRCC3, H2BC21, PLEKHN1, CMTM4, RPAP1, MAOB, TFF1, HPS5 | 1.445 | <0.01 |
KEGG | hsa00360 | Phenylalanine metabolism | 2 | ALDH3A1, MAOB | 64.10 | 0.03 |
KEGG | hsa00340 | Histidine metabolism | 2 | ALDH3A1, MAOB | 46.619 | 0.04 |
KEGG | hsa04621 | Nod-like receptor signaling pathway | 3 | STAT1, TXNIP, CARD4 | 8.361 | 0.04 |
GO, Gene Ontology; KEGG, Kyoto Encyclopedia of Genes and Genomes; BP, biological process; CC, cellular component; MF, molecular function.
The inhibitory effects of CMTM4 on GC cell proliferation, migration, and invasion are STAT1 dependent
To verify whether STAT1 was involved in the effects of CMTM4 on the GC cells, the STAT1 inhibitor fludarabine was used to determine whether inhibiting STAT1 could rescue CMTM4-induced changes in the GC cells. The results showed no obvious difference in proliferation between the AGS-CMTM4OE and AGS-NC cells after fludarabine treatment (Figure 7A,7B). Moreover, the inhibition of GC cell migration (Figure 7C-7F) and invasion (Figure 7G-7J) by CMTM4 overexpression disappeared, which suggests that STAT1 is the key molecule involved in the inhibitory effect of CMTM4 on GC cells.
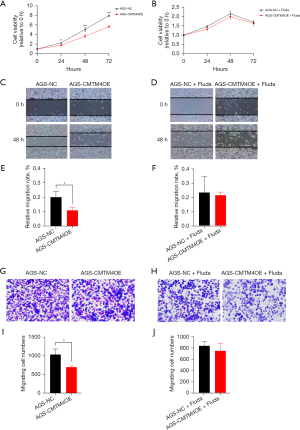
Discussion
Previous results have reported that CMTM4 mRNA expression is decreased in GC tissues and high CMTM4 gene expression is a protective factor for the prognosis of GC patients (26,27). In this study, we explored the function and mechanism of CMTM4 in GC, and found that: (I) CMTM4 expression was reduced in GC tissues, and high CMTM4 expression was significantly associated with a good prognosis in GC; (II) CMTM4 overexpression inhibited the tumorigenesis of GC cells, caused cell cycle G1/S phase arrest, and promoted the apoptosis, while CMTM4 knockdown produced the opposite effects; (III) CMTM4 overexpression upregulated STAT1 expression and activated the related pathways; and (IV) the effect of CMTM4 on the GC cells was restored by the STAT1-specific inhibitor fludarabine. These results suggested that CMTM4 could play a tumor suppressor role in GC.
CMTM4 is one of the most conserved proteins in the CMTM family, with a quadratic transmembrane structure containing the MARVEL domain (8). CMTM4 plays an important role in regulating the transport and stability of membrane molecules and the occurrence and development of tumors. Knizkova et al. (22) reported that CMTM4 assembles with IL-17RC and IL-17RA to form the IL17 receptor complex and is necessary for IL17A signaling pathway. CMTM4 also promotes EGFR degradation by promoting Rab5 activity and inhibits the EGF signaling pathway (13). Li et al. (23) found that CMTM4 significantly inhibited the growth of renal cells, which may be caused by CMTM4 up-regulating p21 protein and affecting the cell cycle. Xue et al. (13) found that CMTM4 inhibits the phosphorylation of AKT, ERK1/2 and STAT3, suppressing the proliferation, invasion, migration and metastasis of tumor cells, which is consistent with our results.
The regulation of the cell cycle plays a key role in cell proliferation. It has been reported that CMTM4 induces G2/M cell cycle arrest and upregulates p21, thus inhibiting cell proliferation (23,31). CMTM4 overexpression also inhibits HeLa cell growth by inducing G2/M phase accumulation (32). Cell cycle regulation involves multiple factors, including negative regulatory factors, such as cyclin-dependent kinase (CDK) inhibitors, positive regulatory factors, such as cyclins, and CDKs (33,34). In our study, the CMTM4 overexpressed cells underwent G1/S phase arrest during the cell cycle, and no significant G2/M phase arrest was observed. The G1-S phase transition is a major checkpoint in the cell cycle. The G1 phase and G1/S transition are regulated by activators (cyclin D1-D3, cyclin E1, and CDK4) and inhibitors (p21, p27, and p16) (35,36). However, the mechanism by which CMTM4 induces G1/S phase arrest requires further investigation.
Protein expression profiling was conducted to explore the potential signaling pathways involved in the inhibitory effect of CMTM4 on GC. The differentially expressed genes were significantly enriched in the interferon-mediated STAT1 signaling pathway. Interferon gamma (IFN-γ) primarily activates Janus kinase (JAK) including JAK1 and JAK2, inducing STAT1 to form homodimers (37). Studies had shown the critical anti-tumor effects of IFN-γ. For example, IFN-γ has been shown to induce immunosurveillance and adaptive immunity against tumor cells, and to inhibit cell proliferation and promote apoptosis (38,39). IFN-γ has also been shown to suppress cell proliferation through the activation of STAT1 and the upregulation of CDK inhibitor 1 (40). Further research has shown that IFN-γ upregulates the expression of caspase-8 in a manner dependent on the STAT1/IRF1 pathway, thereby promoting tumor cell apoptosis (41). STAT1 acts as an inhibitor of tumor angiogenesis and metastasis. Previous studies have shown that STAT1 promotes apoptosis in tumors by inducing the expression of surface cell death receptors and their ligands (42,43). Varikuti et al. (44) reported that STAT1 inhibits tumor growth and metastasis in breast cancer. In this study, CMTM4 was shown to inhibit the proliferation, invasion, and metastasis of GC cells in a STAT1-dependent manner.
In addition, we found that the expression of CMTM4 in non-cancerous tissues was significantly higher than that in GC tissues. The expression of CMTM4 is significantly associated with good prognosis of GC patients. Although currently there is a lack of trials investigating survival according to CMTM4 status. It suggests that CMTM4 has potential value as a prognosis marker and potential therapeutic target for GC therapy. There are some limitations in this study. The mechanism by which CMTM4 regulates STAT1 and STAT1 targeted molecules was not clear. IFN-γ promotes the phosphorylation of STAT1 but not the total STAT1 protein. Therefore, CMTM4 may promote total STAT1 expression through pathways other than IFN-γ. It would be valuable to further validate the upstream molecules of CMTM4.
Conclusions
Our study demonstrated that CMTM4 expression is decreased in GC, and a high expression of CMTM4 is associated with a good prognosis in GC patients. CMTM4 inhibits the proliferation and metastasis of GC cells in a STAT1-dependent manner. These results highlight the tumor-suppressor function of CMTM4 in GC and suggest a new target for GC therapy.
Acknowledgments
We would like to thank Professor Wenling Han (Department of Immunology, School of Basic Medical Sciences, Peking University Health Science Center) and Professor Jun Wang (CAS Key Laboratory of Pathogenic Microbiology and Immunology, Institute of Microbiology, Chinese Academy of Sciences) for their guidance.
Funding: This work was supported by grants from
Footnote
Reporting Checklist: The authors have completed the MDAR reporting checklist. Available at https://jgo.amegroups.com/article/view/10.21037/jgo-24-466/rc
Data Sharing Statement: Available at https://jgo.amegroups.com/article/view/10.21037/jgo-24-466/dss
Peer Review File: Available at https://jgo.amegroups.com/article/view/10.21037/jgo-24-466/prf
Conflicts of Interest: All authors have completed the ICMJE uniform disclosure form (available at https://jgo.amegroups.com/article/view/10.21037/jgo-24-466/coif). The authors have no conflicts of interest to declare.
Ethical Statement: The authors are accountable for all aspects of the work in ensuring that questions related to the accuracy or integrity of any part of the work are appropriately investigated and resolved. The study was conducted in accordance with the Declaration of Helsinki (as revised in 2013). This study was approved by the Ethics Committee of Peking University Third Hospital (No. S2022179) and informed consent was taken from all the patients.
Open Access Statement: This is an Open Access article distributed in accordance with the Creative Commons Attribution-NonCommercial-NoDerivs 4.0 International License (CC BY-NC-ND 4.0), which permits the non-commercial replication and distribution of the article with the strict proviso that no changes or edits are made and the original work is properly cited (including links to both the formal publication through the relevant DOI and the license). See: https://creativecommons.org/licenses/by-nc-nd/4.0/.
References
- Sung H, Ferlay J, Siegel RL, et al. Global Cancer Statistics 2020: GLOBOCAN Estimates of Incidence and Mortality Worldwide for 36 Cancers in 185 Countries. CA Cancer J Clin 2021;71:209-49. [Crossref] [PubMed]
- Yan C, Shan F, Li Z. Analysis of Global Burden of Gastric Cancer in 2020: Focusing on the Statistics in China. China Cancer 2023;32:161-70.
- Cao W, Chen HD, Yu YW, et al. Changing profiles of cancer burden worldwide and in China: a secondary analysis of the global cancer statistics 2020. Chin Med J (Engl) 2021;134:783-91. [Crossref] [PubMed]
- Allemani C, Matsuda T, Di Carlo V, et al. Global surveillance of trends in cancer survival 2000-14 (CONCORD-3): analysis of individual records for 37 513 025 patients diagnosed with one of 18 cancers from 322 population-based registries in 71 countries. Lancet 2018;391:1023-75. [Crossref] [PubMed]
- Akhavan-Niaki H, Samadani AA. Molecular insight in gastric cancer induction: an overview of cancer stemness genes. Cell Biochem Biophys 2014;68:463-73. [Crossref] [PubMed]
- Wu X, Chen L, Cheng J, et al. Effect of Dietary Salt Intake on Risk of Gastric Cancer: A Systematic Review and Meta-Analysis of Case-Control Studies. Nutrients 2022;14:4260. [Crossref] [PubMed]
- Marano L, Polom K, Patriti A, et al. Surgical management of advanced gastric cancer: An evolving issue. Eur J Surg Oncol 2016;42:18-27. [Crossref] [PubMed]
- Han W, Ding P, Xu M, et al. Identification of eight genes encoding chemokine-like factor superfamily members 1-8 (CKLFSF1-8) by in silico cloning and experimental validation. Genomics 2003;81:609-17. [Crossref] [PubMed]
- Wu K, Li X, Gu H, et al. Research Advances in CKLF-like MARVEL Transmembrane Domain-containing Family in Non-small Cell Lung Cancer. Int J Biol Sci 2019;15:2576-83. [Crossref] [PubMed]
- Ge YY, Duan HJ, Deng XL. Possible effects of chemokine-like factor-like MAR-VEL transmembrane domain-containing family on antiphospholipid syndrome. Chin Med J (Engl) 2021;134:1661-8. [Crossref] [PubMed]
- Duan HJ, Li XY, Liu C, et al. Chemokine-like factor-like MARVEL transmembrane domain-containing family in autoimmune diseases. Chin Med J (Engl) 2020;133:951-8. [Crossref] [PubMed]
- Fan D, Fan D, Yuan W. CMTM3 suppresses bone formation and osteogenic differentiation of mesenchymal stem cells through inhibiting Erk1/2 and RUNX2 pathways. Genes Dis 2021;8:882-90. [Crossref] [PubMed]
- Xue H, Li T, Wang P, et al. CMTM4 inhibits cell proliferation and migration via AKT, ERK1/2, and STAT3 pathway in colorectal cancer. Acta Biochim Biophys Sin (Shanghai) 2019;51:915-24. [Crossref] [PubMed]
- Li L, Hu Y, Chen D, et al. CMTM5 inhibits the development of prostate cancer via the EGFR/PI3K/AKT signaling pathway. Mol Med Rep 2022;25:17. [Crossref] [PubMed]
- Yuan W, Liu B, Wang X, et al. CMTM3 decreases EGFR expression and EGF-mediated tumorigenicity by promoting Rab5 activity in gastric cancer. Cancer Lett 2017;386:77-86. [Crossref] [PubMed]
- Zhang J, Ning J, Fu W, et al. CMTM3 protects the gastric epithelial cells from apoptosis and promotes IL-8 by stabilizing NEMO during Helicobacter pylori infection. Gut Pathog 2023;15:6. [Crossref] [PubMed]
- Yu X, Zhai X, Wu J, et al. Evolving perspectives regarding the role of the PD-1/PD-L1 pathway in gastric cancer immunotherapy. Biochim Biophys Acta Mol Basis Dis 2024;1870:166881. [Crossref] [PubMed]
- Mezzadra R, Sun C, Jae LT, et al. Identification of CMTM6 and CMTM4 as PD-L1 protein regulators. Nature 2017;549:106-10. [Crossref] [PubMed]
- Imamovic D, Vranic S. Novel regulators of PD-L1 expression in cancer: CMTM6 and CMTM4-a new avenue to enhance the therapeutic benefits of immune checkpoint inhibitors. Ann Transl Med 2017;5:467. [Crossref] [PubMed]
- Foley JF. CMTM4 makes IL-17 signaling more complex. Sci Signal 2022;15:eadf9180. [Crossref] [PubMed]
- Galdiero MR, Ciaglia E, Dal Col J. CMTM4 as a new partner for IL-17 receptor: Adding a piece in the puzzle of IL17-driven diseases. Allergy 2023;78:3282-4. [Crossref] [PubMed]
- Knizkova D, Pribikova M, Draberova H, et al. CMTM4 is a subunit of the IL-17 receptor and mediates autoimmune pathology. Nat Immunol 2022;23:1644-52. [Crossref] [PubMed]
- Li T, Cheng Y, Wang P, et al. CMTM4 is frequently downregulated and functions as a tumour suppressor in clear cell renal cell carcinoma. J Exp Clin Cancer Res 2015;34:122. [Crossref] [PubMed]
- Bei C, Zhang Y, Wei R, et al. Clinical significance of CMTM4 expression in hepatocellular carcinoma. Onco Targets Ther 2017;10:5439-43. [Crossref] [PubMed]
- Pei Y, Zhang Z, Tan S. Current Opinions on the Relationship Between CMTM Family and Hepatocellular Carcinoma. J Hepatocell Carcinoma 2023;10:1411-22. [Crossref] [PubMed]
- Liang Z, Xie J, Huang L, et al. Comprehensive analysis of the prognostic value of the chemokine-like factor-like MARVEL transmembrane domain-containing family in gastric cancer. J Gastrointest Oncol 2021;12:388-406. [Crossref] [PubMed]
- Zhou L, Lu H, Zeng F, et al. Constructing a new prognostic signature of gastric cancer based on multiple data sets. Bioengineered 2021;12:2820-35. [Crossref] [PubMed]
- Guo Y, Zhang T, Shi Y, et al. Helicobacter pylori inhibits GKN1 expression via the CagA/p-ERK/AUF1 pathway. Helicobacter 2020;25:e12665. [Crossref] [PubMed]
- Buczak K, Kirkpatrick JM, Truckenmueller F, et al. Spatially resolved analysis of FFPE tissue proteomes by quantitative mass spectrometry. Nat Protoc 2020;15:2956-79. [Crossref] [PubMed]
- Zhang H, Liu T, Zhang Z, et al. Integrated Proteogenomic Characterization of Human High-Grade Serous Ovarian Cancer. Cell 2016;166:755-65. [PubMed]
- Li M, Guo H, Wang Q, et al. Pancreatic stellate cells derived exosomal miR-5703 promotes pancreatic cancer by downregulating CMTM4 and activating PI3K/Akt pathway. Cancer Lett 2020;490:20-30. [Crossref] [PubMed]
- Plate M, Li T, Wang Y, et al. Identification and characterization of CMTM4, a novel gene with inhibitory effects on HeLa cell growth through Inducing G2/M phase accumulation. Mol Cells 2010;29:355-61. [Crossref] [PubMed]
- Graña X, Reddy EP. Cell cycle control in mammalian cells: role of cyclins, cyclin dependent kinases (CDKs), growth suppressor genes and cyclin-dependent kinase inhibitors (CKIs). Oncogene 1995;11:211-9. [PubMed]
- Bertoli C, Skotheim JM, de Bruin RA. Control of cell cycle transcription during G1 and S phases. Nat Rev Mol Cell Biol 2013;14:518-28. [Crossref] [PubMed]
- Yang Q, Wang B, Gao W, et al. SIRT1 is downregulated in gastric cancer and leads to G1-phase arrest via NF-κB/Cyclin D1 signaling. Mol Cancer Res 2013;11:1497-507. [Crossref] [PubMed]
- Zhang CY, Bao W, Wang LH. Downregulation of p16(ink4a) inhibits cell proliferation and induces G1 cell cycle arrest in cervical cancer cells. Int J Mol Med 2014;33:1577-85. [Crossref] [PubMed]
- Michalska A, Blaszczyk K, Wesoly J, et al. A Positive Feedback Amplifier Circuit That Regulates Interferon (IFN)-Stimulated Gene Expression and Controls Type I and Type II IFN Responses. Front Immunol 2018;9:1135. [Crossref] [PubMed]
- Kursunel MA, Esendagli G. The untold story of IFN-γ in cancer biology. Cytokine Growth Factor Rev 2016;31:73-81. [Crossref] [PubMed]
- Sun R, Luo J, Li D, et al. Neutrophils with protumor potential could efficiently suppress tumor growth after cytokine priming and in presence of normal NK cells. Oncotarget 2014;5:12621-34. [Crossref] [PubMed]
- Chin YE, Kitagawa M, Su WC, et al. Cell growth arrest and induction of cyclin-dependent kinase inhibitor p21 WAF1/CIP1 mediated by STAT1. Science 1996;272:719-22. [Crossref] [PubMed]
- Rodriguez A, Jung EJ, Yin Q, et al. Role of c-myc regulation in Zta-mediated induction of the cyclin-dependent kinase inhibitors p21 and p27 and cell growth arrest. Virology 2001;284:159-69. [Crossref] [PubMed]
- Verhoeven Y, Tilborghs S, Jacobs J, et al. The potential and controversy of targeting STAT family members in cancer. Semin Cancer Biol 2020;60:41-56. [Crossref] [PubMed]
- Avalle L, Pensa S, Regis G, et al. STAT1 and STAT3 in tumorigenesis: A matter of balance. JAKSTAT 2012;1:65-72. [Crossref] [PubMed]
- Varikuti S, Oghumu S, Elbaz M, et al. STAT1 gene deficient mice develop accelerated breast cancer growth and metastasis which is reduced by IL-17 blockade. Oncoimmunology 2017;6:e1361088. [Crossref] [PubMed]